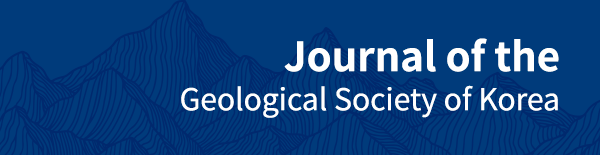
백악기 경상분지 진주층 상부 셰일층 내에 발달하는 점완형 정단층군과 방해석 광맥계의 형성 기작
초록
이번 연구는 백악기 경상분지 진주층 상부의 암회색 셰일층 내에 집중되어 발달하는 정단층군과 단층면 내지 셰일층의 층리에 평행하게 배열되는 방해석맥의 산출 상태와 형성 기작을 이해하는데 목적이 있다. 진주휴게소와 사천시 축동면의 절개사면에서 확인되는 정단층들은 대부분 셰일층을 절단하나 상·하부의 사암층까지 연장되지 못하고, 다양한 주향, 점완형의 기하, 정이동성 내지 횡인장성 운동감각, 그리고 2 m 이내의 수직변위를 보인다. 또한 단층 내지 전단단열면에서 확인되는 조선들은 방사상 인장 환경을 지시한다. 한편, 정단층들과 같이 셰일층 내에서 주로 관찰되는 섬유상의 방해석맥은 지층의 층리와 평행한 광맥(비프)과 단층과 평행하거나 단층면을 따라 충전된 광맥으로 구분된다. 3 cm 이내의 두께와 수 m ~ 수십 m의 연장을 가지는 비프는 주로 모암의 파편을 함유한 암색의 중앙선을 보이며, 섬유상의 방해석 결정은 층리면에 수직하거나 사교한다. 정단층 인접부에서 집중되어 발달하는 단층면에 평행한 광맥은 1 cm 이내의 두께와 수 m의 연장을 가져 비프에 비해 두께와 연속성이 좋지 않으며, 흑색의 중앙선이 관찰되지 않는 경우도 있다. 단층과 전단단열 그리고 방해석맥 간의 선후관계는 단층운동에 수반된 지층의 변형과 방해석맥의 형성이 지질학적으로 동일한 시기에 반복적으로 발생하였음을 지시한다. 또한 단층 인접부에서 단층과 평행한 광맥이 밀집되어 분포하고 하반에 비해 변형이 집중된 단층의 상반에서 비프의 산출 빈도가 높은 특징은 정단층운동과 이에 수반된 변형들이 탄산염 물질을 포함하는 유체의 흐름을 제어하는데 중요한 역할을 하였음을 시사한다. 또한 이러한 연구결과들은 점완형정단층군과 방해석맥의 형성이 지구조적 응력에 의한 영향이기 보다는 퇴적층이 분지 심부에서 하중에 의해 다짐작용을 받는 동안 상대적으로 연성도와 압밀되는 정도가 우세한 셰일층 내에서 유체의 과압력이 반복적으로 발생한 결과로 추정된다.
Abstract
This paper focuses on the occurrence and formation mechanism of normal faults and calcite veins within the shale-dominant strata of the upper Jinju Formation, Cretaceous Gyeongsang Basin. Normal faults observed in two cut slopes (Jinju Service Station and Chukdong-myeon sites) offset shale strata, but do not cut the upper and lower sandstones. They are characterized by various strikes, normal and transtensional slip senses, vertical offsets less than 2 m, as well as radial tensional paleo-stress field calculated from slip data of faults and shear fractures. Fibrous calcite veins observed mostly in the shale strata are grouped into bedding-parallel and fault-parallel (or fault-filling) veins. Bedding-parallel veins (beef), which are less than 3 cm in thickness and a few to tens of meters in length, often show black median lines including host rock particles. The orientations of calcite fibers are perpendicular or oblique to the bedding. Fault-parallel and -filling veins less than 1 cm in thickness and of a few meter in length are thinner and shorter than the beef veins, and often do not show median line. Relative chronologies of the faults, shear fractures, and veins indicate that the faulting and the injection of calcite veins were repeatedly occurred during the geologically same time period. In addition, both the fault-parallel and beef veins are concentratedly observed in more deformed hanging-wall adjacent to the fault surfaces, indicating that the normal faulting and associated structures played an important role in the migration of carbonate-rich fluids. These results also imply that the normal faults and calcite veins were formed as a result of fluid overpressure caused by differential compaction of incompetent shale strata in the deep part of basin, rather than regional tectonic stress.
Keywords:
normal fault, calcite vein, shale, compaction, overpressure키워드:
정단층, 방해석맥, 셰일층, 다짐작용, 과압력1. 서 언
단층은 하나 또는 그 이상의 불연속면이 단속적 또는 연속적으로 발달하는 지각의 대표적인 약대로, 전단작용에 의한 물리/화학적 변형/변질로 인해 모암의 특성이 변화된 물질들(단층암)로 흔히 채워진다(e.g., Sibson, 1977; Caine et al., 1996). 단층암의 특성에 따라 단층대는 지하 유체의 이동 통로(conduit) 또는 장벽(barrier)이 되며, 반대로 유체 압력은 단층 운동의 결정적인 원인이 되기도 한다. 또한 이러한 수리학적 특성은 단층대를 따라 시공간적으로 다양하게 변화될 수 있다(Lunn et al., 2008 and references therein). 따라서 암석 내의 유체 흐름과 광물 이동을 기록하고 있는 광맥은 단층과 같은 단열의 형성과 유체 사이의 상호작용을 이해하는데 있어 중요한 단서가 된다.
정단층은 광역적인 지구조적 응력 이외에도 지각내부의 여러 원인에 의해 형성되어 다양한 양상을 보일 수 있다(Davis and Raynolds, 1996; Twiss and Moore, 2007; Fossen, 2010). 지구조적 인장환경에서 만들어진 정단층들은 대부분 체계적인 기하와 운동감각으로 일정한 방향의 최소수평응력을 지시하는 반면(e.g., Bosworth, 1985; Twiss and Moore, 2007; Son et al., 2013, 2015), 비지구조적(non-tectonic) 환경에서 형성된 정단층들은 흔히 특정 지점 또는 층준에 집중되며, 상반의 이동방향이 방사상(radial movement)을 보일 가능성이 높다(e.g., Cartwright, 1994, 2011; Hibsch, 2003).
이번 연구는 백악기 경상분지 진주층 상부의 암회색 셰일층이 우세한 층준에서 집중적으로 발달하는 점완형 정단층군과 셰일층의 층리 또는 단층면을 따라 주입된 방해석 광맥들의 산출 상태와 형성 기작을 이해하는데 목적이 있다. 이를 위해 노출된 절개사면에서 단층에 의해 구획되는 지괴를 설정하여 지괴별 변형양상을 상세 기재하고, 이들 자료를 바탕으로 단층에 수반된 변형과 방해석 광맥 간의 선후관계 그리고 이들의 형성 기작을 논의하고자 한다.
2. 지질학적 배경
이번 연구의 대상은 경상남도 진주시 호탄동의 남해고속도로 진주휴게소(부산방향)에 위치한 절개사면(35° 09ʺ 38.43ʺ‘N, 128° 07ʺ 27.26ʺ‘E)과 사천시축동면에 위치한 절개사면(35° 06ʺ 11.23ʺ‘N, 128° 03ʺ 58.35ʺ‘E) 노두이며, 이들 절개사면에는 백악기 경상분지 신동층군의 최상부층인 진주층이 노출되어 있다. 경상분지는 국내 최대 규모의 백악기 퇴적분지로서 한반도 남동부에 위치하며, 사교섭입(oblique subduction)하는 고태평양판(proto-Pacific plate)의 영향에 의해 형성된 배호분지(back arc basin)로 해석된다(그림 1; Chough and Sohn, 2010). 약 9 km의 두께의 분지충전물은 화산물질이 거의 포함되지 않은 신동층군, 화산물질을 일부 포함하는 하양층군, 다량의 화산물질로 구성된 유천층군 그리고 이들을 관입하는 불국사화강암류로 구분된다(Chang, 1975; Choi, 1986; Chang et al., 2003; 그림 1).
호성환경에서 퇴적된 진주층은 주로 회색 내지 암회색의 역질 사암, 중·조립질 사암, 미사암 그리고 암회색 내지 흑색의 이암과 셰일이 호층을 이루며 발달하고 부분적으로 응회질 사암이 협재하며, 상부로갈수록 이암과 셰일이 우세하게 발달한다. 진주층 사암에서 추출된 쇄설성 저콘의 SHIRIMP U-Pb 연대측정 결과들은 진주층이 하부 앨비안(lower Albian)동안 퇴적된 것으로 보고하고 있다(Lee, T.-H. et al., 2010; Lee, Y.I. et al., 2010). 연구노두들은 진주층의 상부에 위치하며 흑색 셰일, 중립질 사암 그리고 이회암이 교호하며 발달하고, 특히 진주휴게소에 위치한 절개사면의 하부에는 스트로마톨라이트와 석회질 단괴가 관찰된다(Ha et al., 2015).
3. 정단층군과 방해석맥의 기재학적 특성
3.1 진주휴게소 절개사면
절개사면 상부에 주로 관찰되는 육안으로 변위가 뚜렷한 정단층 내지 횡인장성(transtension) 사교이동단층은 다양한 주향을 가지나 동-서 주향이 우세하며, 대부분 하부로 갈수록 경사각이 완만해지는 점완형 기하를 보인다(그림 2). 단층을 따라 단층각력이나 비지와 같은 단층암은 전혀 관찰되지 않으며, 단층 조선, 홈(groove) 그리고 수다자국(chatter mark)이 뚜렷한 전단면이 발달한다. 또한 이들 단층면을 따라 방해석 광맥이 주입된 특징을 관찰할 수 있다. 단층들의 수직 변위는 2 m 이내이며, 운동감각은 특정한 최소수평응력 방향을 가리키지 않고 방사상으로 발산하는 인장 응력을 지시한다(그림 2). 한편, 이들 단층과 수반되어 육안으로는 변위가 인지되지 않으나 뚜렷한 조선을 보이는 다양한 방향의 전단단열들이 함께 관찰된다. 이들의 운동감각 또한 정이동성 내지 횡인장성 사교이동을 보이며 방사상인장 환경을 지시한다(그림 2).

Outcrop photograph showing structural domains and their border faults at Jinju Service Station. Inset: two lower hemisphere, equal-area nets show the various orientations and normal- to normal oblique-slip senses of faults (left) and shear fractures (right), indicating radial extension. Rʺ=R (σ1 is vertical), or 2-R (σ2 is vertical), or 2+R (σ3 is vertical) [Choi (1995), Delvaux et al. (1997); R=(σ2-σ3)/(σ1-σ3)].
사면 중·상부 셰일층에 발달하는 방해석맥계는 (1) 지층의 층리면을 따라 주입된 광맥(비프, beef)과 (2) 단층과 평행하거나 단층면을 따라 충전된 광맥으로 구분된다. 층리를 따라 발달하는 비프는 맥벽(vein wall)에 거의 수직하거나 사교하는 섬유상의 빗 구조(comb structure)를 보이며, 3 cm 이내의 두께와 수 m에서 수십 m의 연장을 가진다. 연구노두의 방해석 비프 산상에 대한 기존 연구(Ha et al., 2015)는 방해석맥 내부에 나타나는 세립의 방해석 결정, 유체포유물, 모암의 파편 등으로 구성된 암회색 내지 흑색의 중앙선(median line)의 존재를 근거로 이들 광맥이 이향광맥(antitaxial vein)이며 외부에서 이동된 유체에 의해 형성되었을 것으로 추정하였다. 또한 다수의 비프에서 S자형 섬유상 조직, 작은 조각으로 분리된 모암의 고체 포유물, 비프 내 중앙선의 후기 변형 그리고 다중 성장을 지시하는 복합 광맥(composite vein)의 특징에 근거하여(Ha et al., 2015의 그림 3과 4), 이곳 비프의 형성 과정은 복잡하며 상당 기간 동안 지속되었을 것으로 해석하였다. 한편, 단층면과 평행하게 발달하는 방해석맥은 1 cm 이내의 두께와 수 m의 연장을 가지고 있어 비프에 비해 두께가 얇고 연속성이 떨어지며, 방해석맥 내에 중앙선이 잘 관찰되지 않는다.

Outcrop photographs and diagrams showing the major structural features of Domains 1 and 2. (a) E-W-striking fault 1 dividing Domains 1 and 2. (b) Deformed strata and (c) NNE-trending recumbent folds occurring in the northern and lower part of Domain 1. (d) Sinistral-normal oblique-slip striation observed on calcite vein wall. (e) Soft-sediment deformation structure near the recumbent fold. (f) Structural data of Domain 1. Contoured (left) and rose (middle) diagrams show a best-fitting π-axis of stratal attitudes and their dip directions, respectively, indicating a NE-trending fold. Lower hemisphere, equal-area net (right) shows the distribution of slip senses on fault and shear fracture surfaces. (g) Structural data of Domain 2. Contoured (left) and rose (middle) diagrams show a WNW-trending drag fold adjacent to Fault 1. Lower hemisphere, equal-area net (right) shows the west-southwestward normal slip of faults. All orientation data are described in quadrant notation and third component indicates the rake of slickenline on fault or shear fracture surfaces.

Outcrop photographs and diagrams showing the major structural features of Domains 3 and 4. (a) Sinistral-normal oblique-slip striation on Fault 5 surface. (b) Fault 6 dividing Domains 3 and 4-1, which was filled by 5 mm-thick fibrous calcite vein. Bidirectional arrows indicate the orientation of crystal fibers. (d) Fault-filling and -parallel calcite veins observed along Fault 4, which divides Domains 4-2 and 6. (e) Structural data of Domain 3. Contoured (left) and rose (middle) diagrams indicate homoclinal east-dipping strata. Stereo diagram (right) shows the northwest to southwest normal-slip of fault and shear fractures. Structural data of Domains 4-1 (f) and 4-2 (g). Contoured (left) and rose (middle) diagrams show the mainly northwest-dipping strata of the domains. Stereo diagrams (right) also indicate a radial extension.
정단층의 영향을 거의 받지 않은 절개사면 하단의 지층들은 경상분지의 전반적인 지층의 자세와 유사하게 N10~20°E/10~15°SE로 동사구조(homocline)를 보인다. 이곳 방해석 비프들에서는 후기 변형이 인지되지 않으며, 셰일층을 따라 대부분 수십 m로 측방 연속성이 좋다. 반면 정단층의 영향을 받은 절개사면 상단의 지층들은 정단층에 의해 구획되는 지괴에 따라 서로 다른 변형양상이 확인된다. 각 지괴별 변형특성은 다음과 같다.
지괴1은 절개사면의 가장 북쪽에 위치한 단층1 (N89°W/45°NE)의 상반에 해당된다(그림 2의 D1, 3a). 단층1은 하부로 갈수록 경사각이 얕아지며, 단층면을 따라 그리고 인접부에는 단층면과 평행 또는 아평행한 방해석 광맥들이 다수 관찰된다. 지괴1의 가장 북쪽 하부는 사질층이 없는 두꺼운 셰일층으로 구성되는데, 이곳 지층은 특이하게 심하게 교란되어 습곡된 양상을 보여준다(그림 3b). 관찰된 습곡은 N34°E/05°의 축과 N20°E/21°SE의 축면을 가지는 횡와습곡(recumbent fold)의 기하를 보인다(그림 3c). 습곡된 지층의 층리를 따라 발달하는 비프 방해석맥들은 습곡의 날개에서 힌지로 갈수록 두께가 얇아지며 빗 구조에서 보여주는 광물의 성장방향은 습곡의 위치에 관계없이 모두 지표에 거의 수직한 특징을 보여주고 있어, 습곡의 형성 동시 또는 이후에 광맥들이 충전되었음을 시사해준다. 또한 층리와 평행하게 주입된 광맥면 상에는 정이동성 내지 횡인장성 사교이동의 운동감각을 지시하는 조선도 관찰되는데(그림 3d), 이는 비프 광맥들의 형성 이후에도 층간에 전단작용이 발생하였음을 지시한다. 한편, 지괴1에서 측정된 전체 층리 자세는 북서와 동남동 방향으로 경사진 것들이 우세하며 북동 방향의 축을 가지는 습곡의 존재를 지시한다(그림 3f). 전단단열들의 운동감각은 전체적으로 방사상 인장환경을 지시하지만, 단층1을 포함한 주변 전단단열들이 좌수향의 횡인장성 운동감각을 가지며 단층의 상반이 서북서 내지 북서 방향으로 이동한 특징을 보인다(그림 3f). 이러한 특징은 단층 1의 상반이 북서쪽으로 빠르게 침강하면서 상대적으로 강인성(competency)이 약한 두꺼운 셰일층에 굴름배사(rollover anticline)와 함께 국지적 수직 압축력이 집중되어, 상반의 이동 방향에 거의 직교하는 북동 방향의 축을 가지는 횡와습곡이 형성되었을 가능성을 지시한다. 또한 이렇게 습곡된 지층 내에는 연성변형구조(soft-sediment deformation structure)가 흔히 관찰되어(그림 3e), 변형 당시에는 지층이 완전히 암석화되지 않았음을 암시한다.
지괴2는 단층1 (N89°W/~45°NE)의 하반, 단층2 (N61°E/~36°NW)의 상반에 해당한다(그림 2의 D2, 3a). 이곳 지층의 전반적인 자세는 절개사면 하단에 위치하는 지층의 자세(N10~20°E/10~15°SE)와 유사하나, 단층1의 인접부에서는 정단층운동에 수반되어 단층의 주향 방향과 거의 평행한 서북서의 축을 가지는 완만한 끌림습곡(dragging folding)이 인지된다(그림 3g). 한편 지괴2에서의 비프 광맥 산출빈도는 지괴1에 비해 현저히 낮으며, 단층1과 2의 인접부에는 단층과 대체로 평행한 방해석맥들이 3 m이내의 짧은 연장으로 관찰되는데 이들의 분포는 단층의 상반에 집중되는 경향을 보여, 단층의 상반이 하반에 비해 변형이 우세함을 지시한다.
지괴3은 단층2 (N61°E/~36°NW)와 단층6 (N59°E/~22°SE)의 하반에 해당하며(그림 2의 D3, 4b), 지괴 내부에 수십 cm의 수직변위를 가지는 단층5 (N45°E/48°NW)가 발달한다(그림 4a). 단층6의 단층면에는 5 mm 이내 두께를 가지는 방해석맥이 충전되어 있으며, 방해석맥 내부에 발달하는 섬유상 조직의 성장 방향은 단층의 경사 방향과 동일한 방향으로 고각의 경사를 보인다(그림 4c). 지괴3의 지층은 단층운동에 수반된 내부 교란 없이 전반적으로 저각으로 동쪽으로 경사지며, 북서~남서 방향으로 상반이 침강하는 정이동 내지 정이동성 사교이동감각의 전단단열들이 다수 확인된다(그림 4e). 한편, 층리를 따라 발달하는 비프 광맥은 거의 관찰되지 않는다.
지괴4는 지괴4-1과 4-2로 구분하여 구조분석을 실시하였다. 지괴4-1은 단층6 (N59°E/~22°SE)과 단층7 (N71°E/~40°NW)의 상반에 해당한다(그림 2의 D4-1, 4b, 5a). 지괴 내부의 지층들은 단층운동에 의해 일부 교란되어 있지만, 북서로 경사진 지층이 우세하다(그림 4f). 지괴4-2는 단층10 (N61°E/~36°SE)과 단층4 (N75°E/~36°NW)의 상반에 해당하며 지괴4-1과 유사하게 지층의 북서향 경동이 우세하다(그림 2의 D4-2, 4d, 4g). 지괴4의 지층들이 일부 교란에도 불구하고 북서향 경동이 우세한 까닭은 남동쪽으로 경사진 단층6과 단층10의 점완형 기하와 정이동성 운동감각에 기인한 결과로 해석된다. 한편, 지괴4-1과 4-2의 지층 내에는 2 cm 이내의 두께를 가지는 방해석 비프가 다수 확인되고 단층6과 단층4의 상반 인접부에는 단층면과 평행한 수 mm 두께의 방해석맥이 관찰되어(그림 4b, 4c, 4d), 하반에 해당하는 지괴3과 6-1에 비해 방해석맥의 산출 빈도가 높은 경향을 보인다.
지괴5는 단층6 (N59°E/~22°SE)의 상반, 단층7 (N71°E/~40°NW)과 10 (N61°E/~36°SE)의 하반에 해당한다(그림 2의 D5, 5a, 5b). 이들 단층면에는 방해석맥이 충전되어 있는데, 특히 단층7의 단면에서는 당겨열림형(pull-apart) 공간 내에 방해석맥이 충전되어 있다(그림 5c). 그러나 단층면을 따라 발달하는 방해석맥 내 광물의 성장방향은 지표에 수직하거나 단층의 경사보다 고각으로 동일한 방향으로 경사지며 성장되어 있으며(그림 5c, 5d, 5g), 광맥 표면에는 정이동성 감각의 단층조선이 함께 관찰되기도 한다(그림 5e). 지괴5 내에는 북북서 방향으로 경사진 동북동 방향의 소규모 단층(단층8, 단층9; 그림 2, 5f, 5h)뿐만 아니라 정이동성 내지 정이동성 사교이동 감각을 가지는 다양한 주향의 전단단열들이 발달하며(그림 5i, 5j), 교란된 셰일층의 층리를 따라 2 cm이내의 두께를 가지는 방해석 비프가 다수 확인된다(그림 5a).

Outcrop photographs and diagrams showing the major structural features of Domain 5. (a) Border faults 7 and 10 of the domain showing a conjugate geometry and (b) its close-up view. (c) Parallelogram-shaped and (d) planar injection of calcite veins along Fault 7. Bidirectional arrows indicate the orientation of crystal fibers. (e) Normal slip sense on the fault-filling calcite vein wall. (f) Fault 10 dividing Domain 5 and 4-2 and (g) calcite vein injected along the fault surface. (h) Fault 8 within Domain 5, which is antithetic to Fault 10. (i) Shear fracture showing a sinistral-normal oblique-slip sense. (j) Structural data of Domain 5. Contoured (left) and rose (middle) diagrams show dispersedly tilted-strata with east- to southeastward dominance. Stereo diagram (right) shows a northwest to southwestward radial extension.
지괴6은 지괴6-1과 6-2로 구분하여 구조분석을 실시하였다. 지괴6-1은 단층4 (N75°E/36°NW)와 11 (N67°W/4°SW)의 하반, 단층10 (N61°E/36°SE)과 12 (N88°E/29°NW)의 상반에 해당한다(그림 2의 D6-1, 6a, 6c). 단층11 주변의 전단단열면에서 확인되는 조선들은 정이동성 내지 횡인장성 운동을 지시하며(그림 6b), 단층12를 따라 발달하는 방해석맥은 하부로 갈수록 주변 지층의 층리면에 평행해지는 경향을 보인다(그림 6d). 지괴6-1의 지층들은 전반적으로 북쪽으로 경사져 있으며(그림 6e), 층리와 평행한 방해석 비프는 단층10의 상반 인접부의 셰일층 내에서만 국부적으로 관찰된다. 지괴6-2는 단층11 (N67°W/~14°SW)과 13 (N9~16°W/~20°NE)의 상반, 단층12 (N88°E/~29°NW)의 하반에 해당한다(그림 2의 D6-2, 6f, 6g). 저각의 경사를 가지는 단층13 하부의 상반에 해당하는 지괴6-2 내의 방해석 비프의 산출 빈도는 하반에 해당하는 지괴7 내의 산출 빈도에 비해 훨씬 높은 특징을 보인다(그림 6f). 또한 50° 이상의 경사를 가지는 단층13 상부의 상반 인접부에는 정단층운동에 수반되어 만들어진 남남동 방향의 저각의 축을 가지는 끌림습곡과 이들 습곡된 지층을 따라 주입된 방해석 비프 그리고 단층과 평행하게 발달하는 방해석맥들이 밀집되어 분포한다(그림 6g-i).

Outcrop photographs and diagrams showing the major structural features of Domain 6. (a) Domains 6-1 and 6-2 divided with Fault 11. (b) Dextral normal oblique-slip striation of E-W-striking shear fracture adjacent to Fault 11. (c) Fault 12 within Domain 12 and (d) its infilling fibrous calcite veins showing a listric geometry. (e) Contoured (left) and rose (right) diagrams showing the northward homoclinal tilting of strata in Domain 6. (f) Domains 6-2 and 7 divided by Fault 13 and pervasive calcite veins in the hanging wall of the fault. (g, h) An upward extension of Fault 13 with a dip of 55° and its associated dragged and offset beef veins. (i) Structural data of Domain 6-2. Contoured (left) and rose (middle) diagrams of stratal attitudes near Fault 13 indicate a SSE-trending fold.
지괴7은 단층13 (N9~16°W/~20°NE)의 하반, 단층15 (N83°W/40°NE)의 상반에 해당한다(그림 2의 D7, 6f, 7b). 지괴 내부에 발달하는 단층14 (N85°W/34°NE)의 단층활면을 따라 약 10 cm의 수직 변위와 우수향 횡인장성 단층조선이 확인된다(그림 7a). 20 cm 이내의 수직 변위가 인지되는 단층15의 인접부에는 단층과 평행하게 배열된 5 mm 이내 폭의 방해석맥과 10 mm 내외 폭의 비프가 다수 관찰되는데, 이러한 방해석맥들은 단층의 상반에 집중되어 분포하는 경향을 보인다(그림 7c). 또한 이비프와 단층과 평행한 광맥들은 절단관계가 없이 동일한 광물 성장 방향을 가지고 연결되어 있어(그림 7c-g), 동일한 시기에 동일한 기작에 의해 형성되었음을 지시한다.
지괴8은 단층 15의 하반에 해당하는 지괴로서 이보다 남쪽 사면에서는 정단층이 나타나지 않는다. 이 지괴 내에서는 지층면의 교란이 인지되지 않으며 수십 m의 연속성 있는 비프들이 20~30 cm의 간격으로 분포한다.

Outcrop photographs showing the major structural features of Domain 7. (a) Fault 14 within Domain 7 showing a normal separation of about 10 cm. (b) Fault 15 dividing Domain 7 and 8. (c-g) Bedding-parallel and fault-parallel calcite veins observed along Fault 15. Note that these two type veins are continuously connected and show a similar direction of crystal fibers without any cross-cutting relationship. Small dotted circles in Figs. 7f and 7g indicate the connection parts of the veins.
3.2 사천시 축동면 절개사면
사천시 축동면에 위치한 절개사면은 북서 방향으로 약 130 m의 연장을 가지며, 사면의 하부에는 비프가 발달하는 셰일층이 분포하는 반면, 상부에는 비프가 관찰되지 않는 중립질 사암층이 분포한다(그림 8a). 또한 셰일층에 집중되어 발달하는 단층들은 북동과 동-서 방향의 주향이 우세하며 단면에서 1 m 이내의 수직변위를 가지는 정단층의 기하를 보인다. 이 단층들은 상부의 사암을 절단하지 못한다. 단층 인근에 발달하는 전단단열면 상의 조선들은 대부분 정이동성 운동감각을 보이며, 진주휴게소에서와 유사한 방사상 인장환경을 지시하고 있다(그림 8a). 셰일 층리면을 따라 발달하는 방해석 비프는 정단층에 의해 절단되거나 휘어진 양상을 보여주고 있어(그림 8b, 8c, 8e) 비프가 단층 이전에 만들어졌음을 지시한다. 한편, 일부 비프 광맥들은 단층면을 사교하며 절단하거나(그림 8b), 단층 말단부에 단층운동에 의해 굴곡된 지층을 따라 발달한 광맥들은 굴곡된 층리의 변곡점(inflection point)에서 두께가 얇아지는 특징을 보여주고 있어(그림 8c) 단층운동 이후에도 비프 광맥이 주입된 것으로 해석된다. 이러한 구조요소의 기하와 선후관계는 방해석맥의 주입이 정단층운동의 이전과 이후 또는 동시에 반복하여 발생하였음을 지시한다.

Outcrop photographs showing the characteristics of normal faults and calcite veins at Chukdong-myeon, Sacheon-si. (a) Normal faults only developed in shale-dominant strata. Inset: lower hemisphere, equal-area projections of the faults (left) and shear fractures (right) indicating a radial extension. (b) Beef veins and normal faults showing their relative chronologies. Note that the normal faults cut the beef and vise versa. (c) Pinch-out geometry of beefs near the inflection point of normal-fault-related drag fold, indicating vein injection after faulting. (d, e) Highly deformed stratal layers in the hangingwall of normal fault showing the rotated directions of beef crystal fibers. Bidirectional arrows indicate the orientation of crystal fibers.
4. 토 의
4.1 점완형 정단층군과 방해석맥의 특징과 선후관계
이번 연구에서 관찰된 점완형 정단층들은 (1) 셰일이 우세한 특정 층준에서만 집중적으로 분포하고, (2) 다양한 주향과 정이동성 내지 횡인장성 운동감각 그리고 2 m 이내의 수직변위를 보인다. (3) 단층과 전단단열면의 조선을 이용해 복원한 고응력장의 응력비(Rʺ)는 0.237 (진주휴게소 절개사면)과 0.15(사천시 축동면 절개사면)로서, 최대수평응력(σhmax)과 최소수평응력(σhmin) 크기의 차이가 거의 없는 방사상 인장환경을 지시한다(그림 2, 8a; Choi, 1995; Delvaux et al., 1997). 또한 (4) 단층에 의해 구획된 지괴들은 경계 단층의 기하와 운동감각에 따라 서로 다른 변형양상을 보인다.
셰일층 내에서 관찰되는 방해석맥은 층간을 충전하는 비프 광맥 그리고 단층면을 충전하거나 단층에 거의 평행하게 배열된 광맥으로 구분된다. 빗 구조를 보이는 비프와 단층과 평행한 광맥들이 대부분 지표에 거의 수직하게 서로 유사한 광물 성장 방향을 보이고 이들이 절단관계 없이 서로 연결된다는 점 그리고 횡와습곡된 지층의 힌지 또는 단층에 의해 굴곡된 지층의 변곡점 부근에서 비프의 두께가 얇아지는 특징들은 방해석 광맥의 주입이 단층 또는 습곡과 동시이거나 이후임을 지시한다. 반면, 단층에 의해 절단되거나 휘어진 비프 광맥 그리고 다수의 비프와 단층과 평행한 광맥의 맥벽에서 관찰되는 조선들은 방해석맥의 형성 이후에도 단층활동이 있었음을 지시한다. Ha et al. (2015)의 연구에서도 비프 내의 S자형 섬유상 조직, 중앙선이 절단된 양상, 비프 간의 상호절단관계 그리고 복합 광맥의 형태를 보고하고 있어, 정단층 운동과 방해석맥계의 형성이 일정 기간 동안 반복되었음을 지지해준다.
한편, 정단층에 의한 변형의 영향이 거의 없는 지괴 내에서는 수십 m의 연장을 가지는 비프가 1 m 당 5~10매 관찰되는 반면, 단층에 의한 변형이 집중된 지괴, 특히 단층 인접부에서 단층과 평행한 방해 석맥이 밀집되고 또한 하반에 비해 단층 상반에 보다 비프의 산출 빈도가 높은 특징을 보인다. 이러한 특징은 정단층들이 방해석맥계 형성을 주도한 근본원인은 아닐지라도 탄산염 유체의 흐름을 제어하는 역할을 하였음을 지시한다.
4.1 점완형 정단층군과 방해석맥의 특징과 선후관계
정단층은 지구 내부의 다양한 원인에 의해 형성될 수 있으나, 특히 지구조적 인장 내지 횡인장 환경 하에서 형성된 정단층들은 특정 층준 또는 지역에 국한되지 않고 광역적인 분포를 보인다(Allen and Allen, 2013). 또한 이들은 동향(synthetic)과 반향(antithetic)단층들로 지구(graben) 또는 반지구(half graben)를 형성하며, 일정한 방향성을 가지는 최소수평응력을 흔히 보여준다(Twiss and Moore, 2007; Cheon et al., 2012; Son et al., 2015). 반면, 비지구조 환경의 다양한 지질현상으로도 정단층들이 만들어질 수 있는데, 하부의 관입체에 의해 형성된 돔구조(다이아피어)를 절단하는 경우와 화산활동이 발생한 화도 주변에서 중력에 의해 함몰이 발생하는 경우(칼데라)가 대표적이다. 이러한 환경에서 형성된 정단층들은 방사상 또는 원형의 기하를 가져 특정한 수평응력방향을 지시하기 어려우며, 특정한 지역에 제한되어 분포하는 경우가 우세하다(Twiss and Moore, 2007). 특히 다양한 주향을 가지며 세립질 퇴적층 내에서만 국한되어 발달하는 다각 단층계(polygonal fault system)는 전 세계 100개 이상의 퇴적분지 내에서 확인되며(Cartwright, 2011), 이들의 형성 원인은 중력에 의한 붕괴, 퇴적층의 밀도구배 상태, 지층 간의 차별적인 다짐작용에 수반된 탈수, 공극수압의 증가, 화학적 속성작용 동안 발생하는 부피 수축(contraction) 등으로 다양하게 해석된다(Jones and Addis, 1984; Cartwright, 1994, 2011; Cartwright and Lonergan, 1996; Cartwright and Dewhurst, 1998; Hibsch et al., 2003; Neagu et al., 2010; Tewksbury et al., 2014). 주의해야 할 점은 이러한 비지구조적 응력환경에서 형성된 정단층도 당시 지구조적 응력의 영향을 복합적으로 받을 수 있다(Hibsch et al., 2003 and references therein).
앞서 제시한 진주층 상부에 발달하는 정단층군의 특징들은 이들이 지구조 원인보다는 비지구조 원인에 의해 형성되었음을 알려준다. 진주 일원에서 화산 내지 심성활동의 증거가 인지되지 않을 뿐만 아니라 다양한 주향의 단층들이 셰일이 우세한 층준에서만 집중되어 분포한다는 점, 단층을 따라 취성변형의 산물인 단층 비지와 각력과 같은 단층암이 산출되지 않는다는 점 그리고 수 mm에서 수 cm 단위의 연성변형구조가 빈번하게 확인되는 점은 정단층 운동이 퇴적층이 완전히 암석화되기 이전 속성작용을 받는 동안 높은 공극률과 낮은 투수율을 가지는 셰일층 내부의 물리적 또는 화학적 특성의 변화와 관련이 있음을 암시한다. 실제 세립질 지층이 조립질 지층에 비해 지하에서 하중에 의해 압밀되는 정도와 방출되는 공극수의 양이 클 뿐만 아니라(Rieke and Chilingarian, 1974; Velde, 1996; Allen and Allen, 2013), 점토광물의 함량이 우세하여 화학적 속성작용의 정도가 높다(Shin, 2008; Cartwright, 2011).
일반적인 퇴적분지 내 정암압 환경(lithostatic condition)에서는 중력의 영향으로 최대주응력(σ1)이 지표에 수직이고 최소주응력(σ3)이 지표에 수평으로 놓이기 때문에, 지표에 수직한 단열과 이를 충전한 광맥에 비해 수평의 인장 단열 또는 비프의 형성이 쉽지 않다. 하지만 (1) 층리가 지표에 수평하지 않거나 (2) 암석이 이방성으로 층리에 수직한 방향의 인장 강도가 가장 약할 경우 또는 (3) 광역적인 수평 횡압축력이 작동될 때, 층리와 평행한 단열과 광맥이 형성될 수 있다(Cobbold et al., 2013 and references therein). 수평의 인장 단열과 비프의 형성을 설명하는 또 다른 기작은 지표에 수직한 응력이 인장응력이 될 때로서, 이러한 환경은 공극유체의 과압력(overpressure; 유체압력이 정암압보다 큰 상태)이 작동하는 지하에서 형성될 수 있다(Cobbold et al., 2013). 퇴적분지 내의 공극유체에 의한 과압력은 지구조적 압축 또는 퇴적층의 다짐작용에 의한 공극의 감소, 탄화수소의 형성, 열적 팽창 또는 함수광물의 탈수작용에 의한 공극수 양의 증가 등 다양한 원인에 의해서 발생한다(Swarbrick et al., 2001; Cobbold et al., 2013).
유체의 과압력에 의해 지표에 수직한 응력이 최소주응력이 됨을 설명하는 주요 모델로는 첫째, 지표에 수직한 방향의 과압력 구배(overpressure gradient)에 의해 유체가 상승하고자 하는 힘, 즉 수직 누수력(seepage force)이 발생하고 이 힘이 하중을 넘어서게 되면 수직 응력이 인장 유효응력(tensile effective stress)으로 변화된다는 것이 있다(Mourgues and Cobbold, 2003; Cobbold and Rodrigues, 2007; Cobbold et al., 2013). 이러한 과정에서 인장성 수압단열(tensile hydraulic fracturing) 또는 팽창성 전단파괴(dilatant shear failure)가 발생하며, 암석이 등방성이고 지구조적 응력이 존재하지 않는 환경에서도 누수력만으로 비프 또는 누추(cone-in-cone) 구조를 만들 수 있음을 보고하였다. 두 번째 모델은 지하 심부 퇴적층은 수평으로 작동하는 봉압(confining pressure)으로 인해 오직 하중에 의한 지표에 수직한 일축압축변형(uniaxial compressive strain)을 겪게 되며, 이로 인해 지표에 수직한 유효응력이 수평한 유효응력에 비해 모암의 푸아송비(Poissonʺs ratio)로 인해 유체압의 영향을 많이 받아 상대적으로 빨리 인장 또는 전이 포락선(tensile or transitional failure envelope)에 도달한다는 것이다(Jamison, 2013). 세 번째는 유체압력의 증가와 해소에 의해 최소유효응력(σ3(eff))의 방향이 변화한다는 모델로서, 낮은 투수도를 가진 암석으로 덮여 있는 퇴적층 내에 유체압력이 꾸준히 증가하게 되면(z축은 수직방향으로 σ1, x축과 y축은 수평방향으로 각각 σ2와 σ3의 초기응력 상태인 경우), 먼저 σy(eff)가 인장파괴포락선에 도달하여 y축과 지표에 수직한 인장절리가 형성되는 즉시 σy는 공극수압과 같아져 σy(eff)은 0이 되고, 계속되는 유체압력의 증가에 σx(eff)가 두 번째로 인장파괴포락선에 도달하여 앞서 형성된 단열과 지표에 수직한 새로운 인장단열들이 만들어져 σx(eff)도 0이 되고, 이후 유체압의 증가로 마지막으로 σz(eff)가 인장파괴포락선에 도달하면서 지표에 수평한 인장단열이 만들어져, 수직하게 상호 절단하는 두 개의 수직 수압단열군과 하나의 수평 수압단열군이 형성된다는 것이다(Colletini et al., 2006). 하지만 이 모델로 예상되는 서로 수직하게 교차하는 단열계의 기하는 연구노두에서 확인되는 비프와 단층에 평행하게 배열되는 광맥의 기하와는 맞지 않는다.
이번 연구에서 확인되는 방해석 비프들은 대부분 셰일이 우세한 층준에서만 발달하고 이회암과 사암내에서는 관찰되지 않으며, 지층의 변형 이후에 형성된 비프의 경우에도 다양한 경사를 가지는 지층의 층간을 따라서 충전된 양상을 보인다. 이러한 특징들은 셰일층 내에서 발생한 높은 유체압력과 함께 층리에 수직한 방향의 낮은 인장 강도가 비프 형성에 중요한 원인임을 지시해 준다.
퇴적분지 내에서의 높은 공극수압은 포화된 불투수성 퇴적층(water-saturated impermeable sediment)내에서 쉽게 발생되며, 특히 유기물이 풍부한 셰일층은 탄화수소의 대표적 근원암으로서 지하 심부에서 탄화수소의 형성 또는 하중에 의한 다짐작용에 따른 공극수압으로 쉽게 과압력 상태에 놓이게 된다(Cartwright, 1994; Twiss and Moore, 2007; Allen and Allen, 2013). 따라서 경상분지의 규모와 성숙도를 고려할 때, 진주층 상부에 집중적으로 분포하는 셰일층이 지하 심부에서 속성작용을 받는 동안 공극수압의 증가와 공극수의 배출로 과압력이 반복적으로 발생하고 해소되었을 가능성이 높다.
이번 연구에서 얻어진 여러 증거들은 셰일이 우세한 층준에 집중적으로 분포하는 점완형 정단층과 방해석맥의 형성이 상대적으로 강인성이 큰 사암체 사이에 위치하는 연성도가 높은 셰일층 내에서 반복적으로 발생/해소되는 유체의 과압력과 관련이 있음을 시사한다(Allen and Allen, 2013; 그림 9). 이러한 과압력의 형성 원인은 퇴적 이후 세립질 물질로 이루어진 셰일층이 지하로 매몰되는 동안 셰일층 내에 하중에 의한 차등 다짐작용과 탄화수소의 생성이 가장 합리적으로 판단되나, 외부 유체의 주입에 의한 가능성도 배제할 수 없다. 이러한 환경에서 지표에 수직한 방향으로 일축압축변형이 발생하고 지하로 갈수록 공극수압의 증가로 인한 압력구배로 유체가 지표 쪽으로 상승하고자 하는 누수력이 커지면서, 지표에 수직한 유효응력이 가장 빠르게 감소하여 수직응력이 최소주응력으로 변화되어 인장 수압파쇄(tensile hydraulic fracturing) 또는 팽창성 전단파괴(dilatant shear failure)로 단층운동과 함께 방해석 비프가 생성된 것으로 해석된다(Cobbold and Rodrigues, 2007). 단층의 생성은 탄산염 유체의 흐름을 원활하게 하여 공극수압을 빠르게 감소시키는 역할을 하였을 것이며, 이후 이어지는 점진적인 공극수압의 증가로 시기가 다른 방해석 광맥들이 형성되는 사건이 일정 기간 반복되었을 가능성이 높다.
5. 결 론
진주층 상부의 사암, 이암 그리고 셰일층으로 구성되는 진주휴게소와 사천시 축동면 사면에서 확인되는 정단층군의 기하와 운동학적 특징, 정단층에 구획된 지괴별 변형양상, 그리고 방해석맥의 산상에 대해 상세 기재하고 형성 원인을 규명하고자 하였다. 이번 연구를 통해 도출된 결과는 다음과 같다.
(1) 정단층들은 셰일이 우세한 층준에 규제되어 발달하며, 상·하부의 사암층을 절단하지 못한다. 다양한 주향을 가지는 단층들은 하부로 갈수록 경사각이 작아지는 점완형 기하와 정이동성 내지 횡인장성 운동감각 그리고 2 m 이내의 수직변위를 보이며, 단층과 전단단열의 기하와 운동감각을 이용해 복원된 고응력은 방사상의 인장환경을 지시한다. 단층으로 구획된 사면의 지괴들은 경계단층의 기하와 운동학적 특징에 따라 서로 다른 변형양상을 보여준다.
(2) 셰일층 내 방해석맥은 지층의 층리와 평행하게 배열되는 비프 그리고 단층면을 충전하거나 이와 평행한 광맥으로 구분된다. 이들은 특정 절단관계에 의한 선후가 없이 서로 연결되기도 하는 반면, 일부 비프 광맥들은 단층운동에 의해 절단되거나 변형된 양상을 보여준다. 3 cm 이내의 두께와 수 m ~ 수십 m의 연장을 가지는 비프는 모암의 파편을 포함하는 암색의 중앙선을 보여주며, 섬유상의 방해석 결정은 직선 혹은 S자형으로 층리면에 수직하거나 사교한다. 한편, 정단층 인접부에 밀집되어 발달하는 단층면에 평행한 광맥은 1 cm 이내의 두께와 수 m의 연장을 가져 비프에 비해 두께와 연속성이 좋지 않으며, 흑색의 중앙선이 관찰되지 않는 경우도 있다.
(3) 단층, 전단단열 그리고 방해석맥 간의 선후관계는 단층운동에 수반된 지층의 변형과 광맥의 형성이 지질학적으로 동시기에 일정 기간 동안 반복적으로 발생하였음을 지시한다. 또한 단층 인접부에서 단층과 평행한 방해석맥이 집중되고 단층 하반에 비해 변형의 정도가 심한 상반에서 비프의 산출 빈도가 높은 특징은 정단층운동과 이에 수반된 변형들이 탄산염 물질을 포함하는 유체의 흐름을 제어하는 주요한 역할을 하였음을 의미한다.
(4) 이상의 연구결과들을 종합하면, 셰일이 우세한 층준에 규제되어 발달하는 점완형 정단층군과 방해석맥의 형성은 지구조적 응력에 의한 영향보다는 분지 심부에서 상대적으로 강인성이 큰 상부층(사암) 아래에 놓인 연성도가 큰 지층(셰일)이 하중에 의해 차별 다짐작용을 받는 동안 반복적으로 발생한 유체 과압력의 증가 및 해소와 관련이 있을 것으로 추정된다.
Acknowledgments
이 논문은 2014년 정부(교육과학기술부)의 재원으로 한국연구재단의 지원을 받아 수행된 기초연구사업(2014R1A2A1A11053102)의 연구결과이다. 심사과정에서 건설적이고 유익한 조언과 비평을 해주신 김영석 교수님과 한래희 교수님께 감사드린다.
References
- Allen, P.A., and Allen, J.R., (2013), Basin analysis: Principles and Applications, 3rd Edition, Blackwell Scientific Publications, Oxford, p619.
-
Bosworth, W., (1985), Discussion on the structural evolution of extensional basin margins, Journal of the Geological Society, 142, p939-942.
[https://doi.org/10.1144/gsjgs.142.5.0939]
-
Caine, J.S., Evans, J.P., and Forster, C.B., (1996), Fault zone architecture and permeability structure, Geology, 24, p1025-1028.
[https://doi.org/10.1130/0091-7613(1996)024<1025:FZAAPS>2.3.CO;2]
-
Cartwright, J.A., (1994), Episodic basin-wide fluid expulsion from geopressured shale sequences in the North Sea basin, Geology, 22, p447-450.
[https://doi.org/10.1130/0091-7613(1994)022<0447:EBWFEF>2.3.CO;2]
-
Cartwright, J.A., (2011), Diagenetically induced shear failure of fine-grained sediments and the development of polygonal fault systems, Marine and Petroleum Geology, 28, p1593-1610.
[https://doi.org/10.1016/j.marpetgeo.2011.06.004]
-
Cartwright, J.A., and Dewhurst, D.N., (1998), Layer-bound compaction faults in fine-grained sediments, Geological Society of America Bulletin, 110, p1242-1257.
[https://doi.org/10.1130/0016-7606(1998)110<1242:LBCFIF>2.3.CO;2]
-
Cartwright, J.A., and Lonergan, L., (1996), Volumetric contraction during the compaction of mudrocks: a mechanism for the development of regional-scale polygonal fault systems, Basin Research, 8, p183-193.
[https://doi.org/10.1046/j.1365-2117.1996.01536.x]
- Chang, K.H., (1975), Cretaceous stratigraphy of southeast Korea, Journal of the Geological Society of Korea, 11, p1-23.
-
Chang, K.-H., Suzukib, K., Parka, S.-O., Ishida, K., and Uno, K., (2003), Recent advances in the Cretaceous stratigraphy of Korea, Journal of Asian Earth Sciences, 21, p937-948.
[https://doi.org/10.1016/S1367-9120(02)00142-6]
-
Cheon, Y., Son, M., Song, C.W., Kim, J.-S., and Sohn, Y.K., (2012), Geometry and kinematics of the Ocheon Fault System along the boundary between the Miocene Pohang and Janggi basins, SE Korea, and its tectonic implications, Geosciences Journal, 16, p253-273.
[https://doi.org/10.1007/s12303-012-0029-0]
-
Choi, H.I., (1986), Sedimentation and evolution of the Cretaceous Gyeongsang Basin, southeastern Korea, Journal of the Geological Society, 143, p29-40.
[https://doi.org/10.1144/gsjgs.143.1.0029]
- Choi, P.Y., (1995), Aspects of stress inversion methods in fault tectonic analysis, Annales Tectonicae, 9, p22-38.
-
Chough, S.K., and Sohn, Y.K., (2010), Tectonic and sedimentary evolution of a Cretaceous continental arc-backarc system in the Korean peninsula: New view, Earth- Science Reviews, 101, p225-249.
[https://doi.org/10.1016/j.earscirev.2010.05.004]
-
Cobbold, P.R., and Rodrigues, N., (2007), Seepage Forces, Important Factors in the Formation of Horizontal Hydraulic Fractures and Bedding-Parallel Fibrous Veins ('Beef' and 'Cone-in-Cone'), Geofluids, 7, p313-322.
[https://doi.org/10.1111/j.1468-8123.2007.00183.x]
-
Cobbold, P.R., Zanella, A., Rodrigues, N., and Loseth, H., (2013), Bedding-parallel fibrous veins (beef and cone in-cone): Worldwide occurrence and possible significance in terms of fluid overpressure, hydrocarbon generation and mineralization, Marine and Petroleum Geology, 43, p1-20.
[https://doi.org/10.1016/j.marpetgeo.2013.01.010]
-
Collettini, C., De Paola, N., and Goulty, N.R., (2006), Switches in the Minimum Compressive Stress Direction Induced by Overpressure beneath a Low-Permeability Fault Zone, Terra Nova, 18, p224-231.
[https://doi.org/10.1111/j.1365-3121.2006.00683.x]
- Davis, G.H., and Reynords, S.J., (1996), Structural Geology of Rocks and Regions, 2nd Edition, John Wiley & Sons, Inc., New York, p776.
-
Delvaux, D., Moeys, R., Stapel, G., Petit, C., Levi, K., Miroshnichenko, A., Ruzhich, V., and San'kov, V., (1997), Paleostress reconstructions and geodynamics of the Baikal region, Central Asia, Part 2. Cenozoic rifting, Tectonophysics, 282, p1-38.
[https://doi.org/10.1016/S0040-1951(97)00210-2]
-
Fossen, H., (2010), Structural Geology, Cambridge University Press, New York, p463.
[https://doi.org/10.1017/CBO9780511777806]
-
Ha, S., Chae, Y.-U., Jeong, G.Y., Son, M., Yang, K., Paik, I.S., and Lim, H.S., (2015), A preliminary study of calcite beef found in the Cretaceous Jinju Formation, Gyeongsang Basin, Korea, Journal of the Geological Society of Korea, 51, p597-604, (in Korean with English abstract).
[https://doi.org/10.14770/jgsk.2015.51.6.597]
-
Hibsch, C., Cartwright, J., Hansen, D.M., Gaviglio, P., Andre, G., Cushing, M., Bracq, P., Juignet, P., Benoit, P., and Allouc, J., (2003), Normal Faulting in Chalk: Tectonic Stresses Vs. Compaction-Related Polygonal Faulting, Geological Society, London, Special Publications, 216, p291-308.
[https://doi.org/10.1144/GSL.SP.2003.216.01.19]
- Jamison, W., (2013), Bed-parallel expansion seams and shear surfaces in shales, Geoconvention 2013 (abstract), Calgary Canada, May 6-12, p1-4.
-
Jones, M.E., and Addis, M., (1984), Volume change during sediment diagenesis and the development of growth faults, Marine and Petroleum Geology, 1, p118-122.
[https://doi.org/10.1016/0264-8172(84)90081-3]
- Lee, T.-H., Park, K.-H., Chun, J.-H., and Yi, K., (2010), SHRIMP U-Pb zircon ages of the Jinju Formation and Silla Conglomerate, Gyeongsang Basin, Journal of the Petrological Society of Korea, 19, p89-101, (in Korean with English abstract).
-
Lee, Y.I., Choi, T., Lim, H.S., and Orihashi, Y., (2010), Detrital zircon geochronology of the Cretaceous Sindong Group, Southeast Korea: implications for depositional age and Early Cretaceous igneous activity, Island Arc, 19, p647-658.
[https://doi.org/10.1111/j.1440-1738.2010.00717.x]
-
Lunn, R.J., Shipton, Z.K., and Bright, A.M., (2008), How can we improve estimates of bulk fault zone hydraulic properties?, Geological Society, London, Special Publications, 299, p231-237.
[https://doi.org/10.1144/SP299.14]
-
Mourgues, R., and Cobbold, P.R., (2003), Some Tectonic Consequences of Fluid Overpressures and Seepage Forces as Demonstrated by Sandbox Modelling, Tectonophysics, 376, p75-97.
[https://doi.org/10.1016/S0040-1951(03)00348-2]
-
Neagu, R.C., Cartwright, J., and Davies, R., (2010), Measurement of diagenetic compaction strain from quantitative analysis of fault plane dip, Journal of Structural Geology, 32, p641-655.
[https://doi.org/10.1016/j.jsg.2010.03.010]
- Rieke, H.H., and Chilingarian, G.V., (1974), Compaction of Argillaceous Sediments, Elsevier Scientific Publishing Company, New York, p423.
-
Shin, H., Santamarina, C., and Cartwright, J.A., (2008), Contraction driven shear in compacting uncemented sediments, Geology, 36, p931-934.
[https://doi.org/10.1130/G24951A.1]
-
Sibson, R.H., (1977), Fault rocks and fault mechanisms, Journal of the Geological Society, 133, p191-213.
[https://doi.org/10.1144/gsjgs.133.3.0191]
-
Son, M., Song, C.W., Kim, M.-C., Cheon, Y., Cho, H., and Sohn, Y.K., (2015), Miocene tectonic evolution of the basins and fault systems, SE Korea: dextral, simple shear during the East Sea (Sea of Japan) opening, Journal of the Geological Society Korea, 172, p664-680.
[https://doi.org/10.1144/jgs2014-079]
- Son, M., Song, C.W., Kim, M.-C., Cheon, Y., Jung, S., Cho, H., Kim, H.-G., Kim, J.S., and Sohn, Y.K., (2013), Miocene Crustal Deformation, Basin Development, and Tectonic Implication in the Southeastern Korean Peninsula, Journal of the Geological Society of Korea, 49, p93-118, (in Korean with English abstract).
- Swarbrick, R.E., Osborne, M.J., and Yardley, G.S., (2001), Comparison of overpressure magnitude resulting from the main generating mechanisms, In: Huffman, A.R., Bowers, G.L. (Eds.), Pressure Regimes in Sedimentary Basins and Their Prediction, American Association of Petroleum Geologists Memoir, 76, p1-12.
-
Tewksbury, B.J., Hogan, J.P., Kattenhorn, S.A., Mehrtens, C.J., and Tarabees, E.A., (2014), Polygonal faults in chalk: Insights from extensive exposures of the Khoman Formation, Western Desert, Egypt. Geology, 42, p479-482.
[https://doi.org/10.1130/G35362.1]
- Twiss, R.J., and Moores, E.M., (2007), Structural Geology, 2nd Edition, .H. Freeman and Company, New York, p736.
-
Velde, B., (1996), Compaction trends of clay-rich deep sea sediments, Marine Geology, 133, p193-201.
[https://doi.org/10.1016/0025-3227(96)00020-5]