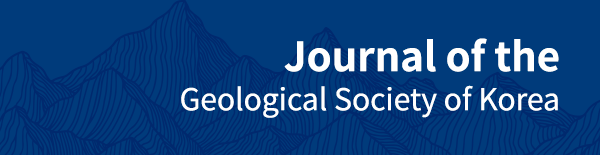
시추코어에서 소단층을 이용한 고응력장 복원: 한반도 남동부 제4기 단층운동 해석에의 적용
Copyright © 2017 The Geological Society of Korea
This is an Open Access article distributed under the terms of the Creative Commons Attribution Non-Commercial License (http://creativecommons.org/licenses/by-nc/3.0/ which permits unrestricted non-commercial use, distribution, and reproduction in any medium, provided the original work is properly cited.
초록
포항 장기4호공의 시추코어로부터 수집한 소단층의 단층이동 자료를 이용하여 고응력장 복원을 시도하였다. 소단층 자료는 공내영상이미지에서 확인되는 층리면의 자세를 이용하여 정방위 복원하였다. 다중역산법을 이용한 고응력장 복원을 통해 남-북 방향과 북서-남동 방향의 압축력을 구하였다. 변형띠와 단층끌림에 대한 기하학적, 운동학적 분석 및 소단층 자료에 대한 오차각 계산결과는 북서-남동 방향의 압축력이 남-북 방향의 압축력 이후에 작용한 것임을 지시한다. 구해진 두 응력조건은 비록 현재 알려진 한반도의 대표적인 주응력방향과는 차이가 있지만 기록된 지층의 연대와 이전의 연구에 기초하면 이들이 한반도 남동부 제4기 단층운동과 관련된 것으로 판단된다. 이러한 연구결과는 본 연구지역과 인접한 지역에서의 신기지체구조운동과 이산화탄소의 지중저장 이후 누출특성을 예측하는 모델수립에 활용될 수 있을 것이다.
Abstract
Paleostress reconstruction for striated faults was conducted to infer stress field evolution in a borehole of JG-4 within the Janggi basin, SE Korea. The fault-slip data from the un-oriented cores were collected with an assumption on the bedding in borehole imagery, which can be used as a reference fabric to reorient the faults into their original positions. The oriented fault data from the core were processed based on multiple inversion method, where two stress states were identified: N-S compression and NW-SE compression. The geometric and kinematic analysis of deformation bands, fault drags from the cores, and the misfit angles on the striated faults suggest that NW-SE compression is younger than N-S compression. Although the inferred stress states are slightly different from the known Quaternary compressive stress direction, they may indicate other faulting events during the Quaternary in SE Korea, based on the age of the recorded rocks and previous studies. This work could help for the establishment of neotectonic framework and the leakage characteristics of CO2 storage in this area.
Keywords:
fault-slip analysis, paleostress, multiple inversion method, the Quaternary fault, drill core키워드:
단층구조 분석, 고응력장, 다중역산법, 제4기 단층, 시추코어1. 서 론
제4기 단층들이 한반도 남동부 지역의 양산-울산 단층계와 이들 단층들로 경계된 포항-울산지괴에서 다수 보고되고 있다(그림 1). 이 지역에서 제4기 단층의 존재는 고지진학적 연구(Okada et al., 1994), 단층암(Lee and Schwarcz, 2001) 혹은 단층에 의해 절단된 퇴적층(Ree et al., 2003; Choi et al., 2009)에 대한 정밀한 연대측정을 통해 밝혀진 바 있다. 2016년 9월 12일, 규모 5.8의 경주지진은 현생 지구조응력조건 하에서 이미 보고된 제4기 단층들의 활동 가능성에 대한 관심을 불러일으키는 계기가 되었다.

Geological map and location map of Quaternary faults in SE Korea. (a, b) Location and regional geological map of the Gyeongsang basin (modified form Lee, 2000). (c) Distribution of Quaternary fault sites and the measured last fault movement age in the southeastern part of the Korean peninsula (modified from Yang, 2006).
한반도 남동부 지역에서 보고된 제4기 단층들의 운동특성을 규명하기 위해 소단층 자료를 이용한 고응력장 복원 연구들이 일부 진행된 바 있으나, 제4기 단층운동과 관련된 지구조응력조건에 대한 논쟁은 현재까지 이어지고 있다(Choi et al., 2002, 2007; Park et al., 2006; Kim et al., 2016). 이번 9.12 경주지진을 포함한 계기지진 자료의 발진기구로부터 구한 현생지구조응력조건은 한반도에서 동북동-서남서 내지동-서 방향의 최대수평주응력이 우세하게 작용하고 있음을 보여준다(Jun and Jeon, 2010). 제4기 단층운동은 이러한 현생 응력조건의 압축응력방향과 관계된 일련의 사건으로 생각되었다(Park et al., 2006; Kim et al., 2016).
한편, Choi et al. (2002)와 Choi et al. (2007)은 한반도 남동부에서 수행한 단층구조분석을 통해 제4기 단층운동과 관계된 적어도 세 개 이상의 지구조응력조건 존재 가능성을 제안하였으며, 그 압축력의 축들은 동북동-서남서, 남-북 그리고 서북서-동남동 방향으로 제시되었다. Choi et al. (2007)은 제4기 단층이동벡터와 그 연대측정 결과를 바탕으로 이러한 복수의 지구조응력조건들이 상호 공존한다고 제안하였다.
최근 Gwon and Kim (2016)은 한반도 남동부 불국사화강암류를 기반암으로 한 트렌치조사에서의 정밀 단열분석을 통해, 북동-남서 그리고 북서-남동방향의 순서로 작용한 최대압축주응력이 제4기 단층운동을 발생시켰을 가능성을 제시하였다. 한반도 남동부 제4기 단층운동과 관련하여 복수의 지구조응력조건들이 존재한다면, 포항-울산지괴에 다수 분포하고 있는 전기 마이오세 분지들에서도 이러한 단층운동과 관계된 지각변형의 증거가 존재할 가능성이 있다. 그러나 이들 분지에 대한 제4기 고응력장복원 연구는 거의 수행된 바가 없다. 이는 복수의 지구조응력이 실제로 존재하지 않았거나 혹은 이 지역에서 고응력 복원에 필요한 소단층 자료들을 충분히 수집하지 못한 결과일 수 있다.
최근 한반도 남동부 전기 마이오세 분지들에서는 지열발전 및 이산화탄소 지중저장을 위한 부지특성화사업이 활발히 진행되고 있으며, 그 일환으로 전기마이오세 암석들의 시추코어들이 상당수 회수되고 있다. 시추코어 내에 발달하는 소단층 자료를 이용한 고응력장 복원기법은 해외에서는 이미 노두발달이 빈약한 지역에서 지질구조해석 및 단층운동특성 규명을 위해 중요하게 활용하고 있으나(Lallemant et al., 1993; Dezayes et al., 1995; Martin and Bergerat, 1996; Rocher et al., 2000; Yamada and Yamaji, 2002), 국내에서는 이러한 시도가 아직 수행된 바가 없다.
한편, 시추코어로부터 수집된 소단층 자료들 간의 상호절단관계에 대한 불확실성은 이들 단층자료를 이용한 고응력 복원에 상당한 장애가 되고 있다. 기존 연구사례들은 공액상 단층조에 기초하여 (Lallemant et al., 1993; Dezayes et al., 1995; Rocher et al., 2000) 혹은 각각의 암상에 발달하는 우세한 단층조의 발달특성 분석(Martin and Bergerat, 1996)을 통해 고응력 복원을 시도하였다. 그러나 암석에 작용한 변형(strain)비에 따라 하나의 응력조건에서도 다양한 방향성의 공액상 단층조들이 발달할 수 있으며, 이로 인해 실제보다 많은 응력조건이 구해질 수도 있다(Reches, 1978). 이러한 불확실성을 극복하기 위해 Yamaji (2000)의 다중역산법(Multiple Inversion Method)이 이용되기도 했다(Yamada and Yamaji, 2002). 다중역산법은 소단층 자료들 간의 상호절단 관계에 대한 자료수집이 불가능한 경우에도 이를 이용하여 고응력 복원을 할 수 있는 방법이다(Yamaji, 2000; Yamaji et al., 2011).
이 연구는 제4기 단층운동특성 규명을 위해 전기마이오세 장기분지 장기4호공 시추코어의 일부 구간 내에 발달하는 소단층 자료를 이용하여 다중역산법으로 고응력장 복원을 시도하였다. 이를 통해 구한 응력조건들의 선후관계는 시추코어 내에서 관찰되는 구조요소들 즉, 단층끌림과 전단띠, 그리고 소단층간의 기하학적·운동학적 분석을 통해 구하였다. 또한 그 결과를 포항-울산지괴에서 수행된 기존 연구결과들과 비교하였으며, 이를 통해 제4기 단층운동과 연관된 지구조응력조건을 제시하고자 하였다. 이러한 연구결과는 현생 지구조응력조건 하에서 제4기 단층들의 재활 가능성을 평가하는데 매우 중요하게 활용될 수 있을 것이며, 이를 통해 이산화탄소 지중저장에서의 누출특성을 이해하는데 도움을 줄 수 있을 것으로 판단된다.
2. 지질개요
연구지역은 경상분지 남동부에 위치한 전기 마이오세 퇴적분지 중 하나인 장기분지에 위치한다(그림 1, 2). 이들 퇴적분지는 포항-울산지괴에 다수 분포하고 있으며, 이들 분지의 확장 및 역전은 동해의 확장 및 폐쇄와 밀접한 관련이 있다(Chough and Barg, 1987; Yoon and Chough, 1995; Kim et al., 2008). 이들 분지의 충전물들은 북서-남동 방향의 인장응력하에서 퇴적동시성 정단층작용에 수반된 화산암류와 육성기원 퇴적물들로 주로 구성된다(Kim et al., 2015; Son et al., 2015).
연구지역 내 지질은 백악기 퇴적암과 에오세 화산암류를 기반암으로 하여 전기 마이오세 퇴적층들이 북서-남동 및 북북서-남남동 방향으로 발달하는 단층을 경계로 소분지 형태로 발달한다(그림 2). 연구지역 내 퇴적층들은 데사이트질과 현무암질의 이원성 화산물질을 건층으로 하부로부터 범곡리층군의 장기역암과 성동리층 그리고 장기층군에 속하는 뇌성산현무질암으로 세분된다(Kim et al., 2011). 데사이트질 화산암류를 포함하는 장기역암과 성동리층은 주로 북서 방향으로 경사하며, 지층의 경사는 약 25°도 내외이다. 데사이트질 화산암류 지층의 자세는 현무암질암체의 주변에서 교란되어 있으며(Kim et al., 2015), 이 현무암질 화산암류의 K-Ar 연대는 약 22~17 Ma로 측정되었다(Lee et al., 1992).
연구지역의 중앙에 위치한 장기4호공에서 시추코어는 장기역암의 하부층준에 해당하는 지표로부터 심도 약 1,100 m까지 회수되었다. 이번 연구에서는 성동리층의 데사이트질 암석이 분포하는 구간에서 획득된 공내영상이미지(심도 583.5~607 m)와 이로부터 상·하 20 m 내의 7개 구간(심도 562~564 m, 566~568.5 m, 610.4~613 m, 616~617 m, 619.8~622 m, 622.5~624.5 m, 626~628.5 m)에 대한 시추코어 분석결과를 종합하였다.
3. 시추코어 내 소단층 자료를 이용한 고응력장 복원
3.1 다중역산법
다중역산법은 Angelier’s (1984)의 직접역산법을 기반으로 한 고응력장 복원기법이다. 직접역산법은 기본적으로 단층면 상의 변위가 면상에 작용하는 최대 전단응력에 평행한 방향으로(Wallace, 1951; Bott, 1959) 즉, M-plane (movement plane)을 따라 발달한다고 가정한다(Marshak and Mitra, 1988). 최소자승법(least square method)을 이용하여 실제 소단층면 상에서 측정된 단층조선의 방향과 이론적인 단층조선 방향 간의 오차각(misfit angle)들의 합을 최소화함으로써 응력 텐서(stress tensor)를 구한다(Angelier, 1984, 1990). 이를 통하여 소단층 발달시 작용한 고응력 세 축의 방향성(σ1, σ2 그리고 σ3)과 차응력비(R = (σ2 - σ3)/(σ1 - σ3))를 추정할 수 있다.
다중역산법의 특징은 야외에서 수집한 소단층 자료를 k개의 소단층들로 이루어진 여러 개의 소집단으로 자동 분류하여, 각각의 소단층 집단에 대한 응력텐서를 직접역산법을 이용하여 구하는 것이다. 예를 들어, k=4로 지정하면 전체 소단층 자료로부터 4개의 소단층 자료로 구성된 각각 소단층 집단에 대한 응력텐서를 구한다. 소단층 자료로부터 얻어지는 전체 소집단의 수는 이항계수(binominal coefficient), NCk = N!/ k!(N-k)!, 0≤k≤N로 구한다. 여기서 N은 수집된 전체 소단층 자료의 수이며, N!은 N 계승으로, N!=N(N-1)···2·1로 정의된다. 다중역산법을 이용하여 응력텐서를 구하는데 있어 k의 값은 4 내지 5가 적절한 값으로 제시되었다(Yamaji et al., 2011). 이를 통해 구해진 유의미한 응력텐서는 입체투영망에서 유사한 형태와 색상을 가진 테드폴(tadpole)의 군집으로 도시된다.
3.2 소단층자료 수집
정방향 보정 없이 시추된 코어(un-oriented drill core) 내에 발달하는 소단층면의 방향성은 시추코어의 장축을 따라 작도한 참조선(arbitrary ‘North’)과 단열면이 이루는 각 관계를 Holcombe (2011)에 의해 제안된 α와 β 각들을 이용하여 측정하였다(그림 3). 시추코어 내 단층면 상에 발달하는 단층조선의 방향성과 이동감각은 선주각, γ를 이용하여 단층이동벡터로 측정하였다. 단층이동벡터는 오른손 법칙에 따라 단층면의 주향으로부터 반시계 방향으로 측정하였다. 예를 들어 단층이동벡터가 90°라는 것은 역이동성 단층을 의미한다(그림 3). 단층면의 상대적인 이동감각은 단층면 상에서 관찰되는 이동감각의 지시자들과 단층에 의해 절단된 층리면의 변위감각으로 구하였다. 여기서 단층의 이동벡터를 표기하기 위해 사용한 선주각, γ는 단층면의 경사방향으로부터 단순히 선구조가 이루는 각도만을 측정하는 Holcombe (2011)의 방법과는 차이가 있다. 여기서 제안한 단층이동벡터 측정방법은 다중역산법을 이용하여 소단층면의 자세를 간단히 계산하기 위해 변형한 것이다(Yamaji et al., 2011).

Drill core angle conventions. Alpha represents the acute angle between the core axis and the dip direction line on the planar structure (0-90°). Beta is the angle between the down-dip direction of the planar structure and a reference line along the core, measured in a clockwise sense (0-360°). Gamma represents slip direction of the hanging-wall fault block on a striated fault and defines an angle between slip direction and strike of the plane (right-hand rule), measured in an anti-clockwise sense (0-360°). In this case, a carrot-shaped groove on the striated fault indicates reverse movement of the hanging-wall fault block. The slip direction is denoted by gamma angle 90°.
시추코어들로부터 수집한 소단층 자료는 영상이미지와 시추코어에서 측정된 층리면의 평균자세를 이용하여 방향성을 복원하였다(그림 4). 층리와 엽리 같은 관통상 구조들(penetrative structures) 혹은 고지자기 선구조(paleomagnetic lineation) 등은 시추코어 내에 발달하는 면구조들의 방향성 복원을 위한 기준으로 이용되고 있다(Laing, 1977; Hinman, 1993; Scott and Berry, 2004). 우리는 장기4호공 영상이미지에서 확인되는 층리면의 평균자세를 시추코어 내에 발달한 면구조들의 방향성 복원을 위한 기준으로 사용하였으며, 이를 위해 장기4호공에서 획득된 공내영상이미지를 이용하였다(그림 4a). 즉, 영상이미지 분석을 통해 수집한 층리면의 평균자세를 피셔평균벡터로 구한 후(그림 4b), 이를 기준으로 시추코어를 수평회전시킴으로써, 시추코어 내에 발달하는 소단층 자료의 방향성을 복원하였다. 또한 층리면의 평균자세에 대한 지나친 가정을 피하고자 공내영상이미지로부터 상·하 20 m 구간에 한정하여 소단층자료 수집을 실시하였다.

(a) An example of the comparison of (left) BHTV and (middle) the corresponding core interval of JG-4 at depth of 602-603.5m. Then, the beddings (green) and fractures (black) on the image plotted on (right) tadpole log. The heads of tadpole symbol represent measured depths and dip angles (0-90°) while the tails indicate azimuths (0-360°). Solid and open circles indicate clear and uncertain discontinuities detected on the image, respectively; dip direction (white arrow) of the sinusoid, peak-to-peak amplitude hof sinusoid, diameter d of borehole, dip angle = arctan(h/d). (b) The equal-area lower hemisphere projection of poles to the beddings and fractures. the average orientation of poles to beddings calculated by fisher mean vector (square) with 5% significant level (small circle) in same manner below.
4. 복원결과
시추코어로부터 방향성이 복원된 단열들로부터 38개의 단층이동자료를 수집하였으며, 이를 입체투영망에 도시하였다(그림 5). 수집한 단층자료는 다중역산법을 이용하여 고응력을 복원하였으며, 이를 통해 3개의 고응력조건을 구하였다(그림 6). 응력 A는 남-북 방향으로 작용하는 σ1 축과 E-W 방향으로 대원 분포하는 σ3 축으로 구성되며, 그 차응력비는 약 0.2이다. 남-북 방향의 압축력 조건에서 σ3 축에 대한 해들을 상세히 살펴보면(그림 6), 매우 낮은 차응력비(약 0.2)의 집합들이 동-서 방향으로 대원분포 하나 약 40°~60°W로 선경사하는 영역에서 밀집된 형태를 보인다.
응력 B는 북북동-남남서 방향으로 작용하는 σ1 축과 수직한 σ3 축방향의 압축응력으로, 그 차응력비는 약 0.3이다. 또한 응력 C는 북서-남동 방향으로 작용하는 σ1 축과 거의 수직에 가까운 σ3 축을 갖는 압축응력으로, 그 차응력비는 약 0.5이다.

Equal-area lower-hemisphere projections of oriented fault-slip data obtained: 4 from the BHTV and 34 from the drill core samples in JG-4 borehole. Arrows indicate the relative slip direction of the hanging-wall fault block.

Paired equal-area Lower-hemisphere projections showing the result of MIM using oriented fault-slip data from the investigated drill core in this study. The left and right stereograms indicate the orientations σ1 and σ3 axis on the stereograms, respectively. The differential stress ratio, R, is represented by the color of the tadpole. The inversion method used the fault-combination number k = 4 and the enhanced factor e = 7; See Yamaji (2011) for details of these parameter.
5. 토 의
5.1 단층운동간의 상대적인 발달순서
다중역산법을 이용한 고응력장 복원결과는 남-북, 북북동-남남서 그리고 북서-남동 방향으로 작용하는 3개의 압축응력들을 보여준다. 이들 사이의 상대적인 선후관계는 시추코어에서 소단층간의 직접적인 상호절단 관계를 확인할 수 없었을 뿐만 아니라, 단층비지 또한 관찰되지 않아 연대측정 또한 불가능하였다. 따라서 우리는 이들 간의 상대적인 발달순서를 결정하기 위하여 시추코어 내에 발달하는 변형띠와 단층끌림에 대한 기하학적, 운동학적 분석을 실시하였으며, 고응력 복원을 통해 구한 응력조건들과 소단층 자료에 대한 오차각을 계산하여 이들 간의 관련성을 분석하였다.
발달된 구조들 간의 상대적 순서를 유추하기 위하여 변형띠와 단층끌림이 잘 발달해 있는 구간의 시추코어에 대한 3차원 스캔을 실시하였다(그림 7). 이 구간은 현무암질 암맥과 데사이트질 퇴적암의 관입경계로부터 상부로 약 1.5 m 구간이다(그림 7a). 펼친 3차원 스캔이미지에서 층리면들의 경사각이 하부로부터 상부로 가면서 급격하게 증가하는 것이 관찰된다(그림 7b). 또한 층리면들은 저각의 면구조에 의해 역이동성 감각으로 변위되어 있을 뿐만 아니라 부분적으로 역전되어 있기도 하다(그림 7c). 실체현미경 하에서 역이동성 감각으로 변위된 저각의 면구조들은 수mm의 폭으로 발달하며, 비불연속성(non-discrete) 면구조의 특징을 보인다(그림 7d). 이러한 특징은 변형띠의 발달특징으로 잘 알려져 있다(Peacock et al., 2016). 또한 시추코어의 하부에서는 현무암질암맥과 데사이트질 퇴적암의 경계면 상에서 역이동 우수감각의 단층조선이 관찰되는데(그림 7e), 이는 이 소단층이 현무암질 암맥의 관입 이후에 발달한 것임을 지시한다.

(a) A representative drill core from 566 to 571 m depth in borehole JG-4. (b) Unrolled 3D scanning image (ca. 1.5 m in length) from the top let side of the Box 119 (left) and its tadpole plot (right). (c) Close-up view of a part of the image. (d) Photomicrograph (×20) of slab section taken from core samples shows dragged-beddings across a deformation band with reverse shear sense. (e) (view to top) Fault striation (white arrow) on fault plane represents relative motion of foot-wall, indicating a reverse dextral fault.
구조요소들의 변형특성을 이해하기 위하여 3차원 이미지에서 분석한 변형띠와 층리면들의 폴(pole)에 대한 밀집도를 입체투영망에 도시하였다(그림 8). 변형띠와 층리면의 폴들은 대원 분포하고 있으며, 이들 대원의 축(π-axis)은 유사한 방향성을 보여준다. 또한 저각 역이동성 변형띠의 폴 밀집도는 북북동과 남남서 방향으로 공액상으로 분포한다(그림 8b). 이로 미루어 볼 때, 이들은 북북동-남남서 방향의 압축성 응력조건 하에서 동시기적으로 발달한 것으로 해석된다. 이러한 응력조건은 시추코어 내에 발달하는 소단층을 이용한 고응력 복원 결과와도 일치하는데, 이는 북북동-남남서 압축력 하에서 거의 동시기적으로 변형띠의 발달에 수반되어 단층끌림이 발달한 것을 지시한다.

Equal-area lower-hemisphere projections of poles to all oriented beddings from investigated core sample (a) and oriented deformation bands in the unrolled 3D scanning image (b) in borehole JG-4. Data was contour mapped above the 2% density, using a 1% area contour interval. black squares are the π-axis (trend/plunge) to the best fit great circles of poles to all beddings (290°/02°) and deformation bands (294°/05°), respectively.
고응력 복원을 통해 구한 응력조건과 현무암질암맥의 경계를 따라 발달하는 단층조선과의 관계를 알아보기 위하여 오차각 계산을 실시하였으며(표 1), 이는 소단층 자료 7번에 해당한다. 추정된 응력조건의 신뢰도는 소단층 자료에 대한 오차각 계산을 통해 확인해 볼 수 있다(Angelier, 1989; Nemcok and Lisle, 1995). 추정된 응력조건을 신뢰할 수 있는 오차각의 한계를 30°라고 가정했을 때(Nemcok and Lisle, 1995), 소단층 자료 7번은 응력 A와 B로 설명될 수 있다. 좀 더 작은 오차각을 갖는 응력조건이 더 신뢰할 만한 응력조건이라 한다면, 소단층 자료 7번은 응력 B로 설명이 가능할 것이다. 이는 변형띠와 단층끌림에 대한 응력조건과 일치하는 결과이다. 성동리층을 관입하고 있는 이 현무암질 암맥을 뇌성산 현무암류 관입활동의 산물이라 가정한다면, 이는 적어도 응력 B가 약 17 Ma 이후의 단층운동임을 지시한다(Kim, J.Y. et al., 1991; Kim, J.-S. et al., 2002).
한편, 추정된 응력조건 하에서 계산된 소단층들의 오차각들을 자세히 살펴보면(표 1), 수집된 소단층 자료에 대한 응력조건은 응력 A와 응력 C 만으로도 충분히 설명이 가능할 것으로 보인다. 즉, 응력 A는 응력 B로 설명할 수 있는 소단층들의 응력조건을 설명할 수 있다. 따라서 응력 B는 기각되어도 무방할 것이다. 이러한 해석이 옳다면, 응력 A는 변형띠의 발달과 관계된 응력조건으로 해석할 수 있다. 또한 응력 C에 해당하는 상당수의 소단층들이 고경사(>50~60°)하는 층리를 절단하고 있다. 분지 내 충전물들은 주로 25°내외 경사하는데(Kim et al., 2015), 이번 연구에서 수행한 공내영상이미지와 정밀 3차원 스캐이미지 분석결과들은 층리면의 자세가 소단층 주변에서 증가하는 것을 보여준다(그림 4와 7). 이러한 층리면들의 고경사 원인을 응력 A에 의한 단층끌림 혹은 경동(tilting)에 의한 것이라 한다면, 이는 응력 C가 응력 A보다 후기에 작용한 응력조건임을 지시한다.
5.2 인근 지역 고응력 복원결과와의 비교
이번 연구에서 우리는 장기4호공 시추코어 내에 발달한 소단층 자료를 분석하여 남-북 방향의 압축력(응력 A)과 이에 뒤따른 북서-남동 방향의 압축력(응력 C)을 구하였으며, 이들의 시기를 관입암의 연대에 근거하여 적어도 약 17Ma 이후로 추정하였다. 이를 한반도 남동부 지역에서 전기 마이오세 분지의 형성 이래 작용한 지구조응력조건들과 비교하였다(그림 9).
Choi et al. (1999)는 포항-울산지괴에서 소단층을 이용한 고응력장 복원을 통해 신생대 지구조사건들을 복원하였는데, 이는 시간적으로 양산단층 서편에서 수립된 지구조사건의 T4~T7에 해당한다(Choi, 1988). 최대압축력 방향만을 고려했을 때, 북동-남서 방향의 압축력(T4, Oligocene-middle Miocene), 서북서-동남동 방향의 압축력(T5, upper middle Miocene-late Miocene), 동-서 방향의 압축력(T6, Pliocene), 동북동-서남서 방향의 압축력(T7, Quaternary) 순이다. T4 시기, 포항-울산지괴에서 전기 마이오세 분지들은 북북서-남남동 방향을 갖는 양산단층과 후포단층의 우수향 재활에 수반된 국지적 북서-남동방향의 인장응력 하에서 형성된 인열분지로 해석되고 있다(Yoon and Chough, 1995; Kim and Park, 2006). 기존 고지자기연구는 분지 확장기에 포항-울산지괴는 양산단층의 서쪽에 비해 약 20~60° 시계방향으로 점진적으로 회전되었으며, 약 17 Ma 경에 이르러 이러한 회전은 종료된 것으로 알려져 있다(Son et al., 2015). 또한 암맥에 대한 연대 및 운동학적 분석결과는 이 분지에서 북서-남동 방향의 인장응력이 적어도 약 17 Ma까지 지속적으로 작용하였음을 보여준다(Kim, J.Y. et al., 1991; Kim, J.-S. et al., 2002). 따라서 이번 연구에서 구한 응력조건들이 포항-울산지괴의 회전이 종료된 이후, 즉 T4 이후의 압축응력 조건과 관계된 것임을 지시한다.
이번 연구를 통해 구한 응력조건들은 제4기 단층운동과 관계된 응력조건들과 상당히 유사성을 보인다. Choi et al. (2002)와 Choi et al. (2007)은 한반도 남동부 지역에서 확인된 제4기 활성단층에 대한 이전연구들을 종합하여 이들 단층운동과 관계된 3개의 지구조응력 방향을 구하였는데, (1) 히말라야 조산운동과 관계된 동북동-서남서 방향의 압축력, (2) 필리핀해판의 섭입과 관계된 서북서-동남동 내지 북서-남동 방향의 압축력, (3) 남-북 방향의 압축력은 명확하지는 않지만 앞서 두 지구조응력의 합력으로 추정하였다. 또한 Choi et al. (2007)은 제4기 단층들의 기존 연대측정 결과들을 바탕으로 이러한 지배적인 3개의 지구조 응력 방향이 반복적으로 나타나며, 이를 지구조적 ‘공격과 후퇴’로 설명한 바 있다.
Choi et al. (2002)은 왕산단층과 흥주리 지점 두 곳에서 남-북 방향의 압축력을 구하였는데, 전자는 σ3 축이 약 70°W 선경사하는 역단층성 응력조건에 해당하고, 후자는 σ3 축이 동-서 방향으로 놓이는 주향이동성 응력조건이다. 이들의 차응력비는 약 0.4로 유사하다. 왕산단층에서 구한 응력축의 방향성은 우리의 해와 상당히 유사성을 보이고 있다(Choi et al., 2002). 반면, 우리의 해에서는 E-W 방향에서 σ3 축들의 군집이 잘 관찰되지 않는다. 이는 연직방향으로 시추된 코어에서 약 70 m 길이의 구간에서만 소단층 자료를 수집하였기 때문에 고각 소단층의 자료수집이 어려웠기 때문으로 판단된다. 그러나 왕산단층에서 수집된 소단층 자료들은 아평행한 소단층들로 구성되어 있고, 흥주리 지점의 경우 대부분 공액상 단층조에서 수집된 자료를 통해 구한 응력조건인데, 이러한 소단층 자료를 이용한 응력비 추정은 상당히 어려운 것으로 알려져 있다(Dupin et al., 1993; Pollard et al., 1993).
하지만 이번 연구에서 시추코어로부터 소단층 자료를 수집하여 구한 고응력장 복원방법의 한계성 또한 존재한다. 첫째로, 소단층 자료들은 공내영상이미지분석을 통해 구한 층리면의 일관성에 대한 가정으로부터 출발한다. Kim et al. (2015)은 현무암질암류 주변에서 나타나는 성동리층의 자세교란이 현무암질화산체의 함몰에 의한 것으로 해석한 바 있다. 이는 장기4호공 성동리층 시추코어 구간에서 지층의 교란이 나타날 수 있다는 것을 의미한다. 그러나 이번 연구를 통해 수행한 공내영상이미지 분석결과는 분석된 구간 내에서 성동리층의 경사방향 일관성과 소단층 주변에서의 경사각 변화를 확인할 수 있었다. 성동리층의 소단층면 주변에서 층리의 경사는 60° 내외로 증가하나 거의 일관되게 남남서로 경사하고 있다. 이는 성동리층의 경사방향을 기준으로 시추코어로부터 소단층 자료를 수집하고 정방향 복원하여 고응력장 복원을 시도하는 것이 유의미함을 시사한다.
둘째로, 시추코어로부터 수집한 소단층 자료로부터 추정한 고응력장의 신뢰도이다. 소단층 자료를 이용하여 고응력장을 복원하기 위해서는 정밀하게 측정된 단층면의 방향성 및 이동벡터의 수집이 필수적이다. 이번 연구에서 층리면의 평균자세를 토대로 소단층 자료를 이용한 고응력장 복원은 신뢰하기 어려운 부분일 수도 있다. 그러나 연직방향으로 시추된 코어와 층리면에 대한 평균자세에 대한 가정에도 불구하고, 우리가 구한 남-북 방향의 응력조건은 기존 연구(Choi et al., 2002)에서 구한 남-북 방향의 응력조건들과 상당한 유사성을 보인다. 이는 적어도 시추코어라는 한정된 공간 내에서도 정밀하게 분석이 이루어진다면 소단층 자료를 이용하여 고응력장의 방향을 추정할 수 있다는 것을 의미한다. 반면, 추정된 응력비의 크기는 수집된 소단층 자료의 정밀도에 크게 좌우되므로, 이번 연구를 통해 추정한 응력비의 신뢰도에는 분명히 한계성이 존재한다. 따라서 시추코어의 소단층 자료를 통한 고응력장 복원의 불확실성을 극복하기 위해서는 경사시추 및 시추공 전체 구간에 대한 공내영상이미지 획득이 필요할 것으로 판단된다.
이번 연구에서 북서-남동 방향의 압축력은 남-북방향의 압축력에 뒤이은 응력조건으로 판단된다. 앞서 설명한 남-북 방향의 압축력이 제4기 단층운동과 관계된 응력조건과 부합된다면 후기의 북서-남동 방향의 압축력 또한 제4기 단층운동과 관계된 것으로 해석된다. 불행하게도 이번 연구에서 수집한 소단층들의 면상에서는 단층암이 발달하지 않고 있어, 이번 연구에서 구한 응력조건들의 연대를 명확히 규명할 수 없었다. 그러나 이번 연구를 통해 구한 응력조건들은 한반도 남동부에서 3개 이상의 지구조응력조건(광역적 또는 지역적)이 존재할 가능성에 대한 면밀한 검토가 필요하다는 점을 시사한다. 또한 한반도 남동부 지역에서 발달하는 단층대의 재활가능성 평가에 있어 동북동-서남서 내지 동-서로 작용하는 압축력을 공통된 현생 지구조응력조건으로 고려하는 것은 상당히 위험할 수 있다는 것을 시사한다.
연구지역을 포함한 한반도 남동부 지역에서는 최근 경주지진 관련단층을 비롯한 제4기 단층의 존재가 보고되고 있으며, 저준위 방사성폐기물처분장을 비롯한 원자력발전시설과 이산화탄소 지중저장을 위한 특성화 부지가 위치하고 있다. 이들 부지의 안정성 평가를 위해 부지 인근에 발달하는 단층대의 지질구조적 발달특성 및 현생 지구조응력 방향에 대한 추가적인 정밀연구가 필요할 것으로 판단된다.
6. 결 론
이번 연구는 제4기 단층의 운동특성과 지구조응력조건과의 관련성을 규명하기 위해 장기분지 장기 4호공의 시추코어 일부 구간에서 수집한 소단층 자료를 이용하여 고응력장 복원을 실시하였다. 이를 통해 구한 응력조건들은 남-북 방향의 압축력과 북서-남동 방향의 압축력이다. 시추코어 내에 발달하는 변형띠와 단층끌림 및 소단층 자료의 오차각 계산을 통해 남-북 방향의 압축력에 뒤이어 북서-남동방향의 압축력이 작용한 것으로 해석되었다. 한반도 남동부 지역에서 수행된 고응력복원 연구들과의 비교를 통해 이들이 잠재적으로 제4기 단층운동과 관련되었을 수 있음을 제시하게 되었다. 또한 제4기 단층운동과 관계된 복수의 지구조응력조건의 존재가능성 및 그 원인에 대한 심도 있는 연구가 있어야 할 것으로 판단된다. 이러한 연구결과는 본 연구지역 내에서 수행되고 있는 이산화탄소 지중저장 특성화 및 그 일대에 위치한 원전부지의 안정성평가 및 차후의 지진재해대책을 수립하는데 있어 중요한 역할을 할 수 있을 것으로 사료된다.
Acknowledgments
이 연구는 2014년도 미래창조과학부 (재)한국이산화탄소포집 및 처리연구개발센터의 지원(NRF-2014M1A8A1049339)과 BK21 플러스 사업 지구환경재해시스템 사업단의 일부 지원으로 수행되었다. 이 연구를 위해 야외자료 수집과 시추코어 분석에 협력해준 지질구조재해연구실원들과 공내영상이미지를 제공해주신 한국지질자원연구원 황세호 박사님과 3차원 코어 이미지스캔에 도움을 주신 한국지질자원연구원 현혜자 박사님께 깊이 감사드린다. 꼼꼼한 지적과 유익한 의견을 통해 이 논문의 질을 더욱 개선할 수 있도록 협조하여 주신 장천중 박사님과 이희권 교수님, 그리고 편집위원이신 손문 교수님께도 진심으로 감사를 드린다.
REFERENCES
-
Angelier, J., (1984), Tectonic analysis of fault slip data sets, Journal of Geophysical Research, 89, p5835-5848.
[https://doi.org/10.1029/jb089ib07p05835]
-
Angelier, J., (1989), From orientation to magnitudes in paleostress determinations using fault slip data, Journal of Structural Geology, 11, p37-50.
[https://doi.org/10.1016/0191-8141(89)90034-5]
-
Angelier, J., (1990), Inversion of field data in fault tectonics to obtain the regional stress-III. A new rapid direct inversion method by analytical means, Geophysical Journal International, 103, p363-376.
[https://doi.org/10.1111/j.1365-246x.1990.tb01777.x]
-
Bott, M.H.P., (1959), The mechanics of oblique slip faulting, Geological Magazine, 96, p109-117.
[https://doi.org/10.1017/s0016756800059987]
-
Choi, J.H., Kim, J.W., Murray, A.S., Hong, D.G., Chang, H.W., and Cheong, C.-S., (2009), OSL dating of marine terrace sediments on the southeastern coast of Korea with implications for Quaternary tectonics, Quaternary International, 199, p3-14.
[https://doi.org/10.1016/j.quaint.2008.07.009]
- Choi, P.Y., (1988), Tectonic evolution of SE Korea since the Early Cretaceous, In: Koh, H.J., and Choi, Y.S. (eds.), Tectonic Evolution of East Asia, The first joint meeting of Japanese and Korean Structure and Tectonic Research Group, p113-121.
- Choi, P.Y., Kwon, S.-K., Hwang, J.-H., and Lee, S.R., (1999), Paleostress analysis of Southeast Korea: Tectonic sequence and timing of block rotation of the Pohang-Ulsan area, Gondwana Research, 2, p532-537.
- Choi, P.Y., Lee, H.-K., and Chwae, U., (2007), Tectonic 'aggression and retreat' in the Quaternary tectonics of southern Korea, Journal of the Geological Society of Korea, 43, p415-425, (in Korean with English abstract).
- Choi, P.Y., Ryoo, C., Kwon, S., Chwae, U., Hwang, J., Lee, S.R., and Lee, B., (2002), Fault tectonic analysis of the Pohang-Ulsan area, SE Korea: Implications for active tectonics, Journal of the Geological Society of Korea, 38, p33-50, (in Korean with English abstract).
-
Chough, S.K., and Barg, E., (1987), Tectonic history of Ulleung basin margin, East Sea (Sea of Japan), Geology, 15, p45.
[https://doi.org/10.1130/0091-7613(1987)15<45:thoubm>2.0.co;2]
- Dezayes, C., Villemin, T., Genter, A., Traineau, H., and Angelier, J., (1995), Analysis of fractures in boreholes of hot dry rock project at Soultz-sous-Forêts (Rhine Graben, France), Scientific Drilling, 5, p31-41.
-
Dupin, J.-M., Sassi, W., and Angelier, J., (1993), Homogeneous stress hypothesis and actual fault slip: a distinct element analysis, Journal of Structural Geology, 15, p1033-1043.
[https://doi.org/10.1016/0191-8141(93)90175-a]
-
Gwon, S., and Kim, Y.-S., (2016), Interpretation of Deformation History and Paleostress Based on Fracture Analysis Exposed in a Trench, The Journal of Engineering Geology, 26, p33-49, (in Korean with English abstract).
[https://doi.org/10.9720/kseg.2016.1.33]
-
Hinman, M., (1993), A lotus 1-2-3 diamond drillhole structural manipulation spreadsheet: Drillcore structural data generation, Computers & Geosciences, 19, p343-354.
[https://doi.org/10.1016/0098-3004(93)90091-i]
- Holcombe, R., (2011), Oriented drillcore: measurement and calculation procedures for structural and exploration geologists, http://www.holcombecoughlin.com/HCA_ downloads.htm.
- Jun, M.-S., and Jeon, J.S., (2010), Focal Mechanism in and around the Korean Peninsula, Jigu-Mulli-wa-Mulli-Tamsa, 13, p198-202, (in Korean with English abstract).
- Kim, H., Song, C.W., Kim, J.-S., Son, M., and Kim, I.-S., (2008), Tertiary geological structures and deformation history of the southern Tsushima Island, Japan, Journal of the Geological Society of Korea, 44, p175-198, (in Korean with English abstract).
- Kim, J.-S., Son, M., Kim, J.S., and Kim, I., (2002), Tertiary dyke swarms and their tectonic importance in the southeastern part of the Korean peninsula, Journal of the Petrological Society of Korea, 11, p169-181, (in Korean with English abstract).
- Kim, J.Y., Jung, C.Y., and Yoon, S., (1991), Early and Middle Miocene dike swarms and regional tectonic stress field in the Janggi Peninsula (1), Journal of the Geological Society of Korea, 27, p330-337, (in Korean with English abstract).
- Kim, M.-C., Gihm, Y.S., Son, E.-Y., Son, M., Hwang, I.G., Shin, Y.J., and Choi, H., (2015), Assessment of the potential for geological storage of CO2 based on structural and sedimentologic characteristics in the Miocene Janggi Basin, SE Korea, Journal of the Geological Society of Korea, 51, p253-271, (in Korean with English abstract).
-
Kim, M.-C., Jung, S., Yoon, S., Jeong, R.-Y., Song, C.W., and Son, M., (2016), Neotectonic crustal deformation and current Stress field in the Korean Peninsula and their tectonic implications: A review, The Journal of the Petrological Society of Korea, 25, p169-193, (in Korean with English abstract).
[https://doi.org/10.7854/jpsk.2016.25.3.169]
- Kim, M.-C., Kim, J., Jung, S., Son, M., and Sohn, Y.K., (2011), Classification and stratigraphy of the Miocene basin fills in the northern area of the Janggi-myeon, Pohang, SE Korea, Journal of the Geological Society of Korea, 47, p585-612, (in Korean with English abstract).
-
Kim, Y.-S., and Park, J.-Y., (2006), Cenozoic deformation history of the area around Yangnam-Yangbuk, SE Korea and its tectonic significance, Journal of Asian Earth Sciences, 26, p1-20.
[https://doi.org/10.1016/j.jseaes.2004.08.008]
-
Laing, W.P., (1977), Structural interpretation of drill core from folded and cleaved rocks, Economic Geology, 72, p671-685.
[https://doi.org/10.2113/gsecongeo.72.4.671]
- Lallemant, S.J., Byrne, T., Maltman, A., Karig, D., and Henry, P., (1993), Stress tensors at the toe of the Nankai accretionary prism: an application of inverse methods to slickenlined faults, Proceedings Ocean Drilling Program Scientific Results, Part B, 131, p103-122.
- Lee, H.K., Moon, H.-S., Min, K.-D., Kim, I.-S., Yun, H., and Itaya, T., (1992), Paleomagnetism, stratigraphy and geologic structure of the Tertiary Pohang and Changgi basins; K-Ar ages for the volcanic rocks, Journal of the Korean Institute of Mining Geology, 25, p337-349.
-
Lee, H.-K., and Schwarcz, H.P., (2001), ESR dating of the subsidiary faults in the Yangsan fault system, Korea, Quaternary Science Reviews, 20, p999-1003.
[https://doi.org/10.1016/s0277-3791(00)00055-x]
- Lee, J.I., (2000), Provenance and thermal maturity of the lower Cretaceous Gyeonsang Supergroup, Korea, Ph.D. thesis, Seoul National University, Seoul, p129.
- Marshak, S., and Mitra, G., (1988), Basic methods of structural geology, Prentice Hall, Englewood Cliffs, New Jersey, p446.
-
Martin, P., and Bergerat, F., (1996), Palaeo-stresses inferred from macro-and microfractures in the Balazuc-1 borehole (GPF programme). Contribution to the tectonic evolution of the Cévennes border of the SE Basin of France, Marine and Petroleum Geology, 13, p671-684.
[https://doi.org/10.1016/0264-8172(95)00063-1]
-
Nemcok, M., and Lisle, R.J., (1995), A stress inversion procedure for polyphase fault/slip data sets, Journal of Structural Geology, 17, p1445-1453.
[https://doi.org/10.1016/0191-8141(95)00040-k]
-
Okada, A., Watanabe, M., Sato, H., Jun, M.S., Jo, W.R., Kim, S.K., Jeon, J.S., Chi, H.C., and Oike, K., (1994), Active fault topography and trench survey in the central part of the Yangsan fault, Southeast Korea, Journal of Geography, 103, p111-126, (in Japanese with English abstract).
[https://doi.org/10.5026/jgeography.103.2_plate3]
-
Park, Y., Ree, J., and Yoo, S., (2006), Fault slip analysis of Quaternary faults in southeastern Korea, Gondwana Research, 9, p118-125.
[https://doi.org/10.1016/j.gr.2005.06.007]
-
Peacock, D.C.P., Nixon, C.W., Rotevatn, A., Sanderson, D.J., and Zuluaga, L.F., (2016), Glossary of fault and other fracture networks, Journal of Structural Geology, 92, p12-29.
[https://doi.org/10.1016/j.jsg.2016.09.008]
-
Pollard, D.D., Saltzer, S.D., and Rubin, A.M., (1993), Stress inversion methods: are they based on faulty assumptions?, Journal of Structural Geology, 15, p1045-1054.
[https://doi.org/10.1016/0191-8141(93)90176-b]
-
Reches, Z., (1978), Analysis of faulting in three-dimensional strain field, Tectonophysics, 47, p109-129.
[https://doi.org/10.1016/0040-1951(78)90154-3]
-
Ree, J.-H., Lee, Y.-J., Rhodes, E.J., Park, Y., Kwon, S.-T., Chwae, U., Jeon, J.-S., and Lee, B., (2003), Quaternary reactivation of Tertiary faults in the southeastern Korean Peninsula: Age constraint by optically stimulated luminescence dating, Island Arc, 12, p1-12.
[https://doi.org/10.1046/j.1440-1738.2003.00372.x]
-
Rocher, M., Lacombe, O., Angelier, J., Deffontaines, B., and Verdier, F., (2000), Cenozoic folding and faulting in the south Aquitaine Basin (France): Insights from combined structural and paleostress analyses, Journal of Structural Geology, 22, p627-645.
[https://doi.org/10.1016/s0191-8141(99)00181-9]
-
Scott, R.J., and Berry, R.F., (2004), A new method for obtaining and quantifying the reliability of structural data from axially-oriented drill core using a fabric of known orientation, Journal of Structural Geology, 26, p643-658.
[https://doi.org/10.1016/j.jsg.2003.08.017]
-
Son, M., Song, C.W., Kim, M.-.C., Cheon, Y., Cho, H., and Sohn, Y.K., (2015), Miocene tectonic evolution of the basins and fault systems, SE Korea: Dextral, simple shear during the East Sea (Sea of Japan) opening, Journal of the Geological Society, 172, p664-680.
[https://doi.org/10.1144/jgs2014-079]
- Wallace, R.E., (1951), Geometry of shearing stress and relation to faulting, The Journal of geology, p118-130.
-
Yamada, Y., and Yamaji, A., (2002), Determination of paleostresses from mesoscale shear fractures in core samples using the multi-inverse method, Journal of Petroleum Geology, 25, p203-218.
[https://doi.org/10.1111/j.1747-5457.2002.tb00004.x]
-
Yamaji, A., (2000), The multiple inverse method applied to meso-scale faults in mid-Quaternary fore-arc sediments near the triple trench junction off central Japan, Journal of Structural Geology, 22, p429-440.
[https://doi.org/10.1016/s0191-8141(99)00162-5]
- Yamaji, A., Sato, K., and Otsubo, M., (2011), Multiple Inverse Method software package-User’s Guide, Kyoto University, p37.
- Yang, J.S., (2006), Quaternary fault in southeastern peninsula, Ph D. thesis, Gangwon National University, Chuncheon, p378, (in Korean).
-
Yoon, S.H., and Chough, S.K., (1995), Regional strike slip in the eastern continental margin of Korea and its tectonic implications for the evolution of Ulleung Basin, East Sea (Sea of Japan), Geological Society of America Bulletin, 107, p83-97.
[https://doi.org/10.1130/0016-7606(1995)107<0083:rssite>2.3.co;2]