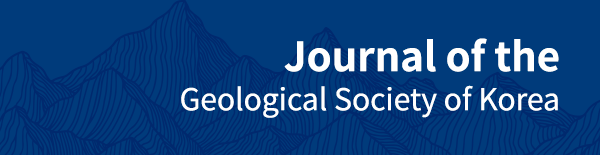
대규모 지진에 수반된 지표파열특성에 관한 최근 연구동향
Copyright © 2017 The Geological Society of Korea
This is an Open Access article distributed under the terms of the Creative Commons Attribution Non-Commercial License (http://creativecommons.org/licenses/by-nc/3.0/ which permits unrestricted non-commercial use, distribution, and reproduction in any medium, provided the original work is properly cited.
초록
활성단층에 대한 고지진학적 연구를 수행하여 고지진의 발생 위치, 시기, 규모 등을 밝히는 것은 장래에 발생 가능한 지진의 특성을 파악하는데 도움을 주며, 이는 지진재해도 작성의 기초자료로 활용가능하기 때문에 지진피해를 줄이는 데 필수적이다. 최근 20년 동안 새롭게 개발된 원격탐사기법을 활용하여 지진에 수반된 지표파열 및 활성단층을 지도화하는 많은 연구가 수행되었다. 특히 단층의 기하와 변위 분포를 바탕으로 단층에 내재된 특성이 지진파열의 동역학적 전파과정 및 지진파열의 시공간적 이력에 미치는 영향에 대한 연구가 이어졌다. 다수의 연구결과들이 공통적으로 지시하는 것은 지진원 규모의 단층분절시스템이 지진파열의 거동 및 단층진화에 핵심적인 역할을 한다는 것이다. 이는 주어진 활성단층을 대상으로 지진의 재발특성을 이해하기 위해서는 단층의 분절시스템에 기초한 고지진학적 연구가 필요하다는 점을 시사한다. 이 논문에서는 지진지표파열 및 활성단층 연구와 관련된 일반적인 개념을 비롯해 연구자료, 연구방법, 연구사, 최신 연구동향에 대한 간략한 검토와 최근 연구에서 도출된 주요 연구결과를 소개하였다. 마지막으로 한반도 남동부에 위치한 양산단층의 고지진학적 연구결과를 바탕으로 양산단층의 분절화를 새롭게 제안하였으며, 우리나라 활성단층 연구 및 이와 관련된 지진재해평가의 방향에 대해 토의하였다.
Abstract
In given active faults, to explore the location, timing, and size of large paleo-earthquakes is the most essential work to examine characteristics of future large earthquakes, which can be facilitated for seismic hazard assessment and loss mitigation. During the last 20 years, many studies have been conducted for mapping of earthquake surface ruptures and/or active faults, which is greatly indebted to the advances in remote sensing techniques. In particular, based on fault geometry and slip distribution, many studies have tried to understand the effects of fault inheritances on coseismic rupture dynamics as well as temporal and spatial rupture history over multiple earthquake cycle. These studies commonly indicate that seismogenic-scale fault segmentation plays a key role in earthquake rupture behavior and fault evolution. This implies that paleoseismological investigations should be carried out based on fault segmentation in order to infer earthquake recurrence characteristics in a given active fault. Here, after brief reviewing of general concepts, materials/methods, and history/trend of the study on earthquake surface ruptures and active faults, we introduce major results of the most recent studies. Finally, we suggest a possible segmentation system of the Yangsan fault in southeast Korea based on previous paleoseismological studies, and discuss research strategies for further active fault researches in Korea and its associated seismic hazard assessment.
Keywords:
strike-slip fault, earthquake, surface rupture, fault segment, slip distribution, seismic hazard assessment키워드:
주향이동단층, 지진, 지표파열, 단층분절, 변위분포, 지진재해도평가1. 서 론
지난 2016년 9월 12일, 경상북도 경주시 인근에서 두 차례의 규모 5 이상 지진(M 5.1 & M 5.8 (KMA); MW 4.9 & MW 5.4 (USGS))이 약 48분 간격으로 발생하였다. 이로 인해 경주를 중심으로 문화재를 포함한 일부 시설물의 벽체, 지붕, 유리, 담장 등이 파손되었고, 전국 대부분의 지역에서 많은 사람들이 강력한 지진동을 겪었다. 최근 전 세계적으로 발생하고 있는 대형 지진재해 사례(e.g., 쓰나미에 의한 원자력 발전소 안전사고가 발생한 2011년 MW 9.0의 일본 Tohoku 지진; 약 9,000명의 사망자를 낸 2015년 MW 7.8의 네팔 Gorkha 지진)와 더불어, 이번 경주 연쇄 지진으로 인해 우리나라 사회 전반에 걸쳐 지진재해에 대한 불안감이 고조되었고, 국가적 차원의 지진대응 및 방재시스템 구축에 대한 중요성이 부각되었다.
지진재해를 평가하고 대비하기 위한 가장 근본적인 방법은 고지진(paleo-earthquake)의 위치, 시기, 규모 등을 추론하고, 이를 바탕으로 지진의 재발특성을 파악하는 것이다. 비교적 대형 지진재해를 유발할 가능성이 높은 대규모 육상지진의 경우 주로 활성단층(active fault)에 의해 변형된 지형 및 지표지질을 분석함으로써 고지진 정보를 추론하는 것이 중요한데, 그 이유는 다음과 같다: 1) 지진은 단층에서 반복적으로 일어나는 일시적 ‘미끌림 운동(slip)’의 결과이다. 즉 판구조 운동에 의한 응력에너지가 주로 지각 내의 단층을 따라 서서히 누적되고, 한 순간의 단층운동(즉 지진)으로 갑작스럽게 방출되는 것이다(Reid, 1910). 2) 일반적으로 활성단층은 현생 지구조·지진원 환경에서 단층운동을 겪었고, 가까운 미래에 지진을 유발할 가능성이 높은 단층이다(Slemmons and McKinney, 1977). ‘활성단층’이라는 용어의 세부정의 및 기준은 나라별로 다소 상이할 수 있는데, 이는 각 나라가 서로 다른 지구조 환경에 위치하기 때문에 지진의 재발특성이 다르기 때문이다. 3) 지진성 단층운동 시 파열되는 단층부를 지진파열(earthquake rupture)이라 하는데, 대규모 지진의 경우 지진파열면이 지표면에 노출되는 지표파열(surface rupture)을 수반한다. 즉 현재 우리가 지표면에서 인지할 수 있는 활성단층의 자취(trace)는 대규모 지진 시 발생한 지표파열이 누적된 결과라 할 수 있다. 4) 지진계를 통해 관측된 계기지진 또는 역사문헌에 기록된 역사지진 자료는 짧은 기간 동안의 최근 지진에 대한 정보만을 포함하고 있기 때문에, 우리나라와 같이 상대적으로 발생주기가 긴 대규모 지진의 재발 특성을 추론하는데 제한적이다(McCalpin and Nelson, 2009). 결과적으로 지형 및 지표지질의 변형에 기초한 활성단층 연구는 시공간적인 대규모 고지진의 이력, 즉 주어진 단층에서의 응력에너지 누적 및 방출과정을 이해하는데 그 목적이 있다.
비록 지진의 규모는 여러 가지 방법으로 표현될 수 있지만, 최근 많이 사용하고 있는 모멘트 규모(MW)의 경우 지진파열의 면적과 평균 변위 및 이를 둘러싼 암석의 전단강도 등을 통해 산정한다(Hanks and Kanamori, 1979). 지진파열의 면적은 파열된 단층부의 길이와 폭을 통해 구할 수 있다. 일반적으로 지진의 규모와 빈도는 지진의 규모에 따라 크게 두 가지 형태의 상관관계를 보이는데, 소-중규모 지진의 경우 구텐베르크 리히터 관계식(Gutenberg and Richter, 1954)으로 알려진 멱함수 분포를 보이는 반면 대규모 지진의 경우 정규분포에 가까운 양상을 보인다(Wesnousky, 1994; Ben-Zion, 2008; 그림 1a). 이는 깊이(즉 온도·압력 조건)에 따라 유발될 수 있는 지진동시성 응력강하(stress drop)량이 다르다는 점을 바탕으로, 최대 지진동시성 응력강하가 일어날 수 있는 깊이에 대한 지진파열의 관통 여부와 관련된 것으로 해석된 바 있다(Zielke and Arrowsmith, 2008; 그림 1b). 즉 소-중규모 지진은 최대 응력강하 깊이를 기준으로 대개 그 상부 또는 하부의 단층면이 제한적으로 파열되는 부분파열(partial rupture)을 수반하는 반면 대규모 지진은 최대 응력강하 깊이를 포함한 전체 지진원 폭(seismogenic width)의 단층면이 파열되는 전체파열(full rupture)을 수반함으로써, 지진파열 폭의 갑작스런 변화에 따라 소-중규모와 대규모 지진의 구분이 가능하고 이들이 서로 다른 규모-빈도 분포를 보인다는 것이다(그림 1). 다만 대규모 지진은 지진파열의 폭이 지진원 깊이(seismogenic depth)를 초과할 수 있고, 이 때 지진원 깊이 아래에서는 ‘비지진성 단층운동(aseismic slip or creep)’이 발생하는데(King and Wesnousky, 2007), 이는 해당 깊이에서의 소규모 지진(deep tectonic tremor) 또는 지진 후 변형(postseismic deformation)을 통해 확인된 바 있다(Reiliner et al., 2000; Shelly, 2010).

Schematic representations of (a) single-fault magnitude frequency relation and (b) the down-dip rupture width of full and partial rupture earthquakes along a fault in relation to the extent of seismogenic depth (from Zielke et al., 2015). See text for details.
한편 아무리 큰 규모의 지진이라 하더라도 한 번의 지진에 의해 단층의 전체 길이가 파열되는 현상은 잘 발생되지 않는다. 일반적으로 단층은 서로 이웃한 여러 분절(segment)로 구성되어 있는데, 대개 한 번의 지진으로 인해 일부 분절만이 파열된다. 즉 개별적인 지진에 의한 지표파열의 길이는 단층의 총길이에 비해 작다고 할 수 있다. 일반적으로 지진파열은 이웃하는 두 단층분절(fault segment)의 경계부에서 개시 및 종결되는 특성을 보이는데, 이는 단층의 연속성이 불량한 분절경계부와 연속성이 양호한 각 단층분절의 중심부 간 응력누적의 불균형으로 인해 개별적인 지진에 의한 지진파열 전파 시 분절경계부가 응력전달을 저항(즉 지진파열의 전파 및 확장을 방해)하는 역할을 하고 나아가 지진종결을 유발하며 이 때 잔존하는 잔여응력이 다시 분절경계부에서의 새로운 지진을 유발하는 것이다(King and Nabelek, 1985; Nielsen and Knopoff, 1998; Duan and Oglesby, 2006). 따라서 주어진 단층에 대한 고지진의 시공간적 이력을 해석하기 위해서는 ‘단층 분절화(fault segmentation)’ 개념을 바탕으로 각 단층분절에 대한 고지진 정보(e.g., 시기, 변위량)를 확보해 이를 종합적으로 분석할 필요가 있다. 이를 통해 해당단층에서 발생할 수 있는 지진의 최대규모 및 대규모 지진의 재발주기 등을 추론할 수 있고, 이러한 정보는 단층 주변지역에 대한 지진재해를 평가함에 있어 매우 중요한 기초자료로 활용될 수 있다. 따라서 활성단층 및 고지진 연구는 공공의 안녕과 이익을 확보하기 위한 필수과제로 매우 가치 있는 연구주제라 할 수 있다.
대규모 지진에 수반된 지표파열에 관한 연구는 미국의 주도적인 역할과 일부 유럽 국가 및 일본 등을 중심으로 꾸준하게 발전하고 있다(e.g., Field et al., 2014). 특히 2000년대 초 이후 급속한 발전을 이루었는데, 이는 1) 원격탐사기법을 이용한 지표관측기술의 발달 및 정밀 활성단층지도 구축, 2) 대형 단층시스템의 분절화 규명 및 각 단층분절에 대한 양질의 고지진 자료 축적, 3) 이러한 자료에 기초한 컴퓨터 시뮬레이션 분석기술의 발달 등에 의한 결과로 판단된다. 반면 국내 활성단층 연구는 최근 수년간 다소 정체된 것으로 평가되는데, 이는 연구인력과 인프라의 부족 및 최신연구기술을 도입하는데 실패한 결과로 판단된다. 대형 지진재해를 직접 경험하지 못한 학습효과의 부족으로 국가적 차원의 연구과제가 수행된 적이 없고, 특정 지역이나 원전시설 등 주요 시설물에 대한 지진재해를 평가함에 있어 실질적인 자료획득을 위한 대규모 투자보다는 과거 조사된 고지진 자료에 의존하는 경향이 있는 실정이다. 이러한 한계점은 여전히 신뢰할 수 있는 활성단층지도가 구축되어 있지 않다는 점에서 잘 드러난다. 최근 일부 국내 연구팀에서 꾸준한 연구를 수행하고 있으나(Choi, S.-J. et al., 2014; Choi, J.-H. et al., 2015a, 2015b; Lee et al., 2015, Im et al., 2016), 연구비 규모 등의 제약으로 여전히 개별적인 지점에서의 지표지질 변형에 대한 조사가 제한적으로 실시되고 있을 뿐 단층의 분절화 또는 고지진의 시공간적 이력 등에 관한 보다 실질적이고 거시적인 관점의 연구가 미흡한 실정이다.
이 논문에서는 주로 주향이동단층에서의 ‘지진지표파열(earthquake surface rupture)’에 대해 최근 발표된 연구들을 검토 및 고찰하였다. 이를 통해 우리나라 활성단층 연구 및 지진재해 평가에 적용될 수 있는 주요 연구결과를 소개하고자 하였다. 또한 여진군집(aftershock cluster), 연쇄지진(sequential earthquake), 이동성지진(migrating earthquake), 지진공백(seismic gap) 등과 같은 대규모 지진과 관련된 특징적인 지진발생추이와 단층의 거동특성에 대해 토의하였다. 마지막으로 우리나라의 대표적인 대규모 주향이동단층 중 하나인 양산단층의 분절시스템과 향후 국내 활성단층 연구의 발전방향에 대해 논의하였다.
2. 연구자료 및 전제조건
일반적으로 고지진에 의한 지표파열은 지형학적 및 층서학적 변형(i.e., geomorphic and stratigraphic deformation)으로 기록된다. 지형학적 변형은 대개 지표파열 또는 단층의 분포와 기하(geometry) 및 이들의 자취를 따른 변위분포(slip distribution) 등 고지진의 공간적인 이력을 분석하기 위한 자료로 활용되고(그림 2), 대개 개별적인 지점에서의 지표지질단면에 기록되는 층서학적 변형은 주로 지진의 횟수 및 시기와 관련된 시간적 정보를 제공한다. 대부분의 지진지표파열은 대규모 지진에 의해 발생하지만, 종종 부분파열과 관련된 중규모 지진에서도 발생할 수 있다(그림 1b). 후자의 경우 지표파열의 발생여부는 진원지의 깊이 등 여러 요소에 좌우될 수 있으며, 지금까지 지표파열을 일으킨 지진의 최소 규모는 약 M 5.5로 알려져 있다(Bonilla, 1988). 상대적으로 작은 규모의 지진에 의한 지표파열은 변위가 거의 없거나 매우 작은 지표균열(ground crack) 형태를 보이기도 한다(그림 2b).

(a, b) Geomorphic offsets along the surface ruptures associated with the 1957 MW 8.1 Gobi-Altay, Mongolia, earthquake (from Choi et al., 2012) and the 2016 MW 6.2 Central Italy earthquake (photo taken on 14th Sep. 2016 by N. Feuillet in IPGP), respectively. Note that geomorphic markers can show variations in offset preservation due to different amounts of coseismic slip as well as different climatic conditions. (c - e) An example of the high-resolution satellite (HRS) image-based offset measurements, complemented by field works, along the 1905 MW 8.3 Bulnay earthquake surface rupture in Mongolia (from Choi et al., 2015c). Note that the estimated slip amounts based on the HRS images and field observations are almost the same.
이 논문에서 주로 다루는 내용은 지진지표파열에 의한 지형학적 변형에 관한 것으로 ‘변위(displacement or offset)’라는 용어는 대부분 지표면에서 인지되는 지형학적 변위를 의미한다. 일반적으로 변위는 지진이 발생하기 이전의 상태로 복원 가능한 지형(또는 인공시설물)을 이용해 측정할 수 있는데, 주로 단층을 가로지르는 선적인 형태의 지형이 변위 지시자(i.e., geomorphic marker)로 활용된다. 변위는 각각의 개별적인 지진과 관련된 ‘지진동시성 변위’와 여러 번의 지진으로 변위가 축적되었음을 의미하는 ‘누적변위’로 구분된다(그림 2c). 각 변위자료의 정확성은 불확실도(uncertainty)와 신뢰도(confidence)를 바탕으로 평가될 수 있다. 불확실도는 복원 가능한 지형의 범위 또는 지표관측 영상자료의 해상도에 따라 달라질 수 있으며, 각 자료값의 오차범위를 통해 표현된다. 신뢰도는 지형 복원의 질적 우수성과 관련된 지표로 조사자의 주관적인 판단이 포함될 수 있는데, 주로 지표파열의 폭(또는 기하학적 복잡성)이나 변위지시자의 선명도(sharpness) 등에 의해 결정된다.
지형학적 변위에 기초한 연구는 지표파열의 변위량 및 변위분포가 지진파열 심부의 변위특성과 거의 유사하다는 가정을 기초로 한다(Scholz, 2002). 한편 GPS(Global Positioning System) 또는 InSAR (Interferometric Synthetic Aperture Radar) 등의 원격탐사자료(i.e., far-field data)를 이용한 연구결과, 개별적인 지진에 의한 지진동시성 변위가 심부에 비해 지표면을 포함한 최상부 지각(0 - 2 km)에서 비교적 급격하게 감소하는 경향이 보고된 바 있다(e.g., Fialko et al., 2005). 이는 최상부 지각이 지진의 휴식기 동안 비지진성 단층운동을 겪으며 이로 인해 누적되는 응력에너지의 양이 심부에 비해 작기 때문으로 추정된 바 있지만(Barbot et al., 2008; Kaneko and Fialko, 2011; see an aseismic zone in the uppermost crust in Figure 1b), 현재까지 이러한 주장을 뒷받침할 만한 최상부 지각에서의 차별적인 비지진성 단층운동이나 ‘지진 후 변위(postseismic slip)’가 보고된 적은 없다. 반면 고해상도 영상자료 및 야외지질조사(i.e., near-field data)에 기초한 지표파열 연구결과, 주단층(main fault)을 따른 변위의 감소는 지표면 근처에서의 단층 기하특성(e.g., 수직적 곡면현상 또는 단층분지)과 관련된 2차적인 지표변형으로부터 기인한 것으로 알려져 왔다(e.g., Vallage et al., 2015). 또한 이러한 양상이 단층의 최종변위(total displacement)가 작을수록 비교적 잘 관찰되고 신기 퇴적층의 두께 등과 같은 지표지질의 영향을 받는 것으로 보고된 바 있다(Dolan and Haravitch, 2014; Zinke et al., 2014). 따라서 최상부 지각에서 지진동시성 변위가 급격하게 감소하는 경향(i.e., shallow slip deficit)은 단층의 진화단계 또는 지표지질과 관련해 단지 일부 사례에서 관찰된 것으로, 지표면에서의 지형학적 변위특성에 기초해 심부 지진파열의 특성을 분석(예를 들면 지진과 관련된 응력에너지를 산정하고 이를 통해 지진재발 특성을 추론하는 것)한다는 가정은 타당성이 높다고 할 수 있다.
3. 연구사 및 최신 연구동향
지진지표파열이 처음 연구논문으로 보고된 것은 1906년 미국의 San Andreas 단층에서 발생한 San Francisco 지진으로 단층을 가로질러 설치된 울타리와 도로, 줄지어 심어진 나무 등이 우수향 감각으로 최대 약 6 m 변위되었다(그림 3a, 3b). 이 사건으로 지진의 기작, 즉 지진과 단층의 상관성을 설명하는 ‘탄성반발이론(elastic-rebound theory)’이 제안되었다(Reid, 1910). 이후 지표파열을 따른 변위분포에 대한 체계적인 분석이 미국 San Andreas 단층의 중남부와 Wasatch 단층을 중심으로 시도되었다(Schwartz and Coppersmith, 1984; Sieh and Jahns, 1984; 그림 3c). 그 결과 단층의 특정지점에서는 개별적인 지진에 의해 거의 동일한 크기의 변위가 규칙적으로 발생한다는 주장이 제안되었으며, 이에 기초하여 제안된 ‘특성지진모델(characteristic earthquake model)’ 및 ‘균일변위모델(uniform-slip model)’은 현재까지도 주요 활성단층에 대한 지진재해평가의 핵심이론으로 인정받고 있다(그림 3d). 한편 이때까지만 해도 비교적 제한된 연구결과가 도출될 수밖에 없었는데, 이는 대부분의 연구가 저해상도 항공사진을 이용한 지형분석 및 야외지질조사에 의존했기 때문이다.

(a) A regional context for the San Andreas Fault (SAF), Walker Lane Belt (WLB), Eastern California shear zone (ECSZ) with respect to several historical ruptures in California (CA) (e.g., the 1857 Fort Tejon, 1872 Owen Valley, 1906 San Francisco, 1992 Landers, 1999 Hector Mine, 2010 El-Mayor Cucapah earthquakes) and the Central Nevada Seismic Belt (CNSB) of Nevada (e.g., 1915 Pleasant Valley, 1932 Cedar Mountain earthquakes, and 1954 Rainbow Mountain - Fairview Peak - Dixie Valley earthquake sequence) (from Haddon et al., 2016). (b) Slip distributions of the 1906 MW 7.8 San Francisco earthquake surface rupture based on field measurements, analysis of geodetic networks, and inversion of teleseismic data (from Zoback, 2006). (c) Prior reconstruction of surface-rupture history along the south-central SAF that is involving the 1857 Fort Tejon earthquake surface rupture (from Sieh and Jahns, 1984). (c) Schematic representation of ‘Characteristic Earthquake Model’ and ‘Uniform-Slip Model’ (from Schwartz and Coppersmith., 1984). See text for details.
1980년대 후반 이후부터 인공위성을 비롯한 원격 탐사기술의 발전으로 점차 정밀한 지표관측이 가능해졌고, 이에 따라 양질의 지표파열 자료가 수집되었다. 이는 다시 컴퓨터 모델링 연구의 기초자료로 활용되었고, 결과적으로 해당 연구분야의 큰 발전을 가져왔다. 가장 대표적인 예로 1992년 미국의 Eastern California 전단대에서 발생한 Landers 지진(MW 7.3) 및 이로 인한 약 85 km 길이의 지표파열을 들 수 있다(e.g., Sieh et al., 1993). 이 지표파열은 InSAR기법을 이용해 변위분포가 조사되었고(Massonnet et al., 1993), 지진 전후에 촬영된 고해상도 위성영상은 계속해서 발전하고 있는 이미지상관법(optical image correlation)에 기초해 현재까지도 정밀하게 분석되고 있다(Milliner et al., 2016). 당시 야외지질조사 및 지진파 분석을 통해 이 지표파열이 여러 단층분절의 연속적인 파열에 기인한 결과로 보고되면서(Sieh et al., 1993), 다양한 방법에 의해 획득된 기초자료들은 단층의 분절시스템을 따른 지진파열의 동역학적 특성에 대한 모델링 연구에 활용되었다(Wald and Heaton, 1994; Peyrat et al., 2001). 다만 원격탐사기법을 이용한 지표관측의 경우, 관련기술이 개발된 이후의 지진지표파열에 대한 정보만을 제공하기 때문에 재발주기가 긴 대규모 지진의 재발특성을 파악하는데 한계가 있다고 할 수 있다.
대규모 고지진의 재발특성에 관한 연구는 2000년 대 초반에 민간과학자들이 1 m 이하의 고해상도 위성영상(e.g., Quickbird, WorldView, Pleiades)을 사용할 수 있게 되면서 한 단계 발전한 것으로 판단된다(Zielke et al., 2015 and references therein). 고해상도 영상을 통해 획득된 최신의 정밀 지형정보를 통해 지진지표파열의 위치나 크기에 상관없이 전 세계 도처에 분포하고 있는 대다수의 활성단층에 대한 조사가 가능해졌고, 또한 저해상도 항공사진을 이용해 이미 조사된 바 있는 단층에 대한 재분석이 시도되었다. 이로써 주향이동단층에서의 대규모 지진과 관련된 여러 지표파열에 대한 체계적인 분석결과가 도출되기 시작하였다(e.g., Wesnousky, 2006; Klinger, 2010). 최근에는 수목을 비롯한 지표상의 장애물을 제거할 수 있는 원격탐사기법인 LiDAR (Light Detection and Ranging) 기법이 적용되면서 보다 정밀한 지형분석이 가능해졌으며(Glennie et al., 2013 and references therein; Kim et al., 2014; 그림 4), 이 방법은 활성단층 연구의 획기적인 발전을 주도한 것으로 평가된다. 가장 대표적인 예로 앞서 언급한 San Andreas 단층의 중남부에 속하는 Carrizo 분절을 들 수 있는데(그림 3c), 최근 LiDAR기법을 이용한 지형분석결과 이 분절에서 최후기에 발생한 1857년 Fort Tejon 지진에 수반된 지표파열의 평균변위가 5.3 +/- 1.4 m로 확인된 바 있다(Zielke et al., 2010, 2012; 그림 4). 이 결과는 8 - 10 m의 평균변위를 제안한 이전의 연구결과(Sieh and Jahns, 1984; 그림 3c)와 큰 차이를 보이는 것으로, 해당 단층분절에 대해 지난 30년 간 적용되어 온 지진규모 및 지진재해 특성이 새롭게 평가되는 계기가 되었다. 광역적인 범위의 지표관측기술의 발전과 더불어 특정 지점에서의 사진기반 3차원 지형정보 획득기술이 발전하면서 활성단층 연구에 큰 도움을 주고 있다(e.g., Westoby et al., 2012; Fonstad et al., 2013). 최근 초경량 무인비행장치(i.e., drone)를 이용해 일부 단층지점 및 구간에 대한 정밀 지형자료가 수집 및 분석되고 있는데, 이는 조사의 비용적인 측면에서 항공 또는 위성영상을 대체할 수 있는 매우 유용한 연구방법으로 평가된다.

Examples of the LiDAR-based offset measurement procedure from right-laterally displaced channels by the 1857 Fort Tejon earthquake surface rupture on the Carrizo Plain (from Zielke et al., 2010). (a) Hillshade map of two channels. (b) An estimation of displacement using two topographic profiles along solid lines, which are projected onto the fault plane based on channel obliquity, in (a). (c) 1/Σ(Δelevation) is a measure of goodness of fit, calculated for each back-slip increment. (d - g) Current and back-slipped hillshade plot of two channels that were offset during the 1857 earthquake.
최근 획득되고 있는 위성영상의 지면해상도(ground resolution)가 약 0.3 m 임을 고려할 때, 이를 이용해 적어도 0.3 m 이상의 변위는 측정할 수 있으며, 각각의 변위자료가 가지는 최소한의 불확실도 또한 이 범위에 속할 것으로 보인다. 비록 고해상도 영상획득 및 컴퓨터 기술의 발전을 바탕으로 실내 지형분석을 통해 비교적 쉽게 변위자료를 수집할 수 있게 되었으나(e.g., LaDiCaoz; Zielke and Arrowsmith, 2012), 여전히 야외지질조사를 통한 지형분석 및 이들 자료와 실내분석 자료와의 비교/검토가 매우 중요하다고 판단된다(Haddon et al., 2016). 한편 단층의 활동연대와 재발주기를 파악하기 위해서는 트렌치를 통한 지질단면 해석과 절대연대측정 등이 동시에 수반되어야 한다. 그러나 이에 대한 내용은 이 논문의 주제에서 벗어난 부분이라 이곳에서는 다루지 않기로 한다.
4. 주요 연구동향
4.1 지진 동시성 지표파열의 기하 및 변위분포 특성
최근 정밀 조사된 대부분의 주향이동성 지표파열은 단층을 따른 단순한 일직선적 형태가 아닌 매우 복잡한 형태를 보인다(Haeussler et al., 2004; Klinger et al., 2005; Xu et al., 2006; Choi et al., 2012; Teran et al., 2015). 대표적인 예로 1) 주단층을 따른 지표파열의 안행상 배열(en-echelon array), 주향 변화, 계단식 배열, 2) 가지단층(branch fault)을 따른 지표파열, 3) 단층 주변 지역에서의 지표균열과 같은 2차적인 지표변형(i.e., off-fault damage or deformation) 등이 있다. 지표파열의 기하학적 복잡성은 수 m에서부터 수십 km의 길이에 달하는 거의 모든 축척에서 관찰되는데, 일반적으로 주단층을 따른 지표파열의 변위분포와 밀접한 공간적 상관성을 보인다(Kim and Sanderson, 2004; Klinger et al., 2005; Choi et al., 2012; Vallage et al., 2015). 즉 주어진 지표파열을 따라 비교적 복잡한 기하를 보이는 지점에서 국지적인 변위의 감소가 발생하는 것이다. Milliner et al. (2016)은 미국의 Eastern California 전단대에서 발생한 1992년 Landers 지진(MW 7.3)과 1999년 Hector Mine 지진(MW 7.1)에 수반된 지표파열 및 지진동시성 변위분포를 분석하였고(see locations in Figure 3a), 이를 통해 국지적으로 변위가 줄어드는 단층구간이 2 km 이상인 경우에는 변위의 감소가 주로 단층의 1차적인 분절시스템에 기인한 반면 변위감소 구간이 그 이하일 경우에는 각 단층분절 내에 존재하는 보다 작은 규모의 기하특성에 기인한 것임을 보고하였다(그림 5). 또한 약 20 km의 거리를 두고 서로 평행하게 발달하는 이들 두 단층이 동일한 단층시스템에 속한다는 점을 바탕으로, 상대적으로 단층의 최종변위가 크고 지표파열의 형태가 단순한 Hector Mine 지진지표파열을 따라 변위의 변화가 드물게 나타나는 것에 주목하였다. 이는 단층의 진화정도(또는 구조적 성숙도; structural maturity)가 지표파열을 따른 변위의 변화양상(i.e., along-fault slip variation)에 영향을 미칠 수 있다는 주장과 일치한다(Kim and Sanderson, 2004; Manighetti et al., 2007; Perrin et al., 2016).

Correlation of along-fault slip variation to geometric complexity of earthquake surface ruptures (from Milliner et al., 2016). Slip profiles for (a) the 1992 Landers and (b) the 1999 Hector Mine earthquake surface ruptures (see locations in Fig. 3a), where colored lines indicate individual fault slip profiles. Below slip profiles, fault traces were mapped by high-resolution imagery correlation (where colored traces correspond to individual colored slip profiles above) as well as in the field immediately following the earthquakes (red lines). Star locates earthquake epicenter.
지진파열이 진원(earthquake origin)으로부터 시작되어 기존에 존재하고 있는 단층을 따라 전파됨을 고려할 때, 지표파열의 양상(기하학적 복잡성) 및 변위분포의 특성(변위의 집중 또는 분산)은 크게 1) 단층을 둘러싸고 있는 매질, 즉 지표지질의 특성과 2) 단층에 내재된 속성에 기인한 결과라 할 수 있다. 전자의 경우 일반적으로 미고화된 퇴적층 및 영구동토층(또는 빙하)의 존재여부와 두께 변화, 또는 낮은 봉압(confining pressure)에서의 자유표면효과(free surface effect)에 의해 영향을 받는다(e.g., Sibson, 2003; Vallage et al., 2015). 한편 지표파열 형태에 영향을 미칠 수 있는 단층요소로는 단층의 종류, 주로 기반암의 종류에 의해 달라지는 단층암의 내부특성, 응력의 불균형을 유발시키는 분절시스템과 가지단층, 그리고 단층손상대(fault damage zone) 등이 있다. 이러한 단층요소들은 3차원 지질구조인 단층대 내부구조(fault zone architecture)와 관련되어 있기 때문에, 지표자료만을 통해 각 단층요소와 지표파열 형태의 상관성을 파악하는 것은 다소 제한적일 수 있다. 이러한 한계점은 단층의 세부적인 속성을 반영한 컴퓨터 모델링 연구를 통해 보완되고 있다(Harris and Day, 1993, 1999; Poliakov et al., 2002; Kame et al., 2003; Duan and Oglesby, 2006; Oglesby and Mai, 2012).
4.2 지진원 규모의 단층 분절시스템
단층의 분절시스템은 지진파열의 특성을 이해하기 위한 가장 핵심적인 단층요소 중 하나이다. 다만 대규모 지진으로 인한 지진재해의 평가측면에서 주어진 활성단층에 대한 최대규모(또는 지진원 규모)의 단층 분절화(seismogenic-scale fault segmentation)를 규명하는 것이 중요하다(Klinger, 2010). 즉 거의 모든 규모에서 관찰되는 단층의 구조적 분절(structural segment) 중에서 지진파열의 거동에 영향을 미칠 수 있는 지진학적 분절(seismic segment)을 구분할 필요가 있다. 이는 주로 지진원 규모의 분절경계부가 개별적인 대규모 지진파열의 개시, 전파 및 확장, 종결 등에 큰 영향을 미치며, 대개 이러한 경향이 여러 번의 지진이 반복되는 동안 지속되기 때문이다(Segall and Pollard, 1980; King and Nabelek, 1985; Klinger, 2010). 따라서 이 논문에서도 주로 단층분절의 최대규모와 관련된 지진파열의 거동특성에 대해 심도 있게 다루었으며, 여기서 ‘분절경계부(inter-segment zone)’라는 용어는 지진원 규모에 해당하는 두 단층분절 사이의 경계부를 의미한다.
지표파열의 분절시스템은 주로 1) 단층의 주향변화와 관련된 단층굴곡(fault bend), 2) 서로 평행한 두 단층분절의 계단식 배열(fault jog): 두 단층분절의 분리 내지 연결 여부에 따라 계단식 분절(fault step) 또는 연속굴곡(fault double-bends)으로 구분됨, 3) 가지단층의 분기점(branch point), 4) 다른 단층과의 교차점(intersection point), 또는 이들이 결합된 형태 등을 기준으로 구분될 수 있다. 일반적으로 분절경계부에서는 단층의 운동감각(slip sense)을 비롯한 주향의 변화방향, 이웃한 단층분절의 배열방향, 주단층과 가지단층의 각관계 등과 같은 단층 기하특성에 따라 국지적인 인장 내지 압축 변형구조(e.g., pull-apart basin, pressure ridge)가 발달한다. 앞서 간략히 언급한 바와 같이, 지진원 규모의 단층 분절시스템은 지표파열을 따른 지진동시성 변위분포의 장파장 변동(long-wavelength variation)과 관련되며, 대표적인 예를 몇 개 들면 1987년 MW 6.6의 미국 Superstition Hills 지진(Sharp et al., 1989), 1997년 MW 7.2의 이란 Zirkuh 지진(Berberian et al., 1999), 1999년 MW ≥ 7.2의 터키 Izmit - Duzce 연쇄지진(Lettis et al., 2002; Duman et al., 2005), 1957년 MW 8.1의 몽골 Gobi-Altay 지진(Choi et al., 2012) 등이 있다(그림 6a - c). 판 내부 주향이동단층에 속하는 각 단층분절의 최대지표길이는 취성변형작용이 발생하는 상부지각의 두께와 유사하며, 지진원 깊이에서도 대개 25 km를 초과하지 않는 것으로 보고된 바 있다(Klinger, 2010; 그림 6d, 6e). 단층의 분절화는 역단층이나 정단층에 비해 주향이동단층에서 더욱 뚜렷하게 관찰되는데, 이는 지진파열의 전파 및 확장이 변위와 평행한 수평방향으로 우세하게 진행되고 이로 인해 이웃한 단층분절로의 응력에너지 전달이 비교적 용이하기 때문이다(Shaw and Scholz, 2001).

Segment geometry and slip distribution along surface ruptures associated with (a) the 1987 MW 6.6 Superstition Hills earthquakes (modified from Sharp et al., 1989), (b) the 1997 MW 7.2 Zirkuh earthquake (modified from Berberian et al., 1999), and (c) the 1957 MW 8.1 Gobi-Altay earthquake (modified from Choi et al., 2012). Star locates earthquake epicenter. (d) The inverse of the slope of the best fit line between the number of segments and the total length of a rupture gives the average length of segment for the data set (from Klinger, 2010; K: Korizan, SH: Superstition Hills, HM: Hector Mine, La: Landers, OV: Owen Valley, Z: Zirkuh, Lu: Luzon, Ko: Kokoxili, GA: Gobi Altay, H: Haiyuan earthquakes). (e) Maximum horizontal extent of slip‐patches measured from slip inversion maps for 18 strike-slip earthquake events (from Klinger, 2010; 1: Gifunken Chubu, 2: Izu Hanto Oki, 3: Oitaken Chubu, 4: Imperial Fault, 5: Izu Hanto Toho, 6: Nagano Ken Seibu, 7: Elmore ranch, 8: Superstition Hills, 9: Joshoa Tree, 10: Landers, 11: Kobe, 12: Antarctica, 13: Duzce, 14: Hector Mine, 15: Izmit, 16: Tottori, 17: Denali, 18: Kokoxili earthquakes).
단층의 계단식 분절경계부는 지진파열의 전파 및 확장을 방해하는 ‘장벽’역할을 하는 것으로 알려져 있다(earthquake barrier model; Aki, 1979; Scholz, 2002; Jin and Kim, 2010; Choi et al., 2012). 즉 하나의 단층분절에서 시작된 지진파열이 분절경계부(i.e., barrier)를 통과함으로써 이웃한 단층분절로 확장된다는 개념으로, 분절경계부에서의 변위감소 및 이와 관련된 2차적인 지표변형(e.g., secondary surface rupture, off-fault ground cracks)은 해당지점에서 지진파열의 전파가 원활하지 못했다는 의미로 해석될 수 있다. 또한 이 이론적 모델은 비교적 많은 지진파열이 분절경계부에서 종결되는 현상을 뒷받침한다(Sibson, 1985; Barka and Kadinsky-Cade, 1988; Knuepfer, 1989). Wesnousky (2006)는 각각 약 10 - 420 km의 길이를 보이는 총 22개의 주향이동지진 지표파열을 대상으로 지진파열의 종결지점을 분석한 결과, 전체의 2/3에 해당하는 종결지점이 단층의 분절경계부임을 보고하였다(그림 7). 특히 두 단층분절 사이의 거리가 3 - 4 km 이상인 경우 모든 지진파열이 종결된 반면 그 이하인 경우 약 40%의 지진파열이 종결된 점에 주목하였다. 또한 지표파열의 총 길이와 무관하게 비교적 공통된 결과가 도출된 점을 바탕으로, 지진파열의 전파방향을 기준으로 파열전면부(rupture front tip)의 응력변화 크기는 지진파열이 전파 및 확장되어 온 거리에 상관없이 비교적 유사하다고 해석하였다.

Relationship of geometrical discontinuities along fault to the endpoints of historical strike-slip earthquake ruptures (from Wesnousky, 2006). The earthquakes are ordered by increasing rupture length. Symbols along the vertical line represent dimension of discontinuities within and at endpoints of each rupture. See text for details.
단층의 진화 기작에 관한 가장 타당성 있는 이론 중 하나는 이웃하는 두 단층분절의 “연결”이다(e.g., Peacock and Sanderson, 1991; Cowie and Scholz, 1992; Walsh et al., 2003; Kim and Sanderson, 2005). 이는 단층의 구조적 성숙도에 따라 분절경계부의 연결성(connectivity)이 변하는 것을 의미하는 것으로, 결국 단층의 진화는 지진파열의 전파와 확장에 영향을 미칠 수 있다(Manighetti et al., 2005; Soliva et al., 2008). 일반적으로 진화 초기의 분절경계부는 비교적 높은 밀도의 2차적인 지표변형이 발달하는 복잡한 기하의 분산변형(distributed deformation)을 보이는 반면 단층이 진화할수록 두 단층분절의 연결성이 양호해짐에 따라 비교적 단순한 기하의 집중변형(localized deformation)을 보인다. 전자의 경우 가장 대표적인 예로써, 단층의 계단식 분절에 의해 주단층을 따른 변위는 감소하고 국지적인 인장 또는 압축변형과 관련된 경사이동 단층운동이 발달한다(Choi et al., 2012). 후자의 경우에는 대표적인 예로 단층의 연속굴곡, 즉 두 단층분절을 잇는 연결단층(connecting fault)이 발달한 형태를 들 수 있는데, 이 때 변위는 주단층을 따라 집중되는 경향이 있다. 이러한 차이점은 분절경계부에서의 서로 다른 수평/수직 변위 간 보상관계(i.e., slip compensation)로 설명된 바 있는데(Choi et al., 2012; 그림 8a), 분절경계부가 구조적으로 진화함에 따라 주변위성분인 수평변위로부터 보조변위성분인 수직변위로의 변환이 점차 줄어든다는 개념이다. 한편 각각의 분절경계부에 작용하는 변형의 종류(인장/압축) 및 이와 관련된 응력의 불균형에 의해 분절경계부의 진화 특성이 상이하고, 이에 따라 지진파열에 미치는 영향이 달라질 수 있다는 점을 이해하는 것이 중요하다(Duan and Oglesby, 2006; Lozos et al., 2011).
초기 계단식 분절을 관통하는 후기의 연결단층이 새로운 단층분절을 형성하기도 하는데, 이 때 새로운 단층분절은 이웃하는 두 분절과 다소 상이한 주향을 보이게 된다(그림 8b). 이러한 진화양상은 구조적 성숙도가 비교적 큰 주향이동단층의 지진원 규모 분절시스템이 주로 약 ~5° 내외의 반복적인 단층굴곡에 의해 구분된다는 주장과 유사하다(Klinger, 2010). 주어진 지구조적 응력장 하에서 기존의 단층이 완전한 주향이동(pure strike-slip)을 보인다고 가정할 때, 다소 변화된 주향을 보이는 새로운 단층분절은 사교이동(oblique slip) 또는 변위분할(slip partitioning)의 특성을 보일 수 있다(King et al., 2005; Klinger et al., 2005). 결과적으로 단층의 분절시스템 및 진화특성은 지진파열의 전파와 종결을 제어함으로써 주어진 단층에서의 지진거동특성을 좌우하는 가장 큰 요소 중 하나임을 알 수 있다. 하나의 단층 분절경계부가 여러 번의 지진에 걸쳐 지진파열에 어떠한 영향을 미쳤는지에 대한 심도 있는 탐구를 위해서는 해당 분절경계부에 이웃한 두 단층분절에 대한 고지진학적 연구가 요구된다(e.g., Elliott et al., 2015).

(a) Compensative relations between horizontal and vertical slip components at inter-segment zones depending on structural maturity of fault linkage zone (from Choi et al., 2012). (b) Example of fault geometry evolving through time along the Haiyuan fault, China (from Klinger, 2010). The bounds of the two segments that connect through the pull‐apart are indicated by the vertical black lines. However, the 1920 MS 8 earthquake rupture went straight across the basin, suggesting that the segment geometry is evolving toward a new configuration, indicated by the dashed vertical lines, where fault bends would be moved both eastward and westward.
4.3 가지단층의 의의
최근 가지단층과 관련한 단층시스템 또한 그 중요성이 부각되고 있는데, 이는 가지단층이 주단층을 따른 지진파열 전파의 ‘경로 전환(path diversion)’을 야기함으로써 대규모 지진의 재발특성을 제어할 수 있기 때문이다(Klinger et al., 2006, 2016; Schwartz et al., 2012). 즉 지진파열이 주단층을 따라 가지단층의 분기점에 도달할 경우, 1) 계속적인 주단층을 따른 전파, 2) 가지단층을 따른 전파, 3) 주단층 및 가지 단층을 따른 전파, 4) 지진파열의 종결을 유발 등 지진파열의 전파에 큰 변화가 발생할 수 있다. 지진파열의 경로를 제어하는 요인은 지구조적 응력장, 가지단층의 분기각(branching angle) 및 길이, 분기점에 도달할 당시의 파열 속도(rupture velocity) 등이 있으며, 이 요인들은 주로 동역학적 시뮬레이션 연구를 통해 밝혀졌다(Poliakov et al., 2002; Kame et al., 2003; Bhat et al., 2007).
가지단층이 주단층의 지진파열에 영향을 미친 가장 대표적인 예로 1905년 몽골에서 발생한 MW 8.3의 Bulnay지진을 들 수 있다(그림 9). 이 지진으로 주단층인 Bulnay단층과 수십 km의 길이를 보이는 두 가지 단층(Teregtyin and Dungen faults)을 따라 총 ~526 km에 달하는 지표파열이 발생하였다(Baljinnyam et al., 1993; Schlupp and Cisternas, 2007). Choi et al. (2015c)은 최근 획득된 고해상도 Pleiades 위성영상 및 야외지질조사를 바탕으로 이 지표파열의 기하학적 특성과 변위분포를 분석하였고, 이를 통해 주단층과 가지단층 시스템에서의 지진파열 전파과정에 주목하였다. 특히 동-서 방향의 주단층을 따른 국지적인 변위의 감소 및 2차적인 지표변형이 1905년 지진으로 인해 지진파열이 일어나지 않은 일부 가지단층의 분기점에서 관찰됨을 확인하였다(그림 9a - 9c). 또한 이러한 경향이 주단층의 인장부(extensional quadrant)에 속하는 북동측 단층지괴 내 동북동-서남서 방향의 가지단층에서 비대칭적으로 집중됨을 확인하였다. 이를 통해 주단층을 따라 동쪽으로 전파하던 지진파열이 주어진 지구조적 응력장 하에서 비교적 단층운동을 일으키기 쉬운 일부 가지단층으로의 ‘경로전환’을 시도하였음을 추론하였다(그림 9d). 또한 주단층을 따른 지표파열의 동쪽 말단부에서 동북동-서남서 방향의 여러 가지단층을 따라 지표파열이 관찰되었는데, 주향이동단층의 대표적인 말단부 손상대(tip damage zone) 구조에 속하는 말꼬리구조(horsetail structure)와 유사하다는 점과 이 지점에서부터 주단층을 따른 변위가 서서히 감소한다는 점을 바탕으로 해당 가지단층의 분기점이 지진파열의 종결을 유도한 것으로 해석하였다(Kim and Sanderson, 2008).

(a) Map of surface ruptures associated with the 1905 M ≥ 8 Tsetserleg - Bulnay earthquake sequence in Mongolia. Stars indicate each earthquake epicenter. (b) A line graph displaying along-fault slip variations, including Max. and Min. values, along the main Bulnay fault. Segmentation of the Bulnay fault can be defined by mainly geometric complexity: the changes of fault azimuth and branch points (junction), and wavy slip profile. (c) An example of detailed mapping for off-fault damages around the junction area between main and branch faults. (d) Effects of major branch faults on propagation of the 1905 Bulnay earthquake rupture. Highly damaged zones (greyish zones) near junction points of the branch faults are located mainly on extensional quadrants. All figures were adapted from Choi et al. (2015c).
다른 사례연구로는 2001년 중국의 Kunlun 단층에서 발생한 MW ~7.8의 Kokoxili 지진에 수반된 지표파열을 들 수 있다(그림 10). 이 지진파열은 1) 소규모 구조분지가 발달된 분절경계부에서 시작되어, 2) 주단층의 분절 중 하나인 약 270 km 길이의 Kusai 분절을 따라 주로 동쪽을 향해 초전단(supershear) 속도로 전파되었으며, 3) 가지단층인 Kunlun Pass 단층을 따라 70 km 길이의 추가적인 지표파열을 일으켰다(Klinger et al., 2005; Vallee et al., 2008; 그림 10a). Klinger et al. (2006)은 Kunlun Pass 단층의 분기점에 도달하기 전까지 주단층을 따른 변위분포가 비대칭적이라는 점과 Kunlun Pass 지표파열을 따라 보조변위성분인 역단층성 수직변위가 우세한 점 등을 바탕으로, 이 지진파열의 종결은 주단층으로부터 Kunlun Pass 단층으로의 지진파열 ‘경로전환’을 통해 유도된 것으로 해석하였다(그림 10). 또한 주단층에 속하는 Kusai 분절과 이에 동쪽으로 이웃한 Xidatan 분절의 연결부에서 국지적으로 집중된 지표균열을 통해 초전단 지진파열 과정에서 계속적인 주단층을 따른 지진파열 전파시도가 있었음을 주장하였다. 이러한 해석은 주단층을 따른 지진파열의 속도 및 가지단층의 분기점을 기준으로 서로 다른 3조의 단층분절이 보이는 각관계에 기초한 모델링 연구결과에 의해 뒷받침된 바 있다(Kame et al., 2003). 나아가 주단층의 Xidatan 분절에 대한 지형분석을 통해 주단층에 속하는 다른 단층분절에 비해 반복적인 지진을 지시하는 누적변위가 잘 관찰되지 않는다는 점에 기초해, Kusai 분절과 Xidatan 분절의 경계부가 여러 번의 지진주기에 걸쳐 지진의 종결 또는 새로운 시작을 유발했음을 추론하였다(Klinger et al., 2006; 그림 10b).
이들 사례연구는 대규모 주향이동단층에서의 지진파열 특성이 지진원 규모의 가지단층에 의해 제어됨을 보여주며, 따라서 주단층-가지단층 시스템의 기하학적 특성에 대한 조사 및 이에 기초한 모델링 연구가 필요함을 보여준다(Mignan et al., 2015; Klinger et al., 2016). 한편 지진파열이 주단층인 Denali 단층으로부터 가지단층인 Totschunda 단층으로 전파된 2002년 알래스카 Denali Fault 지진(MW 7.9)의 경우(그림 11), 여전히 지진파열 경로전환의 원인에 대한 논쟁이 이어지고 있다. 이는 주단층-가지단층 시스템과 지구조적 응력장에 기초한 모델링 연구결과가 제안되었음에도 불구하고(Bhat et al., 2004), 고지진학적 조사를 바탕으로 2002년 Denali Fault 지진 이전의 최후기 지진이 Totschunda단층이 아닌 주단층(i.e., Eastern Denali fault)을 따라 발생했다는 연구결과가 도출되었기 때문이다(Schwartz et al., 2012). 후자의 경우 2002년 지진 이전의 최후기 또는 최근 지진에 의한 단층시스템의 전반적인 응력 불균형이 2002년 발생한 지진파열의 전파에 더욱 큰 영향을 미쳤다는 추론이 가능하다. 따라서 가지단층이 대규모 지진의 재발 특성에 미치는 영향에 대한 보다 심도 있는 탐구를 위해서는 수 ~ 수십 km에 달하는 가지단층을 포함한 전체적인 단층시스템에 대한 양질의 고지진학적 자료가 뒷받침되어야 할 것으로 판단된다(Han et al., 2009; Schwartz et al., 2012).

(a) Rupture map and along-fault slip variations of the 2001 MW ~7.8 Kokoxili earthquake surface rupture in China. Star locates earthquake epicenter. The slip is derived from correlation of Spot images averaged over 5 km along rupture. (c) No clear connection between Kusai and Xidatan fault segments. Earthquake rupture propagated preferably along the Kunlun Pass Fault, whose azimuth requires a thrust component to explain the growth of the Buran Budai Shan. A stress shadow due to the 2001 rupture minimizes chances for future earthquake to initiate at the western end of the Xidatan Fault, and favours propagation from the east and ending at the triple junction. All figures were adapted from Klinger et al. (2006).

Map showing earthquake surface rupture and seismicity associated with the 2002 MW 7.9 Denali Fault earthquake, Alaska (from Fuis and Wald, 2003). Earthquake rupture started with thrust slip on the Susitna Glacier fault, and continued eastward along the Denali fault with sub-seismic events. Then, the rupture started to terminate at a branch point with further rupture propagation onto the Totschunda (branch) fault.
4.4 활성단층을 따른 누적변위의 분포와 특성
앞서 살펴본 바와 같이 지표파열을 따른 지진동시성 변위는 비교적 불규칙적인 양상을 보이는데(e.g., Sieh et al., 1993; Haeussler et al., 2004; Klinger et al., 2005; Xu et al., 2006; Quigley et al., 2010), 다수의 연구결과에서 공통적으로 확인되는 일반적인 사항은 다음과 같이 요약될 수 있다. 1) LiDAR기법 등과 같은 최신 연구기술을 적용한다고 하더라도 지형복원 과정을 방해하는 여러 요인에 의해 변위자료는 어느 정도의 불확실성(평균변위의 크기에 따라 약 11 - 17%의 표준편차)을 포함하고(Gold et al., 2013), 2) 대개 지표지질의 유동학적 특성으로 인해 동일한 단층분절에 속하는 수십 내지 수백 m 길이의 짧은 구간 내에서도 비교적 큰 변위의 변화폭(최대 약 30%)이 관찰되며(Rockwell and Klinger, 2013), 3) 서로 다른 단층분절의 경계부를 중심으로 비교적 큰 파장(대개 수십 km 규모)의 변위 변화가 관찰된다(Manighetti et al., 2007; Wesnousky, 2008). 이 중에서 세 번째 특성은 개별적인 지진파열과 관련된 응력산정 또는 단층속성이 지진파열의 이력과 진화에 미치는 영향을 파악하기 위해 가장 중요한 사항으로 판단되며, 따라서 주어진 활성단층의 지진원 규모 분절시스템에 기초해 각 단층분절에 대한 평균변위 및 변위의 누적 양상을 파악하는 것이 중요하다(Zielke et al., 2015).
최근 고해상도 영상자료를 바탕으로 활성단층을 따른 누적변위의 분포에 대한 연구결과가 도출되고 있다(e.g., Zielke et al., 2012, 2015). 서로 다른 불확실도와 신뢰도를 보이는 각각의 누적변위자료를 이용해 개별적인 지진과 관련된 지표파열의 평균변위를 산정하고자 누적변위확률밀도(cumulative offset probability density) 방법이 주로 활용되고 있다(McGill and Sieh, 1991; 그림 12). 대표적인 사례 연구로는 미국 San Andreas 단층의 중남부에 속하는 Carrizo 분절(Zielke et al., 2010; 그림 12a), 중국의 Fuyun 단층(Klinger et al., 2011; 그림 12b), 미국 San Jacinto 단층에 속하는 Clark분절(Salisbury et al., 2012), 중국의 Haiyuan 단층(Ren et al., 2015), 미국 Walker Lane Belt의 Owen Valley 단층(Haddon et al., 2016) 등이 있다. 단층을 따른 변위의 누적과 관련해 가장 주목할 만한 연구결과 중 하나는, 특정 단층분절 또는 구간을 대상으로 개별적인 지진에 수반된 지표변위의 크기가 거의 일정하다는 것이다(그림 12). 이는 앞서 소개된 지진재발에 관한 ‘균일변위모델’ 또는 ‘특성지진모델’을 뒷받침하는 연구결과로, 전체 단층구간에 비해 각각의 단층분절만을 고려할 때 더욱 명확해지는 경향이 있다. 한편 지진원 규모의 서로 다른 단층분절이 가지는 가장 큰 의미 중 하나는 서로 이웃해 위치한다고 하더라도 개별적인 지진으로 인한 동시적인 지진파열이 발생하지 않을 수 있다는 점이다. 결과적으로 단층의 분절화를 보다 명확하게 규명하고 각 단층분절에 대한 충분한 고지진학적 자료가 뒷받침될 필요가 있다(e.g., Field et al., 2014, Ren et al., 2015).

Along-fault plot of offset PDFs (probability density functions) from selected sites; a) Carrizo Plain and b) Fuyun Fault (from Zielke et al., 2015). PDF area is scaled with quality rating. Individual offset PDFs are summed to generate a COPD (cumulative offset probability density) plot. Clear and well-separated COPD peaks are indicative of surface slip-accumulation pattern. The results show a quasi-periodic repetition of geomorphically recorded offset.
일반적으로 여러 번의 지진주기 동안 유사한 크기의 변위가 규칙적으로 누적되는 것에 비해 층서학적 변형기록에 기초한 고지진학적 자료로 산정된 지진의 재발주기는 비교적 불규칙적이다(e.g., Field et al., 2014; Zielke et al., 2015). 이러한 차이점은 1 m 이하의 작은 지형학적 변위, 즉 비교적 작은 규모의 지진(MW 5.5 - 6.5)에 수반된 지표파열에 의해 발생할 수 있는데, 변위가 작거나 거의 없는 지표균열 형태의 지표파열은 지형학적 변위보다는 지표지질의 층서학적 변위로 기록 및 보존될 가능성이 높기 때문이다(Zielke et al., 2015). 이러한 가능성은 지표지질 조사에 기초한 누적변위량의 규칙성 분석결과, 지형학적 변위를 이용한 분석결과에 비해 더욱 불규칙적이며 오히려 지진의 재발주기가 보이는 불규칙성과 가깝다는 연구결과에 의해 뒷받침된다(Hecker et al., 2013). 이와 유사하게 개별적인 지진지표파열의 종결부에 해당하는 단층분절에 대한 정밀 변위분석이 요구되는데, 이는 다른 단층분절에 비해 변위가 서서히 감소하고 그 평균값이 매우 작은 경향이 있기 때문이다(Ward, 1997).
5. 토 의
5.1 단층손상대와 지진의 상관성
일반적으로 지진원 규모의 큰 단층은 매우 복잡한 내부구조로 구성되는데, 대개 단층의 중심부로써 대부분의 변위가 흡수되는 단층핵(fault core)과 이를 둘러싸고 있는 단층손상대, 즉 단층운동에 의해 변형된 모암으로 구분된다(Chester et al., 1993; Scholz, 2002). 단층핵은 비교적 폭이 얇은 단층비지(fault gouge)나 단층각력암(fault breccia) 등의 단층암으로 구성되는 반면 보다 넓은 폭으로 발달하는 단층손상대는 소규모 단층이나 단열과 같은 2차적인 변형구조로 구성된다. 단층대 내부구조의 특성은 단층의 마찰특성(frictional property)과 미끌림거동(slip behaviors), 즉 지진성 단층운동(i.e., unstable earthquake slip)과 비지진성 단층운동(i.e., stable creep)에 영향을 미친다(Faulkner et al., 2003, 2008). 특히 단층손상대의 경우 여러 번의 반복적인 지진에 의해 변형이 누적된 결과물로서 단층핵을 둘러싼 매질의 탄성강도를 변화시키고 새로운 지진의 발생 시 변형특성(주로 변형의 집중과 분산)에 관여한다(e.g., Sibson, 2003; Thomas et al., 2014). 따라서 단층손상대는 단층에 내재되어 있는 속성과 관련된 지진발생특성을 이해하기 위한 중요한 단서 중 하나이다.
3차원 단층손상대의 개념 및 손상대 구조(damage structure)의 진화특성은 주로 노두규모의 단층에 대한 정밀조사를 통해 정립되었으며(Kim et al., 2003, 2004), 단층 분절시스템을 기준으로 단층면에 대한 손상대의 발달위치 및 관찰대상의 위치에 따라 크게 3종류의 손상대(i.e., along-fault, around-tip, and crossfault damage zones)로 분류할 수 있다(Kim et al., 2004; Choi et al., 2016; 그림 13). 일부 사례연구에서 살펴본 바와 같이, 최근 단층손상대의 개념을 이용해 지진파열의 특성을 이해하고자 하는 연구가 시도되고 있다(e.g., Manighetti et al., 2005; Kim and Sanderson, 2008; Jin and Kim, 2010; Choi et al., 2012). 즉 지진지표파열을 따라 관찰되는 분산변형이 주로 분절경계부 내지 종결부에 집중된다는 사실을 바탕으로, ‘단층손상대’의 기작 및 진화특성을 이용하여 응력의 전달 및 해소를 설명하는 것이다. 가장 대표적인 예로 주향이동단층을 따른 분절경계부에서의 수평/수직변위 보상모델로, 이는 단층의 연결부 손상대(linking damage zones)의 진화모델에 기초해 제안된 것으로 두 단층분절 간 응력의 전달과 관련된다(그림 8a).

A 3-D schematic representation of fault damage zones around a segmented left-lateral fault (from Choi et al., 2016). Fault damage zones can be classified into along-fault, around-tip, and cross-fault damage zones based on different exposures, concerns, and descriptive concepts. C indicates contractional and D indicates dilational zones.
한편 단층의 말단부 손상대(around-tip damage zone)는 주로 지진파열의 종결과 관련된 잔여응력이나 변위의 해소작용으로 설명된다. Kim and Sanderson (2008)은 지진지표파열 종결부의 양측 단층지괴 중주로 인장력이 작용하는 지괴에 손상대 구조가 밀집되는 비대칭성을 보이고, 지진지표파열의 양쪽 종결부를 비교했을 때에도 비대칭적으로 관찰되는 것에 주목하였다. 주로 진원과 먼 쪽에 위치한 지표파열의 종결부에서 손상대 구조가 집중된다는 점을 바탕으로, 전체적인 비대칭적 말단부 손상대가 지진파열의 주전파방향(main propagation direction)에 기인한 결과로 해석하였다. 단층손상대는 3차원의 지질 구조요소로 여진의 분포특성을 통해 설명될 수 있는데(Sibson, 1989; King et al., 1994; Das and Henry, 2003), 즉 여진은 한 번의 지진파열이 종결된 후 잔여응력을 해소시키기 위해 파열된 단층면의 말단부나 연결부에 더 작은 규모의 단층운동이 발생하는 것으로 이해할 수 있다(그림 14). 이러한 관점에 기초해 최근 특정 단층의 일부 단층분절에서 확인되는 지진공백(seismic gap)이 지진원 깊이를 초과한 대규모 지진파열의 여파, 즉 지진파열의 하부 말단부가 연성변형 또는 비지진성 단층운동이 우세한 지진원 깊이 아래에 위치함에 따라 말단부 손상대와 관련된 여진이 발생하지 않는다는 것으로 해석된 바 있다(Jiang and Lapusta, 2016; the Denali earthquake rupture in Figure 14). 반면 지진공백을 보이는 단층분절의 경우, 다른 분절에 비해 가까운 미래에 대규모 지진이 발생할 수 있다는 주장이 제안된 바 있어(e.g., Smith-Konter et al., 2011), 지진공백과 관련된 단층특성을 이해하기 위한 추후 연구가 주목된다.

Observations of large earthquakes and microseismicity patterns on major strike-slip faults (from Jiang and Lapusta, 2016). (a) Spatial relations of the inferred coseismic slip during large earthquakes with hypocenters as stars and microseismicity before (filled circles) and after (unfilled circles), over time periods shown in (b). The large earthquakes are: (i) 2004 MW 6.0 Parkfield, (ii) 1989 MW 6.9 Loma Prieta, and (iii) 2002 MW 7.9 Denali Fault earthquakes. Small earthquakes within 2, 4, and 5 km of the fault for the three cases, respectively, are projected onto the fault plane (except iii) and plotted using a circular crack model with the same seismic moment and 3 MPa stress drop. (b) (Left) Time evolution of the depths of seismicity (gray circles) and (right) the depth distribution of normalized total seismic moment released before, during, and after the mainshock (MS). Here, seismicity and coseismic fault slip inside the regions of the largest slip outlined by the dashed lines in (a) were considered. Seismic moment release before the Denali event is not shown because of the small number of events.
일반적으로 여진의 분포는 지진파열로 인한 국지적인 응력장의 변화와 밀접한 관련이 있는데(King et al., 1994), 응력장의 변화로 인해 주변 단층에서 새로운 지진이 촉발될 수 있다는 점을 이해하는 것이 중요하다. 결국 앞서 언급한 단층 분절시스템에서의 응력 ‘전달’과 유사한 개념으로, 대표적인 예로 지진동시성 지진파열 중에 발생하는 연속적인 지진을 들 수 있다(see sub-events in Figure 11 as an example). 연속적인 지진은 지진파열이 분절경계부를 통과할 때 주로 발생하는데, 이를 통해 단층분절 간응력의 전달 및 지진 촉발을 통해 지진파열이 지속됨을 알 수 있다. 이에 확장된 개념으로 연쇄지진이나 이동성 지진과 같은 여러 번의 지진과 관련된 특징적인 지진발생추이 또한 설명이 가능하다(Stein et al., 1997). 실제 지표파열을 일으킨 다수의 대규모 고지진은 동일한 단층시스템에서 연쇄지진의 일환으로 발생했는데, 대표적인 예를 몇 개 들자면 1905년 Tsetserleg - Bulnay 연쇄지진(약 2주 간격; 그림 9), 1987년 Elmore Ranch - Superstition Hills 연쇄지진(약 12시간 간격; Hudnut et al., 1989), 1992년 Joshua Tree - Landers - Big Bear 연쇄지진(약 2개월과 3시간 간격; Sieh et al., 1993; King et al., 1994), 2002년 Nenana Mountain - Denali Fault 연쇄지진(약 11일 간격; 그림 11), 2010 - 2011년 Canterbury - Christchurch 연쇄지진(약 6개월 간격; Sibson et al., 2011), 2016년 Kumamoto 연쇄지진(약 28시간 간격) 등이 있다.
한편 대규모 지진에 의한 광역적인 응력장의 변화로 인해 그 주변에 발달한 다른 대형 단층시스템에서도 지진이 촉발될 수 있는데, 가장 대표적인 예로 52년 동안(1905 - 1957년) 몽골에서 발생한 총 4회의 대규모 지진(MW ~8)을 들 수 있다. 즉 이들 지진이 약 3,000년의 대규모 지진 재발주기를 보이고, 약 400 km 이상 떨어져 위치하는 서로 다른 단층에서 발생한 점을 감안할 때, 대규모 지진이 단기간에 집중적으로 발생한 것은 서로 간의 역학적 상호작용이 있었음을 시사한다(Chery et al., 2001). 결론적으로 주어진 활성단층에 대한 지진재해를 평가함에 있어 주변의 단층을 포함한 전체적인 단층시스템을 대상으로 한 단층손상대 특성 및 이와 관련된 지진 촉발 기작을 파악하는 것이 중요할 것으로 판단된다.
5.2 국내 활성단층 연구: 양산단층의 분절시스템을 중심으로
우리나라의 활성단층 연구는 1990년대 초기부터 꾸준히 수행되고 있는데, 주로 원자력 발전 산업과 관련된 시설물의 지진학적 안정성을 확보하기 위한 목적으로 실시되었다. 따라서 원자력 발전소가 주로 위치하고 있는 한반도 남동부 지역을 중심으로 진행되었으며, 그 결과 지난 25년간 양산단층, 울산단층 및 일광단층 등을 중심으로 약 60여개의 지점에서 제4기 퇴적층을 변위시킨 단층, 즉 제4기 단층지점이 보고된 바 있다(Kim et al., 2011 and references therein; see locations of the Quaternary fault sites in Figure 15a). 이러한 연구결과가 의미하는 가장 주요한 점 중 하나는, 한반도가 제4기 동안 거의 유사한 지구조환경에 놓여있다는 사실을 고려할 때, 이들 주요 단층들의 전체 또는 일부 단층분절이 ‘활성’일 가능성이 매우 높다는 사실이다. 그럼에도 불구하고 지형분석에 기초한 활성단층의 분절화 및 변위분포 연구는 거의 이루어지고 있지 않은 실정이다.
우리나라의 대표적인 주향이동단층인 양산단층은 전체적으로 북북동-남남서 방향의 단층곡으로 인지되며, 총 길이는 150 - 200 km에 달한다(그림 15). 양산단층에 의해 변위된 백악기 경상분지 내 화강암류의 수평변위를 통해 총 20 - 35 km의 우수향 변위가 추론된 바 있다(Chang et al., 1990; Hwang et al., 2004; 그림 15a). 변형사 연구결과 양산단층은 에오세 이후 주로 우수향 운동을 겪었으며, 좌수향 및 경사이동 단층운동 또한 겪었음을 지시한다(e.g., Chang and Chang, 1998). 비교적 복잡한 구조운동사는 동해의 확장 및 닫힘으로 대표될 수 있는 신생대 이후의 지구조 환경변화 및 이에 따른 지각변형의 산물로 해석된 바 있다(Yoon and Chough, 1995).

(a) Simplified regional geologic map of the Gyeongsang Basin, SE Korea (modified from Chough and Sohn, 2010; Son et al., 2013). Segmentation of the Yangsan Fault proposed by (b) previous studies (Lee and Jin, 1991; Chang and Chang, 2009), and (c) this study. Note that our criteria for fault segmentation are locations of fault bend, jog, and branch points with the Ulsan Fault, and the result indicates that the Yangsan Fault is composed of 9 individual fault segments.
양산단층에 대해 ‘활성단층’으로서의 가능성이 제기된 이후(Lee and Na, 1983), 이를 규명하기 위한 다양한 고지진학적 연구가 수행된 바 있다. Lee and Jin (1991)은 AD 2년부터 1989년까지 양산단층에서 발생한 총 126회의 역사지진 및 14회의 계기지진 자료를 바탕으로 양산단층이 총 3개의 분절(북부, 중부, 남부)로 구성되어 있음을 제안하였다(그림 15b). 이들은 비교적 지진이 드물게 발생하는 지점을 분절 경계부의 기준으로 판단하였다. 이후 Chang and Chang (2009)은 단층의 주향변화, 단층대 폭의 변화, 단층대 내에 발달하는 소규모 단층들의 기하학적 및 운동학적 특성 등에 기초해 포항시와 양산시 사이 80 km 길이의 단층구간을 총 5개의 구역으로 구분하였고, 각 구역의 단층거동 특성에 대해 논하였다(그림 15b). 양산단층의 북부와 남부분절의 일부 지점에서는 제4기 퇴적층을 변위시킨 지표파열에 대한 고지진학적 조사가 수행된 바 있다(Okada et al., 1994; Kyung et al., 1999; Lee, B.J. et al., 1999; Kyung and Chang, 2001; Kyung, 2003, 2010; Kim and Jin, 2006; Lee, J. et al., 2015). 이들 연구결과에 따르면, 양산단층의 북부와 남부는 각각 0.1 mm/yr과 0.02 - 0.07 mm/yr 이하의 제4기 후기 수직 변위률을 보이고, AD 636년(1,314 yr BP) 이후와 홀로세(Holocene) 이전에 각각 최후기 단층운동을 겪었다고 보고되었다(Kyung and Lee, 2006). 다만 양산단층이 제4기 동안 동-서 내지 동북동-서남서 방향의 압축 환경 하에서 주로 우수향 주향이동이 우세한 단층운동을 겪은 것으로 알려져 있음에도 불구하고(Park et al., 2007), 양산단층의 수평 변위률에 대한 정보는 단지 수직 변위률의 수배에 달하는 것으로 추정만 되었을 뿐이다. 최근 양산단층의 자연노두가 비교적 잘 남아있는 일부 지역을 대상으로 연구가 이어져 오고 있으며, 대표적인 연구 사례로는 1) 야외지질조사에 기초한 단층대 내부구조 해석(Choi et al., 2009), 2) 단층비지의 ESR (Electron Spin Resonance) 연대에 기초한 제4기 단층운동의 시공간적 이력 해석(Yang and Lee, 2014), 3) 단층비지의 전단속도변화 실험을 통한 전단특성 해석(Woo et al., 2015; Kim et al., 2016) 등이 있다.
그럼에도 불구하고 양산단층의 기하학적 특성 및 각 분절간 서로 다른 고지진 시기 등을 토대로 한 지진원 규모의 분절시스템이 현재까지 명확하게 규명되지 못한 실정이다. 비록 활성단층정밀지도를 비롯한 보다 많은 자료가 확보되어야 하겠으나, 지금까지 조사된 지진학적, 구조지질학적, 고지진학적 자료 및 위성영상에서 인지되는 단층의 기하학적 특성(i.e., 단층의 굴곡 및 계단식 분절, 가지단층의 분기점)을 바탕으로 양산단층이 크게는 3조의 분절로 구성되고, 작게는 총 9조의 분절로 구성되어 있음을 제안한다(그림 15c). 이들 단층분절은 주로 5 - 15° 내외의 단층 굴곡에 의해 경계지어지며, 각 단층분절의 길이는 약 25 ± 5 km로 지금까지 알려진 경상분지의 지각 두께(26 - 38 km; Chang and Baag, 2005; Yoo et al., 2007; Lee, 2010)를 초과하지 않고 대체적으로 유사하다. 이러한 특성은 비교적 구조적 성숙도가 높은 대형 주향이동단층의 일반적인 분절특성과 일치한다. 따라서 여기서 제안된 양산단층의 분절시스템은 향후 이 단층을 따른 대규모 지진의 특성을 평가하기 위한 기초자료로 활용될 수 있을 것으로 판단된다.
양산단층을 따라 가장 분명하게 관찰되는 분절경계부 중 한 곳은 울산시 울주군 두서면 일대로, 이 지역은 1) 양산단층의 단층곡이 불연속적(또는 계단식 분절)이고, 2) 이웃한 두 단층분절의 주향이 다르고, 3) 지진학적 및 구조지질학적 자료에서 급격한 차이점이 나타난다(Lee and Jin, 1991; Chang and Chang, 2009; 그림 15c). 이 지점은 양산단층 중부와 남부의 경계부로 오랜 기간동안 지진파열의 전파 및 확장을 방해하는 ‘장벽’역할을 했을 것으로 추정된다. 여기서 주목할 만한 사실은 이 분절경계부 주변에서 지난 9.12 경주 연쇄지진 및 이후 한 달간 이어진 약 470여 차례의 여진이 발생했다는 점이다. 따라서 이번 경주 연쇄지진으로 인해 양산단층의 중부 내지 남부분절에서의 지진이 촉발될 가능성이 있다고 할 수 있다. 이들 두 단층분절은 비교적 구조적 성숙도가 높은 것으로 판단되기 때문에 만약 대규모 지진이 발생할 경우 파괴적인 지진재해가 발생할 수 있을 것으로 추정된다.
한편 양산단층의 남동부에 발달하는 북북서-남남동 방향의 울산단층대를 따라 비교적 많은 지점에서 제4기 퇴적층을 변위시킨 지표파열이 보고된 바 있다(Kyung and Lee, 2006; Kim et al., 2011; 그림 15a). Han et al. (2009)은 양산-울산단층계를 울산단층이 양산단층의 중부로 수렴하는 λ-단층 시스템(Du and Aydin, 1995)으로 해석하고, 현생 지구조적 응력장 하에서 양산단층의 북부와 울산단층의 동측지괴에 응력이 주로 집중되고 있다고 주장하였다. 이는 제4기 단층지점 및 근래의 소규모 지진분포와 비교적 일치하는 결과로, 대규모 고지진의 이력 또한 양산-울산 단층시스템의 기하학적 특성과 밀접한 관련이 있음을 시사한다. 따라서 양산단층과 울산단층이 접하는 지역을 중심으로 한 정밀 단층조사를 통해 이들 두 단층의 관계를 명확하게 밝힐 필요가 있으며, 양산단층의 고지진 재발특성을 논함에 있어 지진파열의 경로전환을 유도할 수 있는 ‘가지단층’으로써의 울산단층에 대한 정밀분석과 연구가 요구된다.
결론적으로 양산단층에서의 고지진 이력에 대한 보다 심도 있는 접근을 위해서, 지형학적 변위에 기초한 활성단층 추적이 최우선적으로 요구된다. 따라서 고해상도 위성영상과 LiDAR기법 등의 최신 연구기술 도입이 시급한데, 이는 활성단층의 추적뿐만 아니라 향후 미래지진으로 지표파열이 발생할 경우 지표파열 이전의 지형을 기록한다는 점에서도 매우 중요하다. 또한 우리나라의 경우 수 m의 지형적 변위를 유발하는 대규모 지진에 비해 약 1 m 내외의 작은 변위를 수반하는 중규모의 지진이 상대적으로 빈번하게 발생할 확률이 높기 때문에, 신기 퇴적층과 같은 지표지질의 단면분석을 통해 양질의 고지진 자료가 꾸준히 수집되어야 할 것으로 판단된다.
6. 지진재해 측면에서의 의의 및 결론
일본은 우리나라와는 달리 판의 경계부에 속해 있기 때문에 대규모 지진의 발생 가능성이 높다. 이로 인해 지진 및 지진재해평가에 대한 꾸준한 학술연구가 진행되었고, 그 결과 내진설계를 비롯한 고도의 지진방재시스템을 구축한 것으로 평가받아 왔다. 그럼에도 불구하고 지난 2011년에 발생한 Tohoku 지진(MW 9) 및 이로 인해 유발된 해일로 심각한 인명 및 재산피해가 발생하였으며(Norio et al., 2011), 이어진 원자력 발전소 안전사고 및 방사능 누출은 현재까지도 전 지구적인 피해를 일으키고 있다. 이러한 파괴적인 결과의 가장 주된 요인은 해당 지역에 대한 충분한 고지진학적 연구에도 불구하고 규모 9이상의 초대형 지진을 예상하지 못하고 이에 상응하는 지진방재시스템을 구축하지 못했기 때문이다(Stein and Okal, 2011). 이 지진재해가 발생한 이후, Maercklin et al. (2012)은 Tohoku 지진과 관련된 섭입판의 심부는 각각 규모 8정도의 대규모 지진을 일으킨 적이 있는 분리된 두 개의 분절로 구성된 반면 이 섭입판의 천부는 규모 9에 해당하는 한 번의 지진으로 인해 동시에 단층운동이 발생할 수 있음을 제안하였다. 따라서 이 사건을 통해 배울 수 있는 가장 뚜렷하고 명확한 교훈 중 하나는 지진재해를 평가함에 있어, 단층의 분절화에 기초한 지진파열 시나리오를 평가하되 발생가능한 최대규모의 지진을 추론하여 이에 상응하는 대비를 하는 것이 매우 중요하다는 것이다(Mignan et al., 2015). 실제 우리가 확보할 수 있는 고지진학적 자료는 주어진 활성단층에서 실제 발생한 고지진의 시공간적 이력에 비해 매우 제한적이기 때문에, 일부 단층분절과 관련된 고지진 정보만을 획득하기 쉽다. 이는 결국 고지진의 규모, 즉 앞으로 발생가능한 지진의 최대규모가 저평가될 수 있음에 항상 유의하여야 함을 의미한다.
삼국사기에 따르면 경주지역에서는 지난 779년 발생한 지진으로 인해 주택붕괴는 물론 총 사망자가 100여명에 달하는 인명 피해가 발생하였다. 당시 피해 정도를 바탕으로 추정된 지진의 규모는 최소 6.0에 해당한다. 경주지역을 중심으로 양산단층과 울산단층이 분포하고 이들 단층을 따라 비교적 최근까지 지진지표파열이 발생하였다. 결과적으로 이러한 사실들을 종합해 볼 때, 양산단층 또는 울산단층을 따라 큰 규모의 지진이 재발할 수 있음을 의미한다. 만일 이들 단층에서 규모 6 또는 그 이상의 지진이 다시 발생한다면 779년 경주지진과는 비교할 수 없는 엄청난 피해를 초래할 가능성이 매우 높다. 그 이유는 오늘날 양산단층과 울산단층의 인근에는 인구와 산업시설이 밀집된 부산, 울산, 포항 등의 대도시뿐만 아니라 원자력 산업과 관련된 시설물들이 위치해있기 때문이다. 그러나 우리나라의 경우 9.12 경주연쇄지진 이전까지 활성단층 연구에 대한 국민적 관심이 결여되어 있었고, 이로 인해 관련 분야의 발전도 느리게 진행되어 왔다. 따라서 이를 계기로 양산-울산 단층시스템에 대한 국가적 차원의 활성단층조사가 시급하며, 나아가 한반도 전역에 대한 꾸준한 고지진학적 연구를 수행하여 지진재해를 평가하기 위한 기초자료를 확보할 필요가 있다.
원격탐사기법의 급속한 발전을 바탕으로 선진국에서는 대규모 지진에 수반된 지표파열의 기하학적 특성 및 변위분포에 관한 체계적인 연구가 시작되었고, 또한 추가적인 자료수집이 꾸준히 진행되고 있다. 이로써 단층과 지진파열의 상관성에 대해 보다 많은 접근과 이해가 있었으며, 이는 지진재해평가를 위한 기초자료를 갱신 또는 추가하는 매우 중요한 결과로 이어지고 있다. 지금까지의 연구결과가 공통적으로 지시하는 것 중 가장 의미 있는 사실은 정밀한 활성단층지도제작(active fault mapping)을 통해 활성단층의 분포/기하 및 변위분포와 관련된 자료를 수집하는 것이 매우 중요하다는 것이다. 또한 단층의 분절시스템을 규명하고 각 단층분절에 대한 변위의 누적 및 고지진의 이력을 분석할 필요가 있다. 아직까지 대규모 지진의 발생시기를 정확히 예측하는 것은 현재의 과학기술로 거의 불가능하다. 그러나 지진의 재발특성을 평가하여 대비함으로써 언제 발생할지 모르는 대규모 지진으로부터의 피해를 줄이는 것은 가능하다는 점을 잊지 않는 것이 중요하다.
Acknowledgments
이 논문은 2014-2015년도 (재)기상기술개발원에서 지원하는 지진기술개발사업 “한반도 동남부 지진특성분석과 단층과의 연관성 해석”과제(CATER 2014-8010) 및 2016년도 정부(교육부)의 재원으로 한국연구재단(NRF-2016R1A6A3A03010613)의 지원을 받아 수행된 기초연구사업임.
REFERENCES
-
Aki, K., (1979), Characterization of barriers on an earthquake fault, Journal of Geophysical Research, 84(B11), p6140-6148.
[https://doi.org/10.1029/jb084ib11p06140]
- Baljinnyam, I., Bayasgalan, A., Borisov, B.A., Cisternas, A., Demyanovich, M.G., Ganbaatar, L., Kochetkov, V.M., Kurushin, R.A., Molnar, P., Philip, H., and Vashchilov, Y.Y., (1993), Ruptures of major earthquakes and active deformation in Mongolia and its surroundings, Geological Society of America Memoirs, 181, p43-52.
-
Barbot, S., Fialko, Y., and Sandwell, D., (2008), Effect of a compliant fault zone on the inferred earthquake slip distribution, Journal of Geophysical Research, 113, pB06404.
[https://doi.org/10.1029/2007jb005256]
-
Barka, A.A., and Kadinsky-Cade, K., (1988), Strike-slip fault geometry in Turkey and its influence on earthquake activity, Tectonics, 7(3), p663-684.
[https://doi.org/10.1029/tc007i003p00663]
-
Ben-Zion, Y., (2008), Collective behavior of earthquakes and faults: Continuum-discrete transitions, progressive evolutionary changes, and different dynamic regimes, Reviews of Geophysics, 46(4), pRG4006.
[https://doi.org/10.1029/2008rg000260]
-
Berberian, M., Jackson, J.A., Qorashi, M., Khatib, M.M., Priestley, K., Talebian, M., and Ghafuri-Ashtiani, M., (1999), The 1997 May 10 Zirkuh (Qa’enat) earthquake (Mw 7.2): Faulting along the Sistan suture zone of eastern Iran, Geophysical Journal International, 136(3), p671-694.
[https://doi.org/10.1046/j.1365-246x.1999.00762.x]
-
Bhat, H.S., Dmowska, R., Rice, J.R., and Kame, N., (2004), Dynamic slip transfer from the Denali to Totschunda Faults, Alaska: Testing theory for fault branching, Bulletin of the Seismological Society of America, 94(6B), pS202-S213.
[https://doi.org/10.1785/0120040601]
-
Bhat, H.S., Olives, M., Dmowska, R., and Rice, J.R., (2007), Role of fault branches in earthquake rupture dynamics, Journal of Geophysical Research, 112, pB11309.
[https://doi.org/10.1029/2007jb005027]
- Bonilla, M.G., (1988), Minimum earthquake magnitude associated with coseismic surface faulting, Bulletin of the Association of Engineering Geologists, 25, p17-29.
- Chang, C.J., and Chang, T.W., (1998), Movement history of the Yangsan Fault based on paleostress analysis, The Journal of Engineering Geology, 8(1), p35-49, (in Korean with English abstract).
- Chang, C.J., and Chang, T.W., (2009), Behavioral characteristics of the Yangsan Fault based on geometric analysis of fault slip, The Journal of Engineering Geology, 19(3), p277-285, (in Korean with English abstract).
- Chang, K.-H., Woo, B.-G., Lee, J.-H., Park, S.-O., and Yao, A., (1990), Cretaceous and Early Cenozoic stratigraphy and history of Eastern Kyongsang Basin, S. Korea, Journal of the Geological Society of Korea, 25(5), p471-487.
-
Chang, S.J., and Baag, C.E., (2005), Crustal structure in southern Korea from joint analysis of teleseismic receiver functions and surface-wave dispersion, Bulletin of the Seismological Society of America, 95(4), p1516-1534.
[https://doi.org/10.1785/0120040080]
- Chery, J., Carretier, S., and Ritz, J.-F., (2001), Postseismic stress transfer explains time clustering of large earthquakes in Mongolia, Earth and Planetary Science Letters, 194(1), p277-286.
-
Chester, F.M., Evans, J.P., and Biegel, R.L., (1993), Internal structure and weakening mechanisms of the San-Andreas fault, Journal of Geophyscal Research, 98(B1), p771-786.
[https://doi.org/10.1029/92jb01866]
-
Choi, J.-H., Edwards, P., Ko, K., and Kim, Y.-S., (2016), Definition and classification of fault damage zones: A review and a new methodological approach, Earth-Science Reviews, 152, p70-87.
[https://doi.org/10.1016/j.earscirev.2015.11.006]
- Choi, J.-H., Jin, K., Enkhbayar, D., Davvasambuu, B., Bayasgalan, A., and Kim, Y.-S., (2012), Rupture propagation based on damage patterns, slip distribution, and fault segmentation of the 1957 Mw 8.1 Gobi-Altay earthquake rupture along the Bogd fault, Mongolia, Journal of Geophysical Research, 117, pB12401.
- Choi, J.-H., Kim, Y.-S., and Choi, S.-J., (2015a), Identification of a suspected Quaternary fault in eastern Korea: Proposal for a paleoseismic research procedure for the mapping of active faults in Korea, Journal of Asian Earth Sciences, 113, p897-908.
- Choi, J.-H., Kim, Y.-S., Gwon, S., Edwards, P., Rezaei, S., Kim, T., and Lim, S.-B., (2015b), Characteristics of large-scale fault zone and Quaternary fault movement in Maegok-dong, Ulsan, The Journal of Engineering Geology, 25(4), p485-498, (in Korean with English abstract).
- Choi, J.-H., Klinger, Y., Ferry, M., Ritz, J.-F., Kurtz, R., Rizza, M., Bollinger, L., Davaasambuu, B., Tsend-Ayush, N., Ulziibat, M., Chimed, O., and Demberel, S., (2015c), High-resolution surface-rupture map and slip distribution for the 1905 M8 Tsetserleg-Bulnay, Mongolia, earthquake sequence, 2015 AGU Fall Meeting (Abstract), San Francisco, Dec. 14-18, pT41B-2874.
- Choi, J.-H., Yang, S.-J., and Kim, Y.-S., (2009), Fault zone classification and structural characteristics of the southern Yangsan fault in the Sangcheon-ri area, SE Korea, Journal of the Geological Society of Korea, 45(1), p9-28, (in Korean with English abstract).
-
Choi, S.-J., Jeon, J.S., Choi, J.-H., Kim, B., Ryoo, C.-R., Hong, D.-G., and Chwae, U., (2014), Estimation of possible maximum earthquake magnitudes of Quaternary faults in the southern Korean Peninsula, Quaternary International, 344, p53-63.
[https://doi.org/10.1016/j.quaint.2014.05.052]
-
Chough, S.K., and Sohn, Y.K., (2010), Tectonic and sedimentary evolution of a Cretaceous continental arc-backarc system in the Korean peninsula: New view, Earth-Science Reviews, 101, p225-249.
[https://doi.org/10.1016/j.earscirev.2010.05.004]
-
Cowie, P.A., and Scholz, C.H., (1992), Physical explanation for the displacement-length relationship of faults, using a post-yield fracture mechanics model, Journal of Structural Geology, 14, p1133-1148.
[https://doi.org/10.1016/0191-8141(92)90065-5]
-
Das, S., and Henry, C., (2003), Spatial relation between main earthquake slip and its aftershock distribution, Reviews of Geophysics, 41(3), p1013.
[https://doi.org/10.1029/2002rg000119]
-
Dolan, J.F., and Haravitch, B.D., (2014), How well do surface slip measurements track slip at depth in large strike-slip earthquakes? The importance of fault structural maturity in controlling on-fault slip versus off-fault surface deformation, Earth and Planetary Science Letters, 388, p38-47.
[https://doi.org/10.1016/j.epsl.2013.11.043]
-
Du, Y., and Aydin, A., (1995), Shear fracture patterns and connectivity at geometric complexities along strike-slip faults, Journal of Geophysical Research, 100, p18093-18102.
[https://doi.org/10.1029/95jb01574]
-
Duan, B., and Oglesby, D.D., (2006), Heterogeneous fault stresses from previous earthquakes and the effect on dynamics of parallel strike-slip faults, Journal of Geophysical Research, 111, pB05309.
[https://doi.org/10.1029/2005jb004138]
-
Duman, T.Y., Emre, O., Dogan, A., and Ozalp, S., (2005), Step-over and bend structures along the 1999 Duzce earthquake surface rupture, North Anatolian fault, Turkey, Bulletin of the Seismological Society of America, 95(4), p1250-1262.
[https://doi.org/10.1785/0120040082]
-
Elliott, A.J., Oskin, M.E., Liu-Zeng, J., and Shao, Y., (2015), Rupture termination at restraining bends: The last great earthquake on the Altyn Tagh Fault, Geophysical Research Letters, 42(7), p2164-2170.
[https://doi.org/10.1002/2015gl063107]
-
Faulkner, D.R., Lewis, A.C., and Rutter, E.H., (2003), On the internal structure and mechanics of large strike-slip fault zones: field observations of the Carboneras fault in southeastern Spain, Tectonophysics, 367, p147-156.
[https://doi.org/10.1016/s0040-1951(03)00134-3]
-
Faulkner, D.R., Mitchell, T.M., Rutter, E.H., and Cembrano, J., (2008), On the structure and mechanical properties of large strike-slip faults, Geological Society, London, Special Publications, 299, p139-150.
[https://doi.org/10.1144/sp299.9]
-
Fialko, Y., Sandwell, D., Simons, M., and Rosen, P., (2005), Three-dimensional deformation caused by the Bam, Iran, earthquake and the origin of shallow slip deficit, Nature, 435, p295-299.
[https://doi.org/10.1038/nature03425]
-
Field, E.H., Arrowsmith, R.J., Biasi, G.P., Bird, P., Dawson, T.E., Felzer, K.R., Jackson, D.D., Johnson, K.M., Jordan, T.H., Madden, C., Michael, A.J., Milner, K.R., Page, M.T., Parsons, T., Powers, P.M., Shaw, B.E., Thatcher, W.R., Weldon II, R.J., and Zeng, Y., (2014), Uniform California Earthquake Rupture Forecast, Version 3 (UCERF3)-The Time-Independent Model, Bulletin of the Seismological Society of America, 104(3), p1122-1180.
[https://doi.org/10.1785/0120130164]
-
Fonstad, M.A., Dietrich, J.T., Courville, B.C., Jensen, J.L., and Carbonneau, P.E., (2013), Topographic structure from motion: a new development in photogrammetric measurement, Earth Surface Processes and Landforms, 38, p421-430.
[https://doi.org/10.1002/esp.3366]
- Fuis, G.S., and Wald, L.A., (2003), Rupture in south-central Alaska-The Denali fault earthquake of 2002, U.S. Geological Survey Fact Sheet, p014-03.
-
Glennie, C.L., Carter, W.E., Shrestha, R.L., and Dietrich, W.E., (2013), Geodetic imaging with airborne lidar: the Earth's surface revealed, Reports on Progress in Physics, 76(8), p086801.
[https://doi.org/10.1088/0034-4885/76/8/086801]
-
Gold, P.O., Oskin, M.E., Elliott, A.J., Hinojosa-Corona, A., Taylor, M.H., Kreylos, O., and Cowgill, E., (2013), Coseismic slip variation assessed from terrestrial lidar scans of the El Mayor-Cucupah Surface Rupture, Earth and Planetary Science Letters, 366, p151-162.
[https://doi.org/10.1016/j.epsl.2013.01.040]
- Gutenberg, B., and Richter, C.F., (1954), Seismicity of the Earth and Associated Phenomena, (2nd ed.), Princeton University Press, Princeton, New Jersey.
-
Haddon, E.K., Amos, C.B., Zielke, O., Jayko, A.S., and Burgmann, R., (2016), Surface slip during Large Owens Valley earthquakes, Geochemistry, Geophysics, Geosystems, 17(6), p2239-2269.
[https://doi.org/10.1002/2015gc006033]
-
Haeussler, P.J., Schwartz, D.P., Dawson, T.E., Stenner, H.D., Lienkaemper, J.J., Sherrod, B., Cinti, F.R., Montone, P., Craw, P.A., Crone, A.J., and Personius, S.F., (2004), Surface rupture and slip distribution of the Denali and Totschunda faults in the 3 November 2002 M 7.9 earthquake, Alaska, Bulletin of the Seismological Society of America, 94(6B), pS23-S52.
[https://doi.org/10.1785/0120040626]
- Han, S.-R., Park, J.Y., and Kim, Y.-S., (2009), Evolution modeling of the Yangsan-Ulsan fault system with stress changes, Journal of the Geological Society of Korea, 45(4), p361-377, (in Korean with English abstract).
-
Hanks, T.C., and Kanamori, H., (1979), A moment magnitude scale, Journal of Geophysical Research, 84, p2348-2350.
[https://doi.org/10.1029/jb084ib05p02348]
-
Harris, R., and Day, S., (1993), Dynamics of fault interaction: Parallel strike-slip faults, Journal of Geophysical Research, 98(B3), p4461-4472.
[https://doi.org/10.1029/92jb02272]
-
Harris, R., and Day, S., (1999), Dynamic 3D simulations of earthquakes on En Echelon Faults, Geophysical Research Letters, 26(14), p2089-2092.
[https://doi.org/10.1029/1999gl900377]
-
Hecker, S., Abrahamson, N.A., and Wooddell, K.E., (2013), Variability of displacment at a point: implications for earthquake-size distribution and rupture hazard on faults, Bulletin of the Seismological Society of America, 103(2A), p651-674.
[https://doi.org/10.1785/0120120159]
-
Hudnut, K.W., Seeber, L., and Pacheco, J., (1989), Cross-fault triggering in the November 1987 Superstition Hills eartqhauake sequence, Southern California, Geophysical Research Letters, 16(2), p199-202.
[https://doi.org/10.1029/gl016i002p00199]
- Hwang, B.-H., Lee, J.-D., and Yang, K., (2004), Petrological study of the granitic rocks around the Yangsan Fault: Lateral Displacement of the Yangsan Fault, Journal of the Geological Society of Korea, 40(2), p161-178, (in Korean with English abstract).
-
Im, C.B., Shim, T.M., Choi, H.S., Yang, J.M., and Park, S.J., (2016), Technical status and applications of the probabilistic fault displacement hazard analysis, Journal of the Geological Society of Korea, 52(4), p447-455, (in Korean with English abstract).
[https://doi.org/10.14770/jgsk.2016.52.4.447]
-
Jiang, J., and Lapusta, N., (2016), Deeper penetration of large earthquakes on seismically quiescent faults, Science, 352(6291), p1293-1297.
[https://doi.org/10.1126/science.aaf1496]
-
Jin, K., and Kim, Y.-S., (2010), Review and new interpretation for the propagation characteristics associated with the 1999 Chi-Chi earthquake faulting event, Island Arc, 19(4), p659-675.
[https://doi.org/10.1111/j.1440-1738.2010.00740.x]
-
Kame, N., Rice, J.R., and Dmowska, R., (2003), Effects of pre-stress state and rupture velocity on dynamic fault branching, Journal of Geophysical Research, 108(B5), p2265.
[https://doi.org/10.1029/2002jb002189]
-
Kaneko, Y., and Fialko, Y., (2011), Shallow slip deficit due to large strike-slip earthquakes in dynamic rupture simulations with elasto-plastic off-fault response, Geophysical Journal International, 186, p1389-1403.
[https://doi.org/10.1111/j.1365-246x.2011.05117.x]
-
Kim, C.-M., Han, R., Jeong, G.Y., Jeong, J.O., and Son, M., (2016), Internal structure and materials of the Yangsan fault, Bogyeongsa area, Pohang, South Korea, Geosciences Journal, 20(6), p759-773.
[https://doi.org/10.1007/s12303-016-0019-8]
-
Kim, H.-T., Kim, Y.-S., and We, K.-J., (2014), Basic concepts and geological applications of LiDAR, The Journal of Engineering Geology, 24(1), p123-135, (in Korean with English abstract).
[https://doi.org/10.9720/kseg.2014.1.123]
- Kim, Y.-S., and Jin, K., (2006), Estimated earthquake magnitude from the Yugye Fault displacement on a trench section in Pohang, SE Korea, Journal of the Geological Society of Korea, 42(1), p79-94, (in Korean with English abstract).
- Kim, Y.-S., Jin, K., Choi, W.-H., and Kee, W.-S., (2011), Understanding of active faults: A review for recent researches, Journal of the Geological Society of Korea, 47(6), p723-752, (in Korean with English abstract).
-
Kim, Y.-S., Peacock, D.C.P., and Sanderson, D.J., (2003), Mesoscale strike-slip faults and damage zones at Marsalforn, Gozo Island, Malta, Journal of Structural Geology, 25(5), p793-812.
[https://doi.org/10.1016/s0191-8141(02)00200-6]
-
Kim, Y.-S., Peacock, D.C.P., and Sanderson, D.J., (2004), Fault damage zones, Journal of Structural Geology, 26(3), p503-517.
[https://doi.org/10.1016/j.jsg.2003.08.002]
-
Kim, Y.-S., and Sanderson, D.J., (2004), Similarities between strike-slip faults at different scales and a simple age determining method for active faults, Island Arc, 13(1), p128-143.
[https://doi.org/10.1111/j.1440-1738.2003.00410.x]
-
Kim, Y.-S., and Sanderson, D.J., (2005), The relationship between displacement and length of faults: a review, Earth-Science Reviews, 68, p317-334.
[https://doi.org/10.1016/j.earscirev.2004.06.003]
- Kim, Y.-S., and Sanderson, D.J., (2008), Earthquake and fault propagation, displacement and damage zones, In: Landowe, S.J., Hammler, G.M. (eds.), Structural Geology: New Research, Nova Sciences, Hauppauge, New York, p99-117.
- King, G., Klinger, Y., Bowman, D., and Tapponnier, P., (2005), Slip-partitioned surface breaks for the 2001 Kokoxili earthquake, China (Mw 7.8), Bulletin of the Seismological Society of America, 95(2), p731-738.
- King, G.C., and Nabelek, J., (1985), The role of fault bends in faults in the initiation and termination of earthquake rupture, Science, 228, p984-987.
- King, G.C.P., Stein, R.S., and Lin, J., (1994), Static stress changes and the triggering of earthquakes, Bulletin of the Seismological Society of America, 84(3), p935-953.
-
King, G.C.P., and Wesnousky, S.G., (2007), Scaling of fault parameters for continental strike-slip earthquakes, Bulletin of the Seismological Society of America, 97(6), p1833-1840.
[https://doi.org/10.1785/0120070048]
-
Klinger, Y., (2010), Relation between continental strike-slip earthquake segmentation and thickness of the crust, Journal of Geophysical Research, 115, pB07306.
[https://doi.org/10.1029/2009jb006550]
- Klinger, Y., Choi, J.-H., and Vallage, A., (2016), Fault branching and long-term earthquake rupture scenario for strike-slip earthquakes, In: Thomas, M.Y., Mitchell, T.M., Bhat, H.S. (eds.), Fault Zone Dynamic Processes: Evolution of Fault Properties During Seismic Rupture, American Geophysical Union and John Wiley & Sons, Inc. (accepted).
-
Klinger, Y., Etchebes, M., Tapponier, P., and Narteau, C., (2011), Characteristic slip for five great earthquakes along the Fuyun Fault in China, Nature Geosciences, 4, p389-392.
[https://doi.org/10.1038/ngeo1158]
-
Klinger, Y., Michel, R., and King, G.C.P., (2006), Evidence for an earthquake barrier model from Mw ~7.8 Kokoxili (Tibet) earthquake slip-distribution, Earth and Planetary Science Letters, 242(3-4), p354-364.
[https://doi.org/10.1016/j.epsl.2005.12.003]
- Klinger, Y., Xu, X., Tapponnier, P., Van der Woerd, J., Laserre, C., and King, G.C.P., (2005), High-resolution satellite imagery mapping of the surface rupture and slip distribution of the Mw ~7.8, November 14, 2001 Kokoxili Earthquake (Kunlun fault, Northern Tibet, China), Bulletin of the Seismological Society of America, 95(5), p1970-1987.
- Knuepfer, P.L.K., (1989), Implication of the characteristics of end-points of historical surface ruptures for the nature of fault segmentation, U.S. Geological Survey Open File Report, 89-315, 193-228.
- Kyung, J.B., (2003), Paleoseismology of the Yangsan fault, southeastern part of Korean peninsula, Annals of Geophysics, 46, p983-996.
- Kyung, J.B., (2010), Paleosismological study and evaluation of maximum earthquake magnitude along the Yangsan and Ulsan fault zones in the southeastern part of Korea, Geophysics and Geophysical Exploration, 13(3), p187-197, (in Korean with English abstract).
- Kyung, J.B., and Chang, T.-W., (2001), The lastest fault movement on the Northern Yangsan fault zone around the Yugye-Ri area, southeast Korea, Journal of Korean Earth Science Society, 37(4), p563-577, (in Korean with English abstract).
- Kyung, J.B., and Lee, K., (2006), Active fault study of the Yangsan fault system and Ulsan fault system, southeastern part of the Korean Peninsula, Special Volume of the Journal of the Korean Geophysical Society, 9, p219-230.
- Kyung, J.-B., Lee, K., Okada, A., Watanabe, M., Suzuki, Y., and Takemura, K., (1999), Study of fault characteristics by trench survey in the Sangchon-ri area in the southern part of Yangsan fault, southeastern Korea, Journal of Korean Earth Science Society, 20(1), p101-110, (in Korean with English abstract).
- Lee, B.J., Choi, S.-J., Chwae, U.-C., and Ryoo, C.-R., (1999), Characteristics of the Quaternary faulting of the Wolpyeong, Yangsan, S.E. Korea, Journal of the Geological Society of Korea, 35(3), p179-188, (in Korean with English abstract).
-
Lee, J., Rezaei, S., Hong, Y., Choi, J.-H., Choi, J.-H., Choi, W.-H., Rhee, K.-W., and Kim, Y.-S., (2015), Quaternary fault analysis through a trench investigation on the northern extension of the Yangsan fault at Dangu-ri, Gyungju-si, Gyeongsangbuk-do, Journal of the Geological Society of Korea, 51(5), p471-485, (in Korean with English abstract).
[https://doi.org/10.14770/jgsk.2015.51.5.471]
- Lee, K., (2010), Comments on seismicity and crustal structure of the Korean peninsula, Geophysics and Geophysical Exploration, 13(3), p256-267, (in Korean with English abstract).
- Lee, K., and Jin, Y.G., (1991), Segmentation of the Yangsan fault system: Geophysical studies on major faults in the Kyeongsang Basin, Journal of the Geological Society of Korea, 27(4), p434-449.
- Lee, K., and Na, S.H., (1983), A study of microearthquake activity of the Yangsan fault, Journal of the Geological Society of Korea, 19(3), p127-135, (in Korean with English abstract).
-
Lettis, W., Bachhuber, J., Witter, R., Brankman, C., Randolph, C.E., Barka, A., Page, W.D., and Kaya, A., (2002), Influence of releasing step-overs on surface fault rupture and fault segmentation: examples from the 17 August 1999 Izmit earthquake on the North Anatolian fault, Turkey, Bulletin of the Seismological Society of America, 92(1), p19-42.
[https://doi.org/10.1785/0120000808]
-
Lozos, J.C., Oglesby, D.D., Duan, B., and Wesnousky, S.G., (2011), The effects of double fault bends on rupture propagation: A geometrical parameter study, Bulletin of the Seismological Society of America, 101(1), p385-391.
[https://doi.org/10.1785/0120100029]
- Maercklin, N., Festa, G., Colombelli, S., and Zollo, A., (2012), Twin ruptures grew to build up the giant 2011 Tohoku, Japan, Scientific Reports, 2, p709.
-
Manighetti, I., Campillo, M., Bouley, S., and Cotton, F., (2007), Earthquake scaling, fault segmentation, and structural maturity, Earth and Planetary Science Letters, 253(3-4), p429-438.
[https://doi.org/10.1016/j.epsl.2006.11.004]
-
Manighetti, I., Campillo, M., Sammis, C., Mai, P.M., and King, G., (2005), Evidence for self-similar, triangular slip distributions on earthquakes: Implications for earthquake and fault mechanics, Journal of Geophysical Research, 110, pB05302.
[https://doi.org/10.1029/2004jb003174]
-
Massonnet, D., Rossi, M., Carmona, C., Adragna, F., Peltzer, G., Feigl, K., and Rabaute, T., (1993), The displacement field of the Landers earthquake mapped by radar interferometry, Nature, 364, p138-142.
[https://doi.org/10.1038/364138a0]
-
McGill, S., and Sieh, K.E., (1991), Surficial offsets on the Central and Eastern Garlock fault associated with prehistoric earthquakes, Journal of Geophysical Research, 96(B13), p21597-21621.
[https://doi.org/10.1029/91jb02030]
- McCalpin, J.P., and Nelson, A.R., (2009), Introduction to Paleoseismology, In: Paleoseismology, McCalpin, J.P., (2nd ed.), International Geophysics Series v. 95, Elsevier Publishing, p1-25.
-
Mignan, A., Danciu, L., and Giardini, D., (2015), Reassessment of the maximum fault rupture length of strike-slip earthquakes and inference on Mmax in the Anatolian Peninsula, Turkey, Seismological Research Letters, 86(3), p890-900.
[https://doi.org/10.1785/0220140252]
- Milliner, C.W.D., Sammis, C., Allam, A.A., Dolan, J.F., Hollingsworth, J., Leprince, S., and Ayoub, F., (2016), Resolving fine-scale heterogeneity of co-seismic slip and the relation to fault structure. Scientific Reports, 6, p27201.
-
Nielsen, S., and Knopoff, L., (1998), The equivalent strength of geometrical barriers to earthquakes, Journal of Geophysical Research, 103(B5), p9953-9965.
[https://doi.org/10.1029/97jb03293]
-
Norio, O., Ye, T., Kajitani, Y., Shi, P., and Tatano, H., (2011), The 2011 eastern Japan great earthquake disaster: Overview and comments, International Journal of Disaster Risk Science, 2(1), p34-42.
[https://doi.org/10.1007/s13753-011-0004-9]
-
Oglesby, D.D., and Mai, P.M., (2012), Fault geometry, rupture dynamics and ground motion from potential earthquakes on the North Anatolian Fault under the Sea of Marmara, Geophysical Journal International, 188(3), p1071-1087.
[https://doi.org/10.1111/j.1365-246x.2011.05289.x]
-
Okada, A., Watanabe, M., Sato, H., Jun, M.S., Jo, W.R., Kim, S.K., Jeon, J.S., Chi, H.C., and Oike, K., (1994), Active fault topography and trench survey in the central part of the Yangsan fault, southeast Korea, Journal of Geography, 103, p111-126, (in Japanese with English abstract).
[https://doi.org/10.5026/jgeography.103.2_plate3]
-
Park, J.-C., Kim, W., Chung, T.W., Baag, C.-E., and Ree, J.-H., (2007), Focal mechanisms of recent earthquakes in the Southern Korean Peninsula, Geophysical Journal International, 169(3), p1103-1114.
[https://doi.org/10.1111/j.1365-246x.2007.03321.x]
-
Peacock, D.C.P., and Sanderson, D.J., (1991), Displacement, segment linkage and relay ramps in normal fault zones, Journal of Structural Geology, 13(6), p721-733.
[https://doi.org/10.1016/0191-8141(91)90033-f]
-
Perrin, C., Manighetti, I., Ampuero, J.-P., Cappa, F., and Gaudemer, Y., (2016), Location of largest earthquake slip and fast rupture controlled by along-strike change in fault structural maturity due to fault growth, Journal of Geophysical Research, 121(5), p3666-3685.
[https://doi.org/10.1002/2015jb012671]
-
Peyrat, S., Olsen, K., and Madariaga, R., (2001), Dynamic modeling of the 1992 Landers earthquake, Journal of Geophysical Research, 106(B11), p26467-26482.
[https://doi.org/10.1029/2001jb000205]
-
Poliakov, A.N., Dmowska, R., and Rice, J.R., (2002), Dynamic shear rupture interactions with fault bends and off-axis secondary faulting, Journal of Geophysical Research, 107(B11), p2295.
[https://doi.org/10.1029/2001jb000572]
- Quigley, M., Van Dissen, R., Villamor, P., Litchfield, N., Barrell, D., Furlong, K., Stahl, T., Duffy, B., Bilderback, E., Noble, D., Townsend, D., Begg, J., Jongens, R., Ries, W., Claridge, J., Klahn, A., Mackenzie, H., Smith, A., Hornblow, S., Nicol, R., Cox, S., Langridge, R., and Pedley, K., (2010), Surface rupture of the Greendale Fault during the Mw 7.1 Darfield (Canterbury) Earthquake, New Zealand: initial findings, Bulletin of the New Zealand Society for Earthquake Engineering, 43(4), p236-242.
- Reid, H.F., (1910), The mechanics of the earthquake, In: Lawson, A.C. (chariman), Vol. II of the California earthquake of April 18, 1906: Report of the state earthquake investigation commission, Carnegie Institution of Washington Publication 87, (reprinted in 1969).
-
Reilinger, R.E., Ergintav, S., Burgmann, R., McClusky, S., Lenk, O., Barka, A., Gurkan, O., Hearn, L., Feigl, K.L., Cakmak, R., Aktug, B., Ozener, H., and Toksoz, M.N., (2000), Coseismic and postseismic fault slip for the 17 August 1999, M = 7.5, Izmit, Turkey earthquake, Science, 289(5484), p1519-1524.
[https://doi.org/10.1126/science.289.5484.1519]
- Ren, Z., Zhang, Z., Chen, T., Yan, S., Yin, J., Zhang, P., Zheng, W., Zhang, H., and Li, C., (2015), Clustering of offsets on the Haiyuan fault and their relationship to paleoearthquakes, Geological Society of America Bulletin, 128(1-2), p3-18.
-
Rockwell, T.K., and Klinger, Y., (2013), Surface rupture and slip distribution of the 1940 Imperial Valley Earthquake, Imperial Fault, Southern California: implications for rupture segmentation and dynamics, Bulletin of the Seismological Society of America, 103(2A), p629-640.
[https://doi.org/10.1785/0120120192]
-
Salisbury, J.B., Rockwell, T.K., Middelton, T.J., and Hudnut, K.W., (2012), Lidar and field observations of slip distribution for the most recent surface ruptures along the Central San Jacinto Fault, Bulletin of the Seismological Society of America, 102(2), p598-619.
[https://doi.org/10.1785/0120110068]
-
Schlupp, A., and Cisternas, A., (2007), Source history of the 1905 great Mongolian earthquakes (Tsetserleg, Bolnay), Geophysical Journal International, 169(3), p1115-1131.
[https://doi.org/10.1111/j.1365-246x.2007.03323.x]
-
Scholz, C.H., (2002), The Mechanics of Earthquakes and Faulting, (2nd Ed.), Cambridge University Press, Cambridge, United Kingdom.
[https://doi.org/10.1017/cbo9780511818516]
-
Schwartz, D.P., and Coppersmith, K. J., (1984), Fault behavior and characteristic earthquakes: Examples from the Wasatch and San Andreas fault zones, Journal of Geophysical Research, 89(B7), p5681-5698.
[https://doi.org/10.1029/jb089ib07p05681]
-
Schwartz, D.P., Haeussler, P.J., Seitz, G.G., and Dawson, T.E., (2012), Why the 2002 Denali fault rupture propagated onto the Totschunda fault: Implications for fault branching and seismic hazards, Journal of Geophysical Research, 117, pB11304.
[https://doi.org/10.1029/2011jb008918]
-
Segall, P., and Pollard, D.D., (1980), Mechanics of discontinuous faults, Journal of Geophysical Research, 85(B8), p4337-4350.
[https://doi.org/10.1029/jb085ib08p04337]
- Sharp, R.V., Budding, K.E., Boawright, J., Ader, M.J., Bonilla, M.G., Clark, M.M., Fumal, T.E., Harms, K.K., Lienkaemper, J.J., Morton, D.M., O’Neill, B.J., Ostergren, C.L., Ponti, D.J., Rymer, M.J., Saxton, J.L., and Sims, J.D., (1989), Surface faulting along the Superstition Hills fault zone and nearby faults associated with the earthquake of 12 November 1987, Bulletin of the Seismological Society of America, 79(2), p252-281.
-
Shaw, B.E., and Scholz, C.H., (2001), Slip-length scaling in large earthquakes: Observations and theory and implications for earthquake physics, Journal of Geophysical Research, 28(15), p2995-2998.
[https://doi.org/10.1029/2000gl012762]
-
Shelly, D.R., (2010), Migrating tremors illuminate complex deformation beneath the seismogenic San Andreas fault, Nature, 463, p648-652.
[https://doi.org/10.1038/nature08755]
-
Sibson, R.H., (1985), Stopping of earthquake ruptures at dilational fault jogs, Nature, 316, p248-251.
[https://doi.org/10.1038/316248a0]
-
Sibson, R.H., (1989), Earthquake faulting as a structural process, Journal of Structural Geology, 11(1-2), p1-14.
[https://doi.org/10.1016/0191-8141(89)90032-1]
-
Sibson, R.H., (2003), Thickness of the seismic slip zone, Bulletin of the Seismological Society of America, 93(3), p1169-1178.
[https://doi.org/10.1785/0120020061]
-
Sibson, R., Ghisetti, F., and Ristau, J., (2011), Stress control of an evolving strike-slip fault system during the 2010-2011 Canterbury, New Zealand, earthquake sequence, Seismological Research Letters, 82(6), p824-832.
[https://doi.org/10.1785/gssrl.82.6.824]
-
Sieh, K.E., and Jahns, R.H., (1984), Holocene activity of the San Andreas fault at Wallace Creek, California, Geological Society of America Bulletin, 95(8), p883-896.
[https://doi.org/10.1130/0016-7606(1984)95<883:haotsa>2.0.co;2]
-
Sieh, K., Jones, L., Hauksson, E., Hudnut, K., Eberhart-Phillips, D., Heaton, T., Hough, S., Hutton, K., Kanamori, H., Lilje, A., Lindvall, S., McGill, S.F., Mori, J., Rubin, C., Spotila, J.A., Stock, J., Thio, H.K., Treiman, J., Wernicke, B., and Zachariasen, J., (1993), Near-field investigations of the Landers earthquake sequence, April to July 1992, Science, 260(5105), p171-176.
[https://doi.org/10.1126/science.260.5105.171]
- Slemmons, D.B., and McKinney, R., (1977), Definition of “Active Fault”. U.S. Army Engineer Waterways Experiment Station Soil and Pavements Laboratory, paper S-77-8, Final Report, p22.
-
Smith-Konter, B.R., Sandwell, D.T., and Shearer, P., (2011), Locking depths estimated from geodesy and seismology along the San Andreas Fault System: Implications for seismic moment release, Journal of Geophysical Research, 116(B6).
[https://doi.org/10.1029/2010jb008117]
-
Soliva, R., Benedicto, A., Schultz, R.A., Maerten, L., and Micarellie, L., (2008), Displacement and interaction of normal fault segments branched at depth: Implications for fault growth and potential earthquake rupture size, Journal of Structural Geology, 30(10), p1288-1299.
[https://doi.org/10.1016/j.jsg.2008.07.005]
- Son, M., Song, C.W., Kim, M.-C., Cheon, Y., Jung, S., Cho, H., Kim, H.-G., Kim, J.S., and Sohn, Y.K., (2013), Miocene crustal deformation, basin development, and tectonic implication in the southeastern Korean Peninsula, Journal of the Geological Society of Korea, 49(1), p93-118, (in Korean with English abstract).
-
Stein, R.S., Barka, A.A., and Dieterich, J.H., (1997), Progressive failure on the North Anatolian fault since 1939 by earthquake stress triggering, Geophysical Journal International, 128(3), p594-604.
[https://doi.org/10.1111/j.1365-246x.1997.tb05321.x]
-
Stein, S., and Okal, E.A., (2011), The size of the 2011 Tohoku earthquake need not have been a surprise, EOS, 92(27), p227-228.
[https://doi.org/10.1029/2011eo270005]
-
Teran, O.J., Fletcher, J.M., Oskin, M.E., Rockwell, T.K., Hudnut, K.W., Spelz, R.M., Akciz, S.O., Hernandez-Flores, A.P., and Morelan, A.E., (2015), Geologic and structural controls on rupture zone fabric: A field-based study of the 2010 Mw 7.2 El Mayor-Cucapah earthquake surface rupture, Geosphere, 11(3), p899-920.
[https://doi.org/10.1130/ges01078.1]
-
Thomas, M.Y., Avouac, J.-P., Gratier, J.-P., and Lee, J.-C., (2014), Lithological control on the deformation mechanism and the mode of fault slip on the Longitudinal Valley Fault, Taiwan, Tectonophysics, 632, p48-63.
[https://doi.org/10.1016/j.tecto.2014.05.038]
- Vallage, A., Klinger, Y., Grandin, R., Bhat, H.S., and Pierrot-Deseilligny, M., (2015), Inelastic surface deformation during the 2013 Mw 7.7 Balochistan, Pakistan, earthquake, Geology, 43(1), p1079-1082.
- Vallee, M., Landes, M., Shapiro, N.M., and Klinger, Y., (2008), The 14 November 2001 Kokoxili (Tibet) earthquake: High-frequency seismic radiation originating from the transitions between sub-Rayleigh and supershear rupture velocity regimes, Journal of Geophysical Research, 113(B7).
- Wald, D., and Heaton, T., (1994), Spatial and temporal distribution of slip for the 1992 Landers, California, earthquake, Bulletin of the Seismological Society of America, 84(3), p668-691.
-
Walsh, J.J., Bailey, W.R., Childs, C., Nicol, A., and Bonson, C.G., (2003), Formation of segmented normal faults: A 3-D perspective, Journal of Structural Geology, 25(8), p1251-1262.
[https://doi.org/10.1016/s0191-8141(02)00161-x]
- Ward, S.N., (1997), Dogtails versus rainbows: Synthetic earthquake rupture models as an aid in interpreting geological data, Bulletin of the Seismological Society of America, 87(6), p1422-1441.
- Wesnousky, S.G., (1994), The Gutenberg-Richter or characteristic earthquake distribution, which is it?, Bulletin of the Seismological Society of America, 84(6), p1940-1959.
-
Wesnousky, S., (2006), Predicting the endpoints of earthquake ruptures, Nature, 444(7117), p358-360.
[https://doi.org/10.1038/nature05275]
-
Wesnousky, S., (2008), Displacement and geometrical characteristics of earthquake surface ruptures: Issues and implications for seismic-hazard analysis and the process of earthquake rupture, Bulletin of the Seismological Society of America, 98(4), p1609-1632.
[https://doi.org/10.1785/0120070111]
-
Westoby, M.J., Brasington, J., Glasser, N.F., Hambrey, M.J., and Reynolds, J.M., (2012), ‘Structure-from-Motion’ photogrammetry: A low-cost, effective tool for geoscience applications, Geomorphology, 179, p300-314.
[https://doi.org/10.1016/j.geomorph.2012.08.021]
-
Woo, S., Lee, H., Han, R., Chon, C.-M., Son, M., and Song, I., (2015), Frictional properties of gouges collected from the Yangsan Fault, SE Korea, Journal of the Geological Society of Korea, 51(6), p569-584, (in Korean with English abstract).
[https://doi.org/10.14770/jgsk.2015.51.6.569]
-
Xu, X.W., Yu, G.H., Klinger, Y., Tapponnier, P., and Van der Woerd, J., (2006), Reevaluation of surface rupture parameters and faulting segmentation of the 2001 Kunlunshan earthquake (Mw 7.8), northern Tibetan Plateau, China, Journal of Geophysical Research, 111, pB05316.
[https://doi.org/10.1029/2004jb003488]
-
Yang, J.-S., and Lee, H.-K., (2014), Quaternary fault activity of the Yangsan fault zone in the Samnam-myeon, Ulju-gun, Ulsan, Korea, Economic and Environmental Geology, 47(1), p17-27, (in Korean with English abstract).
[https://doi.org/10.9719/eeg.2014.47.1.17]
-
Yoo, H., Herrmann, R.B., Cho, K.H., and Lee, K., (2007), Imaging the three-dimensional crust of the Korean peninsula by joint inversion of surface-wave dispersion and teleseismic receiver functions, Bulletin of the Seismological Society of America, 97(3), p1002-1011.
[https://doi.org/10.1785/0120060134]
-
Yoon, S.H., and Chough, S.K., (1995), Regional strike slip in the eastern continental margin of Korea and its tectonic implications for the evolution of Ulleung Basin, East Sea (Sea of Japan), Geological Society of America Bulletin, 107(1), p83-97.
[https://doi.org/10.1130/0016-7606(1995)107<0083:rssite>2.3.co;2]
-
Zielke, O., and Arrowsmith, J.R., (2008), Depth variation of coseismic stress drop explains bimodal earthquake magnitude-frequency distribution, Geophysical Research Letters, 35, pL24301.
[https://doi.org/10.1029/2008gl036249]
- Zielke, O., and Arrowsmith, J.R., (2012), LaDiCaoz and LiDARimager-MATLAB GUIs for LiDAR data handling and lateral displacement measurement, Geosphere, 8(1), p206-221.
-
Zielke, O., Arrowsmith, J.R., Grant Ludwig, L., and Akciz, S.O., (2010), Slip in the 1857 and earlier large earthquakes along the Carrizo Plain, San Andreas Fault, Science, 327(5969), p1119-1122.
[https://doi.org/10.1126/science.1182781]
-
Zielke, O., Arrowsmith, J.R., Grant Ludwig, L., and Akciz, S.O., (2012), High-resolution topography-derived offsets along the 1857 Fort Tejon earthquake rupture trace, San Andreas fault, Bulletin of the Seismological Society of America, 102(3), p1135-1154.
[https://doi.org/10.1785/0120110230]
-
Zielke, O., Klinger, Y., and Arrowsmith, J.R., (2015), Fault slip and earthquake recurrence along strike-slip faults - Contributions of high-resolution geomorphic data, Tectonophysics, 638, p43-62.
[https://doi.org/10.1016/j.tecto.2014.11.004]
-
Zinke, R., Hollingsworth, J., and Dolan, J.F., (2014), Surface slip and off-fault deformation patterns in the 2013 Mw 7.7 Balochistan, Pakistan earthquake: Implications for controls on the distribution of near-surface coseismic slip, Geochemistry, Geophysics, Geosystems, 15(12), p5034-5050.
[https://doi.org/10.1002/2014gc005538]
-
Zoback, M.L., (2006), The 1906 earthquake and a century of progress in understanding earthquakes and their hazards, GSA Today, 16(4-5), p4-11.
[https://doi.org/10.1130/gsat01604.1]