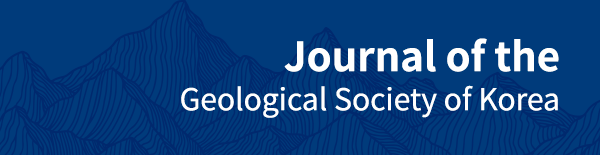
강–연안–해양 환경 내 유기탄소∙질소의 공간적 기원 변동 특성
Copyright ⓒ The Geological Society of Korea 2024
초록
본 연구는 최근 인위적 활동이 증가되고 있는 육상환경(포항 형산강)을 포함하여 인접한 해양환경(영일만, 동해)에서 물리화학적 공간 분포 변동을 파악하고 입자성 유기물 내 안정동위원소비 분석을 통하여 각 권역별 유기물 기원을 파악하고자 하였다. 형산강 내 상대적으로 높은 농도의 영양염(DIN; 1.6±1.0 mg/L, DIP; <0.1 mg/L, SiO2; 5.6±2.5 mg/L), 입자성(POC; 0.6±0.2 mg/L, PN; 0.2±0.1 mg/L) 및 용존성 유기원소(DOC; 2.7±1.3 mg/L, DTN; 1.9±1.1 mg/L) 경향과 함께, 정점간 차별적인 안정동위원소비는 주변 농지, 산업지, 도심지 내 기인한 인위적 기원의 유·무기 원소들이 지표수 유출을 통해 유입된 것이라 판단된다. 영일만-동해 내 이들 요소는 상대적으로 낮으며 서로 다른 안정동위원소 비를 보임에 따라 자생기원(식생, 식물플랑크톤)과 인위적 기원유기물(비료, 오폐수 및 연료 연소, 대기 침적) 유입 및 생성 영향을 차별적으로 나타냈다. 입자성 유기물의 탄소·질소 안정동위원 소비를 적용한 베이지안 혼합 모델 결과, 각 권역별 자연적(C3 식물, 해양 식물플랑크톤) 및 인위적(비료, 오폐수 및 연료 연소) 기원 유기물 기여도는 서로 다른 범위를 보였으며, 다량의 인위적 기원(비료; 16±7%, 오폐수 및 연소; 34±18%)들의 유출로 인해 해양환경 내 영양염-유기물 순환 변동 가능성을 파악할 수 있었다. 추후 지속적인 모니터링을 통해 대기 및 수층으로 유입될 수 있는 유·무기 유입원의 정밀한 동위원소 데이터베이스 구축이 요구되며, 이를 통해 육상-연안-해양 연결성(수생태계 물질순환 변동성 진단 및 예측)을 파악할 중요한 기초자료를 제공할 수 있을 것이라 판단된다.
Abstract
We investigated spatial variations of physicochemical components from terrestrial to marine environments (Hyeong-san River, Young-il Bay, East Sea) where recent anthropogenic activities were increasing. Based on these results, we additionally determined discriminative origins of organic matter (OM) by analyzing stable isotopic compositions of particulate OM. Together with the relatively high concentration of nutrients (DIN; 1.6±1.0 mg/L, DIP; <0.1 mg/L, SiO2; 5.6±2.5 mg/L), particulate and dissolved organic elements showed discriminative variations (POC; 0.6±0.2 mg/L, PN; 0.2±0.1 mg/L, DOC; 2.7±1.3 mg/L, DTN; 1.9±1.1 mg/L) within the Hyeong-san River. In addition, different isotopic patterns of particulate OMs indicate that anthropogenic sources within agricultural, industrial, and urban boundaries may be substantially transported through surface runoff. Meanwhile, hydrochemical properties at marine systems (Young-il Bay and East Sea) were relatively lower ranges than those of river systems. The discriminative isotopic signatures within water columns may reflect mixed source contributions between autochthonous (algae) and anthropogenic OMs (fertilizers, sewage and combustion, atmospheric deposition). Based on these results, the contributions of natural (C3 plants, marine phytoplankton) and anthropogenic (fertilizers, sewage and combustion) OMs showed significant differences between each boundary, causing that predominant anthropogenic sources (fertilizers: 16±7%, sewage and combustion: 34±18%) transported from river systems may potentially influence nutrient-organic matter cycling within the marine environment. In near future, further study via periodic monitoring is required for establishing systematical strategy (i.e., isotope database) related to the precise tracing of anthropogenic origins into the atmosphere-aquatic boundaries. Finally, these approaches provide important information for tracing biogeochemical interactions between river-estuary-ocean boundaries.
Keywords:
stable isotope, nutrient, organic matter, land-use type, Bayesian mixing model키워드:
안정동위원소, 영양염, 유기물, 토지이용형태, 베이시안 혼합 모델1. 서 론
수환경 내 유기탄소·질소는 육상 강·하천의 지표수 유출 및 수층 내 분해 등 다양한 생지화학적 과정을 거치며 순환하고 있다(Aufdenkampe et al., 2011; Bauer et al., 2013; Regnier et al., 2013; Wang et al., 2018). 특히 유기탄소·질소는 무기 탄소와 무기 질소의 생합성 과정을 통한 산물로서(Dubinsky and Berman-Frank, 2001; Frigstad et al., 2011), 생물 섭식관계(예; 영양단계)를 통해 에너지 흐름을 전달하여 수생태계 먹이구조를 형성할 수 있다(Moe et al., 2005; Branco et al., 2018; Vanderlee et al., 2021). 특히 하천을 통해 바다로 유출되는 육상유기탄소·질소의 이동량과 유출특성을 파악하는 것은, 기수역이나 연안 생태계의 생물다양성을 유지 관리하는 연구를 포함하여(Schlünz and Schneider, 2000; Smittenberg et al., 2006; McGovern et al., 2020), 지구규모의 탄소 물질순환을 해석하는 핵심 요소로서 중요성이 높아지고 있다(Schlesinger and Melack, 1981; Hedges et al., 1997; Regnier et al., 2022). 하지만 최근 육상 내 토지 이용률 변동 및 인간활동 증가로 인해 다량의 유기물 및 영양염류(질소, 인)가 배출되고 있으며 이로 인해 수생태계 에너지 흐름이 변동되고 있다(Galloway et al., 1995; Falkowski et al., 2000; Liu et al., 2020). 실제로 한국 연안환경 내 심각한 사회환경 문제(예; 부영양화, 유해조류 번성, 빈산소수괴 발생 등)가 보고됨에 따라(Huang et al., 2018; Woo et al., 2019; Yang et al., 2020; Kim et al., 2024a, 2024b), 수환경 내 체계적인 환경진단에 따른 효과적인 수질 관리방안 필요성 증대가 요구되는 실정이다.
수생태계의 건강성을 향상시키고 높은 회복력을 유지시키기 위해 국내외 환경관리 평가전략에 대한 활발한 연구가 진행되고 있다(Apitz et al., 2006; Choi et al., 2013). 국외의 경우, 국가연안평가를 통해 생태계 구성 요소인 수질, 퇴적환경, 저서생물, 서식지 그리고 어류오염 등 5가지 지표를 설정하고 연안환경을 통합적으로 평가하고 있다(U.S. EPA, 2012). 유럽은 물관리체계를 기반으로 수계의 화학적 상태(미량금속 및 유기화합물의 농도)와 생태적상태(수온, 염분, 용존산소, 영양염, 식물플랑크톤 종조성, 현존량, 대형조류 풍도, 저서동물 다양성 등)를 평가할 수 있는 인자를 통합하여 5개 등급으로 평가하고 있다(Borja and Dauer, 2008). 국내의 경우, 해양환경관리법(제9조), 어장관리법(제6조)에 근거하여 전국 연안 모니터링 사업(해양환경측정망 및 어장환경모니터링)이 운영되며, 정기적인 연안환경조사를 통해 해양환경관리 정책수립과 관련된 기초자료를 도출하고 있다(MOF and KOEM, 2024). 또한 육상 강·하천 및 호소 환경의 경우 환경정책기본법(제2조)에 근거하여 수질인자(pH, 생물학적 및 화학적 산소요구량, 용존산소, 총유기탄소량, 총인 등) 기준을 설정하여 국가수질측정망 운영을 통해 수집된 수질인자 결과를 바탕으로 수질 등급을 측정하고 있다(MOE, 2024). 하지만 지구환경 시스템 내 유기원소(예; 탄소, 질소, 수소, 산소 등)의 거동은 각 권역별 밀접한 연결성을 가짐에도 불구하고(Rabouille et al., 2001; Aichner et al., 2022; Zhang et al., 2022), 이들 원소의 거동(생성-이동-제거)에 관한 통합적인 이해는 여전히 미비한 실정이다. 이러한 관점에서 수질관리 체계 진단과 미래 유기원소 순환 변동 예측을 효과적으로 구축하기 위해서는 각 권역(강-연안-해양) 내 유기원소 기원 파악에 따른 이들의 거동을 통합적으로 이해하는 것이 중요하다.
본 연구에서는 최근 인위적 기인 활동이 증가되고 있는 육상환경(포항 형산강)을 포함하여 인접한 해양환경(영일만, 동해)에서 사례연구(case study)를 진행하였으며, 이들 환경 내 물리화학자료(수온, 염분, 용존산소, 영양염류, 용존유기탄소·질소, 입자성유기탄소·질소)의 공간적 분포 특성을 파악하고자 하였다. 더욱이 최신 화학분석기법(안정동위원소)을 이용하여 각 유기원소(탄소, 질소) 내 포함된 다양한 기원을 추적함으로써 각 권역별 차별적인 공급 기여도를 평가하고자 하였다.
2. 재료 및 방법
2.1. 시료 채집
본 연구를 위해 2023년 9월 육상(형산강 상-하류) 및 해양조사(영일만, 동해)를 동시에 수행하여 표층수를 채수하였다(그림 1). 육상 정점의 경우 포항시 내 공간적 토지이용형태 분포(포스코 산업단지, 도시 지역, 농업 지역)를 고려하여 연구정점(R1-12)을 설정하였으며, 하천변에서 저밀도 폴리에틸렌(Low-Density Polyethylene, LDPE) 용기를 이용하여 표층수를 채수하였다. 시료 채집에 사용한 LDPE용기는 현장수로 3번 세척한 뒤 사용하였다. 연안 정점(E1-6)의 경우 부경대학교 나라호 해양조사선 내 보유한 소형선박을 이용하여 표층수를 채수하였으며, 해양 정점(M1-6)의 경우 채수기(SBE32 Carousel Water Sampler, Sea-Bird Scientific, USA)를 이용하였으며, 채취된 모든 정점시료는 연구선 내 실험실에서 여과를 수행하였다. 여과된 시료는 실험실 이동 후 기기분석 전까지 -20℃에서 냉동 보관하였다.
2.2. 수층 이화학 분석
수층 물리적 특성(수온, 염분, 용존산소)은 표층수 채집과 동시에 현장에서 측정하였다. 육상권역은 다항목수질측정기(YSI 6600; Xylen Analytics, USA)를 이용하여 측정하였으며, 해양권역은 채수기에 부착된 수온염분측정기(conductivity-temperature-depth; SBE 9 plus, Sea-Bird Scientific, USA)를 이용하였다.
현장 표층수 시료는 수층 내 큰 부유 물질을 제거하기 위해 200 μm 표준망으로 거른 후, 고온(450℃, 4시간)에서 잔여 유기물을 미리 제거한 유리섬유여과지(GF/F 0.7 μm, Whatman, Germany)를 사용하여 입자성(particulate) 과 용존성(dissolved) 물질을 분리하였다. 또한 일부 여과액은 유리병에 분취 후 용존성 유기탄소(dissolved organic carbon, DOC) 및 용존성 총질소(dissolved total nitrogen, DTN) 농도 분석을 위해 염화수은(HgCl2) 처리 후 냉장(4℃) 보관하였다. 잔여 여과액은 영양염(질산염, 암모늄, 인산염, 규산염) 농도 분석을 위해 코니칼 용기(conical tube)에 분취하여 기기분석 전까지 냉동(-20℃) 보관하였다.
입자성 유기탄소(particulate organic carbon, POC) 및 입자성 질소(particulate nitrogen, PN) 농도 분석을 위해, 여과된 입자성 시료는 수분 제거를 위해 동결건조 후 1N 염산을 이용하여 데시케이터에서 24시간 증기 처리하였다. 이후 중화를 위해 수산화나트륨(NaOH)를 이용하여 24시간 동안 반응시킨 후 원소분석기(Elemental analyzer; Elementar Vario MICRO CUBE, Elementar, Langenlbold, Germany)를 이용하여 입자성 유기탄소 및 입자성 질소의 농도(%)를 분석하고 최종 여과량에 따라 농도(mg/L)를 환산하였다. 측정 중 기기 분석 정밀도는 인증표준 물질인 IAEA-CH3 (cellulose)와 IAEA-N1 (ammonium sulfate)를 사용하여 측정하였으며, 각각 ±0.1%, ±0.1%의 범위를 나타냈다.
용존성 유기탄소(DOC) 및 용존성 총 질소(DTN) 농도는 총 유기탄소 분석기(TOC-L CPH/CPN, Shimazu, Kyoto, Japan)를 사용하여 분석하였다. 측정을 위해 표준시약(DOC; WC-TOC-10X-1, AccuStandard, USA / DTN; Potassium nitrate, Sigma-aldrich, Canada)을 사용하였으며, 농도 검보정 선(DOC; 0-5 ppm, R2 = 0.99, DTN; 0-5 ppm, R2 = 0.99)을 각각 작성한 뒤, 정량분석을 수행하였다. 측정 중 기기 분석 정밀도는 1 ppm 표준시약을 사용하였으며, 각각 ±0.03 ppm, ±0.1 ppm의 범위를 나타냈다.
수층 영양염 농도는 극지연구소에서 분석되었다. 이와 관련하여, 냉동된 여과시료를 실온 해동 후 영양염 자동분석기(QuAAtro, Seal Analytical company, Southampton, UK)를 사용하여 분석하였다. 용존 무기질소의 경우 암모니아성 질소, 아질산성 질소, 질산성 질소의 합으로 나타내었다.
2.3. 안정동위원소비 분석
입자성 유기탄소(POC) 및 입자성 총 질소(PN)의 안정동위원소 비(δ13C, δ15N) 측정을 위해 동결 건조된 여과지는 산 증기 처리 및 중화 후 원소분석기-동위원소 질량분석기(EA-IRMS, VisION Elementar, Hesse, Germany)를 사용하여 분석하였다. 측정된 안정동위원소비는 국제표준물질(δ13C; Vienna Pee-Dee Belemnite, δ15N; atmospheric N2)을 사용하여 최종적으로 δ (delta) 표기법으로 환산하여 나타냈다. 측정 중 기기 분석 정밀도는 인증표준 물질인 IAEA-CH3 (cellulose, -24.7‰)와 IAEA-N1 (ammonium sulfate, 0.4‰)를 사용하여 측정하였으며, 각각 ±0.1‰, ±0.1‰의 표준편차를 나타냈다.
2.4. 통계분석
각 권역별 수층의 주요 물리화학적 특성을 파악하고 이들 사이의 상호작용을 파악하기 위해 통계프로그램 R(version 3.6.1, FactoMineR an R package, version 1.42)을 사용하여 주성분분석(principal component analysis, PCA)을 실시하였다. 통계에 사용한 물리화학 자료들은 Z-score 표준화(normalization) 작업을 통해 각 항목간 단위 차이에 따라 발생할 수 있는 분포 편향을 보정하여 사용하였다(Yunker et al., 2005). 각 권역별 수층 물리화학인자들의 상관관계(Pearson correlation) 분석은 SPSS 통계(IBM Analytics, Chicago, USA, Ver. 29.0.2)프로그램을 이용하여 분석하였다.
2.5. 베이지안(bayesian) 동위원소 혼합 모델
베이지안 동위원소 혼합 모델(Bayesian isotope mixing model)은 동위원소비 결과를 물질 수지 식(mass balance equation)에 적용한 기법으로, 베이지안 확률 통계 기법 기반의 특정 기원(유입원)의 정량적 기여율을 산정할 수 있는 기법이다(Stock et al., 2018). 본 연구에서는 통계프로그램 R 내에 MixSIAR 페키지(version 3.1.12)를 사용하여 기원별 기여도를 산정하였다. 기여도 산정에 사용한 유기물 기원(source)은 육상 내 토지이용형태를 고려하여 인공적 유입원인 비료(δ13C; -25.9±2.4‰, δ15N; 9.0±5.2‰), 오폐수 및 연료 연소(δ13C; -23.0±3.3‰, δ15N; -0.5±4.9‰)기원과 자연적 유입원인 수층 식물플랑크톤(δ13C; -19.2±2.7‰, δ15N; 6.7±4.6‰), 육상 식물(δ13C; -31.0±4.9‰, δ15N; 4.4±6.1‰)을 선정하여 각각의 안정동위원소비를 모델에 대입하였다(Lee, Y. et al., 2017; Derrien et al., 2018; Hong et al., 2019; Lee, H.S. et al., 2021; Kim et al., 2022; Mie et al., 2022). 또한 수층 내 채집한 입자성 유기탄소, 입자성 총 질소를 모델 내에 혼합물로 지정하고 이들의 동위원소(δ13C, δ15N)값을 대입하여 각 권역별 유기탄소 및 질소 기원의 기여도를 산정하였다.
3. 연구 결과
3.1. 권역별 수층 이화학 및 유기원소(탄소·질소)
각 권역별 수층 물리화학적 특성(수온, 염분, 용존산소, 영양염, 입자성·용존성 유기탄소, 입자성·용존성 총 질소)의 공간적 분포를 비교하였다(그림 2; 표 1). 각 권역별(형산강, 영일만, 동해) 표층 수온은 각각 26.9±1.2℃, 26.0±0.6℃, 26.1±0.3℃의 범위를 나타냈다(그림 2a). 염분은 권역별 각각 3.6±2.9 psu, 30.9±2.5 psu, 32.1±0.1 psu의 범위를 보였다(그림 2b). 용존산소는 권역별 각각 7.4±0.8 mg/L, 5.5±1.1 mg/L, 4.5±0.1 mg/L의 범위를 보였다(그림 2c).
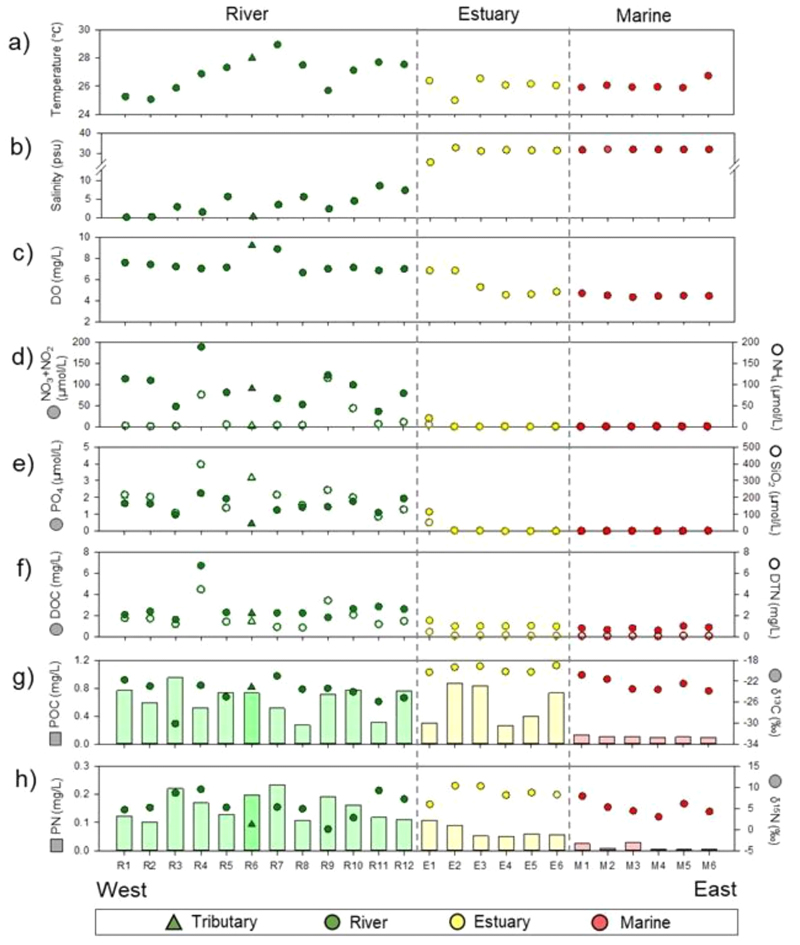
Spatial variations of physico-chemical properties (a-h; temperature, salinity, dissolved oxygen, NOx-, NH4+, PO43-, SiO2-, DOC, DTN, POC (concentration, isotopes), PN (concentration, isotopes)). The concentration and stable isotopic composition of POC and PN are represented by colored rectangles and circles, respectively.
수층 영양염은 각 권역별 차별적인 농도 범위를 보였다(형산강, DIN; 1.6±1.0 mg/L, DIP; <0.1 mg/L, SiO2; 5.6±2.5 mg/L, 영일만, DIN; 0.1±0.1 mg/L, DIP; <0.1 mg/L, SiO2; 0.3±0.6 mg/L, 동해, DIN, DIP, SiO2; <0.1 mg/L) (그림 2d-e). 용존성 유기탄소 및 질소의 농도는 형산강에서 2.7±1.3 mg/L, 1.9±1.1 mg/L의 범위를 보였으며, 해양환경에서 1.0±0.2 mg/L, 0.2±0.1 mg/L의 범위를 보였다(그림 2f). 입자성 유기탄소 및 질소의 농도는 형산강에서 0.6±0.2 mg/L, 0.2±0.1 mg/L의 범위를 보였으며, 해양환경에서 0.3±0.3 mg/L, 0.1 mg/L이하의 범위를 보였다(그림 2g-h).
각 권역별 수층 입자성 유기탄소 및 총 질소의 안정동위원소비는 형산강에서 -24.1±2.4‰, 5.3±3.0‰, 영일만에서 -19.7±0.6‰, δ15NPN; 8.6±1.6‰, 동해에서 -22.6±1.2‰, δ15NPN; 5.2±1.7‰의 범위를 보였다(그림 2g-h; 표 1).
3.2. 주성분 분석
각 권역별 수층 이화학적 특성을 파악하기 위해 주성분분석(principal componenet analysis, PCA)을 실시하였다(그림 3). 분석결과 제1주성분(PC1)과 제2주성분(PC2)의 누적 기여율은 77.2%으로 나타났다. 제1주성분과 제2주성분의 주성분 고유값(Eigen value)은 각각 8.6과 1.4로 모두 1 이상의 값을 보여 각 인자간 유의미한 상관성이 있음을 나타냈다. 주성분분석 결과 제1주성분은 전체 분산에 대해 66.3%의 기여율을 보였으며, 수층 내 수온, 용존산소 및 입자성 및 용존성 유·무기원소 요인들에 의해 구분되었다(그림 3). 제2주성분은 전체 분산에 대해 10.9%의 기여율을 보였으며, 연구정점에서 염분, 용존성 유·무기 원소, 화학 원소 비율과 수온, 용존산소, 입자성 유기탄소, 입자성 총질소 특성 간에 차별적인 상관관계가 나타났다(그림 3).
4. 토 의
4.1. 권역별 수층 이화학적 특성의 공간적 분포
수환경 내 분석된 물리화학적 요소(온도, 염분, 용존산소)들은 권역별 뚜렷한 차이를 보였다(그림 2). 그 중 염분은 육상-해양환경을 따라 가장 뚜렷한 공간적 농도 변동성(육상; 3.6±2.9, 해양; 31.46±1.8 psu)을 보이며 이는 전형적인 권역별 물 순환 흐름 체계(예; 지표유출, 담수 및 해수 혼합)를 보여주고 있다(그림 2a-c; Darnell and Soniat, 1979; Liang et al., 2023). 더욱이 위의 혼합체계에서 분석된 화학성분(용존산소, 영양염, 용존성 유기탄소, 입자성 유기탄소 및 총 질소)의 농도는 육상 수환경(R1-12)에서 뚜렷한 증가경향을 보였을 뿐 만 아니라 내부적으로도 뚜렷한 이질적(heterogeneous) 특징을 보였다(그림 2d-h). 이와 관련하여, 주성분 통계 분석결과에서 나타나는 육상권역 내 영양염(NOX, NH4, PO4, SiO2), 유기탄소 및 총 질소의 양의 상관관계(R2 > 0.4, p < 0.05; 표 2)는 육상-해양환경 간에 차별적인 유·무기 원소의 생성 기원 및 지표수 유출을 통한 이들의 이동 가능성을 반영한 것이라 판단된다(그림 3). 본 연구지역인 형산강의 경우 주변 토지이용율 형태로는 농경지, 도심지, 공업단지를 포함하고 있으며(Kim et al., 2024c), 질소 및 인 영양염의 농도가 가장 높았던 상류R4 정점은 농경지, 도심지 및 하수처리장에서 기인된 비료와 생활 하수 및 배출수 같은 인공적인 유입원과 밀접한 관계를 나타낼 수 있을 것이라 판단된다(Thayalakumaran et al., 2008; Zendehbad et al., 2019; Chen et al., 2020; Malagó and Bouraoui, 2021). 또한 하류 정점(R9, R10)에서의 상대적으로 높은 질소계 영양염의 농도는 공단지역 내 산업활동(예; 연료 연소, 공업폐수)의 영향을 고려해 볼 수 있다(Zhang et al., 2018; Hong et al., 2019; Tian et al., 2021). 비록 선행연구를 통해 보고된 연구지역 내 대기중 질소산화물(NO2)의 농도(0.01-0.03 ppm)가 수층에 비해 미비하지만 (Lee et al., 2023), 연구 지역 내 대기중 미세먼지(PM10)의 대부분(97%, 50.5-70.2 μg/m3)이 철강산업단지에서 기인하는 것으로 보고된 바 있다(Jung et al., 2010). 또한 연료 연소 중 질소 산화물의 추가적 산화를 통해 형성된 질산(HNO3)은 도로 침전을 통한 지표 유출 및 직접적인 대기 습식·건식 침적을 통해 주변 강 유역 내 혼합적 축적이 이루어질 수 있다(Walters et al., 2015; Lee et al., 2023). 최종적으로 이들 무기원소는 지표유출을 통해 해양환경으로 이동(transport) 되는 것과 관련하여, 육상권역에 가장 인접한 E1정점(NOX; 20.7 μM, NH4; 6.5 μM, PO4; 1.2 μM, SiO2; 51.9 μM)까지 영향을 받을 수 있으며 대부분 해양환경 정점(E2-E6, M1-M6)에서는 물리화학적 반응(예; 희석, 생물동화; Kamjunke et al., 2023)을 통해 낮은 농도범위(NOX; 0.2±0.2 μM, NH4; 1.3±0.4 μM, PO4; <0.03 μM, SiO2; 2.2±1.3 μM)를 보였다. 따라서 형산강에서 지표수 유출을 통해 유입되는 자연적 및 인위적 유·무기 원소들은 연안환경과 해양환경 내 자생기원 유기물의 생성과 밀접한 연관성이 있을 것이라 판단된다.
권역별 용존성 유기탄소 및 총 질소의 농도 분포는 수층 물리화학적 요인들과 유사하게 육상에서 상대적으로 높은 농도 경향을 나타냈다(그림 2f). 특히 형산강 일부정점(R4, R9, R10)에서 상대적으로 높은 영양염류의 농도와 함께 용존성 유기탄소 및 총 질소의 높은 농도범위(DOC; 3.8±2.6 mg/L, DTN; 3.4±1.2 mg/L)를 보였다(그림 2d-f). 이는 주변 농경지, 도심지, 하수처리장 및 공단지역 내 지표수 유출 및 주변 우수관 배출을 통해 인위적 기원의 용존성 유·무기 원소들의 공급 가능성을 고려해 볼 수 있다(Lee et al., 2017; Ke et al., 2020; Heredia et al., 2022). 형산강 내 분석된 용존성 유기 탄소 및 총 질소 농도의 공간적 변동을 토대로 이들 원소 비율(용존성 유기탄소/총 질소)은 상대적으로 매우 낮은 범위(1.6±0.6)를 보였다(표 1). 일반적으로 유기탄소/총 질소 비(DOC/DTN)는 육상 식생(C3, C4, >12), 수층 식물플랑크톤(6-8) 등 그 기원에 따라 특정한 값을 반영할 수 있다(Milliman et al., 1984; Lobbes et al., 2000; Lee et al., 2020). 특히 육상환경 내 인간활동 기원의 과잉 질소원 유입 영향은 DOC/DTN 비를 두드러지게 낮출 수 있어 인공적 유입원의 잠재적 영향 가능성 파악에 유용한 지시자로 활용되고 있다(Lutz et al., 2011; Yates et al., 2019; Wymore et al., 2021). 특히 형산강 내 R4, R11정점의 경우, 이들 원소비율 특성을 통해 증가된 인위적 기원의 유·무기 질소 유입 가능성이 잠재적으로 반영된 결과라 판단된다. 한편 영일만-동해에서는 상대적으로 낮은 용존 유기탄소 및 총 질소 농도범위(DOC; 1.0±0.2 mg/L, DTN; 0.2±0.1 mg/L)를 보였으며, 이들 권역에서 상대적으로 높은 원소비율(7.0±1.4)을 보였다(그림 2f; 표 1). 일반적으로 해양환경의 경우, 수층 내 상대적으로 낮은 질소농도로 인해 이들 원소비율은 6-8 범위를 보일 수 있으며(Milliman et al., 1984; Lobbes et al., 2000; Lee et al., 2020), 이는 자생기원의 플랑크톤의 생성 및 분해 영향 가능성을 잠재적으로 반영할 수 있다(Song et al., 2019; Zhang et al., 2023). 특히 일차생산자의 활동 및 분해를 통해 용존 유기탄소는 지속적으로 생성되지만, 질소계 영양염의 소비와 상대적으로 빠른 용존 유기질소(dissolved organic nitrogen, DON)의 분해는 DTN 농도를 낮추어 DOC/DTN 비를 상대적으로 증가시킬 수 있다(Garber, 1984; Hopkinson et al., 2002). 따라서 유기물 생성 기작과 관련하여 해양권역 내 상대적으로 높은 원소비율은 육상으로 공급된 영양염이 동화(assimilation)되어 수층 일차생산 과정에 잠재적 중요한 역할을 담당할 것이라 판단된다.
입자성 유기탄소 및 총 질소의 경우 상대적으로 낮은 농도 범위를 보임에도 불구하고 권역별 차별적 공간 변동성을 보였다(형산강 POC; 0.6±0.2 mg/L, PN; 0.2±0.05 mg/L, POC/PN; 4.3±1.6, 영일만 POC; 0.6±0.3 mg/L, PN; 0.1±0.02 mg/L, POC/PN; 8.9±5.0, 동해 POC; 0.1±0.01 mg/L, PN; <0.1 mg/L, POC/PN; 13.3±8.2; 그림 2g-h). 형산강의 경우 농경지, 도심지·공단지에서 인간기원 질소원(비료, 오폐수 및 연료 연소)의 지표 유출이 증가될 수 있는 점을 고려했을 때(Jung et al., 2021; Seo, 2022; Kim et al., 2024c), 인접한 강 유역 정점(R4-R12)에서 입자성 유기원소들이 상대적으로 높은 농도가 나타날 수 있을 것이라 판단된다. 영일만의 경우 입자성 유기탄소 및 총 질소의 농도(POC; 0.6±0.3 mg/L, PN; <0.1 mg/L)는 상대적으로 높으며 이들의 원소비율(POC/PN)은 육상에 비해 상대적으로 높은(8.9±5.0) 경향을 보였다(그림 2g; 표 1). POC/PN비는 용존성 유기원소 비율과 마찬가지로 입자성 유기물의 기원(육상 식생; >20, 식물 플랑크톤; 5-8) 추적 연구에 활발히 활용되어 왔다(Hedges et al., 1986; Chen et al., 2021; Liang et al., 2023). 이와 관련하여 해양권역 내 상대적으로 증가된 이들의 원소비는 수층 내 자생기원 유기물의 우점성을 잠재적으로 반영할 수 있으며, 육상권역과는 차별적인 특징을 보일 것이라 판단된다. 특히 용존성 및 입자성 유·무기원소의 연결성과 관련하여, 육상환경에서 공급되는 과잉의 영양염 및 용존성 유기물의 유입은 연안 환경 내 식물플랑크톤의 생합성(탄소고정) 과정에 조절요인이 될 수 있다(Boyer et al., 2006; Cloern et al., 2013; Paczkowska et al., 2020). 이에 따라 형산강으로부터 공급되는 용존성 유·무기원소의 증가는 영일만 수층 내 POC/PN비를 증가시키는 주된 요인이 될 수 있을 것이며, 입자성 유기원소 내 자생기원 유기물 유입 증거가 반영된 것이라 판단된다. 이후 동해에서는 수층 내 물리적(해수 혼합 및 희석), 화학적(유기물 생분해) 반응에 따라 입자성 유기탄소, 총 질소의 농도가 급격하게 감소하는 경향을 나타냈으나, 상대적으로 일정한 농도(POC 및 PN; <0.1 mg/L)를 유지하였다. 이는 연안에서의 활발한 일차생산 활동으로 인해 해양으로 전달되는 수층 영양염류가 고갈되어 상대적으로 빈영양 상태(oligotrophic)를 반영할 수 있으며, 수층 내 일차생산 활동이 약화되는 주된 요인이 될 수 있을 것이라 판단된다. 이러한 측면에서 동해에서 상대적으로 가장 높은(13.3±8.2) POC/PN 비는 해양 수층 내 외부 기작(대기 침적)으로부터 발생될 수 있는 유기물 생성 가능성을 잠재적으로 반영할 것이라 판단된다.
4.2. 권역별 유기원소 내 동위원소 분포 특성
권역별 입자성 유기물의 명확한 기원 판별을 위해 탄소, 질소 안정동위원소 비(δ13C, δ15N)의 공간적 변동성과 문헌상에 보고된 잠재적 유입원(육상 C3식물, 수층 플랑크톤, 비료, 오폐수 및 연료 연소)들의 값과 비교하였다(그림 4; 표 1). 육상의 경우 탄소, 질소 안정동위원소 비는 뚜렷한 공간적 변동성(δ13CPOC; -30.1 ‒ -21.0‰, δ15NPN; 0.1 ‒ 9.5‰)을 나타냈으며, 잠재적 유입원의 혼합 영향이 반영된 것이라 판단된다. 일반적으로 육상 내 산림 식생 및 토양, 농경지의 비료, 도심지 및 공단의 오폐수 유출, 연료 연소와 같은 인위적 유입원들은 지표 유출이나 배출수를 통해 강·하천 유역으로 유입될 수 있다(Nriagu and Pacyna, 1988; Fernandes et al., 2016). 특히 농경지, 도심지, 공단에서 방출되는 비점오염원의 경우, 지표수 유출과 함께 수로 및 우수관과 같은 다양한 경로를 통해 공간적으로 넓게 유출될 수 있다(Cachada et al., 2012; Gómez et al., 2012). 이와 같은 환경에서 비료 및 연료 연소와 같은 인공적인 유입원들은 촉매제, 열 처리와 같은 화학적인 처리 과정 중 동위원소 분별작용을 거치게 된다(Walters et al., 2015; Sáez et al., 2020). 따라서 인위적 유입원들은 각각의 특징적인 안정동위원소 비 범위(비료; δ13C; -29.2 ‒ -23.5‰, δ15N; -0.3±8.8‰, 오폐수; δ13C; -26.0 ‒ -22.0‰, δ15N; 7 ‒ 22‰)를 나타낼 수 있다(Mayer et al., 2002; Barros et al., 2010; Gao et al., 2012; Fernandes et al., 2016). 한편 자연적 유기물(식생 및 식물플랑크톤)의 경우 대기 중 이산화탄소 고정 및 영양염을 동화하는 과정에서 차별적 동위원소 분별작용(C3 식물 δ13C; -30 ‒ -23‰, δ15N; -5 ‒ 5‰, 해양 식물플랑크톤 δ13C; -22 ‒ -18‰, δ15N; 3 ‒ 12‰)을 거칠 수 있다(Meyers, 1994; Thornton and McManus, 1994; Torres et al., 2012; Huang et al., 2020). 이런 점을 고려했을 때, 형산강 정점 내 입자성 유기원소의 안정동위원소 비가 공간적으로 큰 변동성을 보이는 것은 자연적·인위적 유입원들의 차별적인 공급 영향을 반영할 수 있으며, R1-R4 정점과 R9-R10 정점에서 각각 가장 우세한 식생 및 비료와 오폐수 및 연료 연소 유입 증거를 분명히 나타낸다.
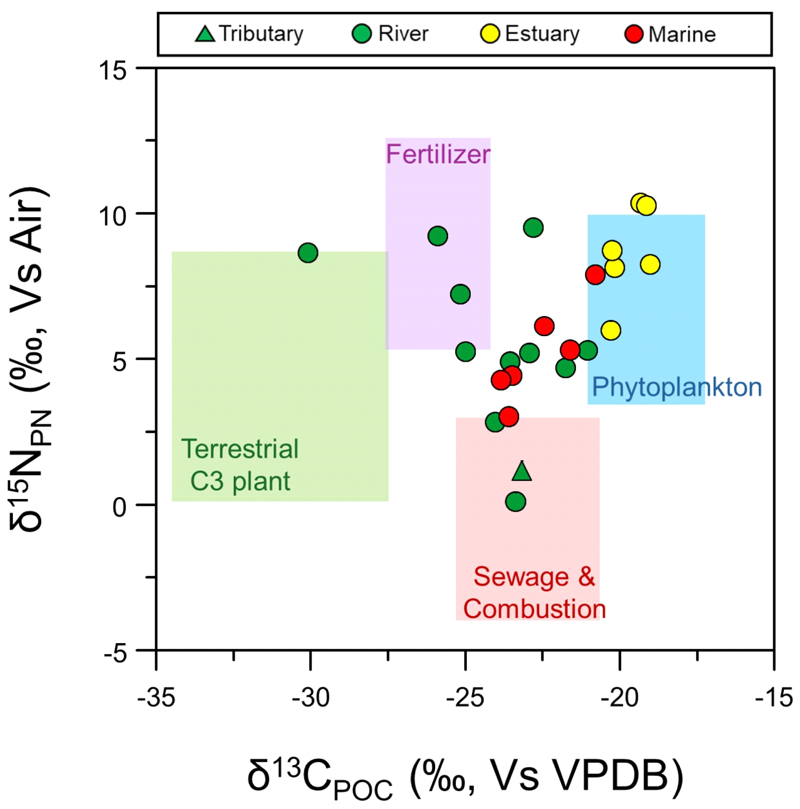
Stable carbon and nitrogen isotopic compositions of POM in study sites. Colorful boxes indicated reference source end-members (terrestrial C3 plant, marine plankton, fertilizer, and sewage & combustion).
연안-해양 환경에서의 수층 입자성 유기물의 안정동위원소비는 육상과 달리 일정한 공간적 변화 경향을 나타냈다(그림 2g-h). 영일만 정점(E1 및 E2)에서 동위원소 비 조성이 점차 무거워진 (δ13CPOC; -19.8±0.7‰, δ15NPN; 8.2±3.1‰) 이후 상대적으로 일정한 범위(δ13CPOC; -19.6±0.7‰, δ15NPN; 8.9±1.0‰)를 나타냈다. 이러한 안정동위원소 특성은 육상과 달리 수층 내 우점적 단일 유기공급원 우세가 반영된 결과라 판단된다. 특히 영양염 및 POC/PN 비와 비교했을 때, 수층 내 증가된 탄소고정으로부터 기인된 자생기원 유기물 기원이 우점적으로 존재할 수 것이라 판단된다. 한편, 동해의 경우 상대적으로 증가된 POC/PN비와 함께 δ13CPOC 및 δ15NPN 값의 상대적으로 가벼워지는 경향(δ13CPOC; -22.6±1.2‰, δ15NPN; 5.2±1.7‰)을 보였다. 이는 수층 내 외부기원 유기물의 차별적 공급 가능성을 나타낼 수 있으며, 대기 중 질소(δ15NAir; ~ 0‰)고정 영향과 밀접한 연관성이 있을 것이라 판단된다(Simoneit, 2006; Kang et al., 2017). 특히 영일만 내 육상 지표수 유출을 통해 유입된 질소 동화과정과는 달리, 동해의 경우 주변 화석연료 연소와 같은 인위적 대기 질소 공급원 유입을 통해 수층 질소고정이 우세하게 진행될 수 있을 것이라 판단된다. 연료 연소의 부산물은 대기로 방출되고, 상대적으로 장거리 이동 특성을 고려했을 때(Huang et al., 2018; Rastogi et al., 2020), 빈영양 상태의 동해 수층에서 대기 중 질소 고정을 통해 일차생산 활동을 유지할 수 있을 것이라 판단된다. 따라서 영일만-동해에서 자생기원 유기물 생성과 관련된 공간적 안정동위원소 변화는 서로 다른 육상기인 질소기원 유입 특성에 따라 차별적 동위원소 분별작용이 발생될 수 있으며, 이로 인해 해양 입자성 유기원소 내 서로 다른 안정동위원소 범위를 보일 것으로 판단된다.
4.3. 권역별 유기원소 기여도 산정
육상 토지이용형태로부터 유입되는 인간활동 기원 요소와 수층 내 자생기원 유기물 생성 영향을 고려하여 육상 C3식물, 비료, 오폐수 및 연료 연소, 식물플랑크톤을 동위원소 대표값(end-member)으로 설정하였다. 이를 통해 권역별 기여도 산정 결과, 형산강은 오폐수·연료 연소(34±18%), 식물플랑크톤(28±14%), 육상 C3식물(22±17%), 비료(16±7%)의 순으로 각각 정점간 차별적 기여도를 나타냈다(그림 5). 특히 가장 우세한 기여를 보인 오폐수·연료 연소 유입원의 영향은 도심지와 공단이 밀집해 있는 형산강 하류(R6-R10) 부분에서 상대적으로 높은(48±16%) 기여를 나타냈다. 하류 지역의 경우 도심지 및 철강산업단지가 밀집되어 있는 지역으로, 앞서 언급한 바와 같이 철강산업단지에서 대부분 유래되는 것으로 보고된 다량의 대기중 미세먼지(PM10; 14.0-89 μg/m3) 내 질소(0.01-0.03 ppm)와 탄소(0.1-1.0 ppm)는 직접적인 대기침적 및 도로 침적 후 지표 유출을 통해 수 환경으로 유입될 수 있다 (Jung et al., 2010; Lee et al., 2023). 또한 형산강 내 상대적으로 높은 플랑크톤의 기여는 다양한 토지이용형태로부터 지속적인 공급되는 영양염 성분들의 영향이 일부 반영된 결과라 판단된다. 반면 식생이나 비료의 경우, 토양 내 단단히 결속되어 대기연소 기인 유기원소 유입 기여에 비해 상대적으로 수층으로의 전달이 제한적인 것으로 판단된다(Brahim and Ibrahim, 2018). 영일만의 경우 식물플랑크톤(81±7%) 기여가 그외 유입원(오폐수·연료 연소; 9±4%, 비료; 6±2%, 육상 C3식물; 4±1%)에 비해 월등히 높은 수준을 나타냈다. 이 결과는 형산강으로부터 공급되는 과잉의 영양염류 배출이 영일만 내 일차생산 기작을 급격하게 증가시킬 수 있음을 의미한다. 한편 동해의 경우 식물플랑크톤(39±17%)과 오폐수·연료 연소(36±11%)가 우점적 기여를 나타냈다. 이와 같은 결과는 해양 내 수층 일차 생산기작과 더불어, 입자성 유기물 내 대기를 통한 인위적 질소 기원 유입 가능성을 반영한 것으로 판단된다. 실제로 대기 중 질소 침적 발생으로 인해 반영될 수 있는 입자성 유기질소의 동위원소 변동은 시공간적 특성에 따라 범위를 보일 수 있으며(Rastogi et al., 2020; Xie et al., 2023), 본 연구지역 내 나타난 입자성 유기원소의 동위원소 결과를 통해 이들의 유입 가능성을 분명히 반영할 수 있을 것으로 판단된다. 추후 이들 원소의 안정동위원소 비가 수층 플랑크톤의 에너지원 변화와 유기물 재광물화 영향으로 변동될 가능성을 고려했을 때(Altabet, 2006; Mompeán et al., 2016), 동해 수괴별(동한난류 및 북한한류) 다원소 동위원소 데이터베이스 확보 및 수층 재광물로 기인된 질소성분의 안정동위원소 대표값 설정에 관한 추가 연구가 필요할 것이라 판단된다.
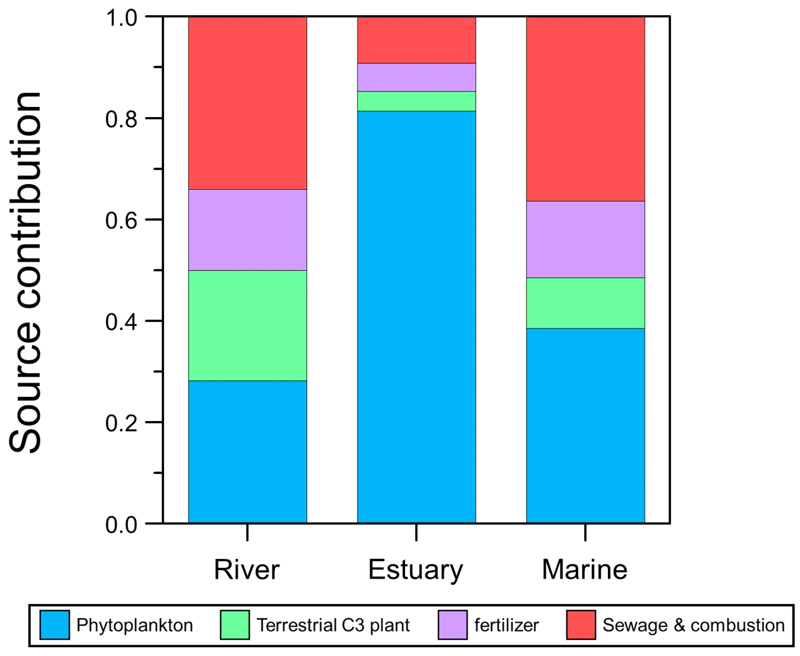
Relative contribution of natural (phytoplankton and terrestrial C3 plant) and anthropogenic (fertilizer and sewage & combustion) sources. Each POM source denoted by different color.
권역별 수층 물리화학적 요소들의 공간적 변화는 육상-연안-해양권의 밀접한 연결성을 포함할 수 있으며 수층 영양염류 및 유기물 순환에 중요한 인자로 고려될 수 있다. 형산강 내 발생되는 인위적 기원 유·무기원소의 방출은 영일만 내 생물대사작용(탄소고정)에 중요한 요소로 작용될 수 있으며, 동해의 경우 대기 침적을 통해 고갈된 질소원을 추가적으로 공급받을 수 있는 가능성을 확인하였다. 특히 본 연구에서 수행한 입자성 유기원소의 탄소, 질소 안정동위원소 비 변화는 권역별 유기물의 유입원(육상 식생, 비료, 오폐수·연료 연소, 식물플랑크톤) 변동 및 수층 물질 순환(질소고정 및 동화) 주요 기작을 파악하는데 유용한 정보를 제공하며, 안정동위원소 대표값을 활용하여 각 권역별 자연적·인위적 유기원소의 기원 특성을 정량적으로 파악할 수 있었다. 위의 안정동위원소 접근법은 육상-연안-해양으로 순환되는 유·무기 원소의 시공간적 변동성이 커지는 조건에서 인위적 기원 유·무기 원소 추적 및 수생태계 영향 파악을 위한 중요한 자료로서 활용될 수 있을 것이라 판단된다. 또한 현재 각 권역별 진행되는 국가모니터링 사업과 관련하여, 수층 유·무기 안정동위원소의 추가활용은 정밀한 육상기인 오염원 추적 및 수환경 물질순환 변동성 진단에 유용하게 적용될 수 있을 것이라 판단된다. 특히 기후변화 및 해류 흐름의 영향이 변동되는 조건에서 동해 해역 내 증가된 일차생산성과 인간활동 증가에 관한 상관성을 파악하는 데 중요한 정보를 제공할 수 있을 것이다. 이와 더불어 환경적 이슈(부영양화, 조류 대발생, 빈산소 수괴 형성) 해결을 위해 구체적인 과학적 증거 제공을 할 수 있을 것이라 판단된다. 추후 용존성 유기탄소 및 질소계 영양염의 안정동위원소 분석과 관련하여, 수층 내 유·무기 원소의 거동 특성(일차생산, 유기물 분해, 영양염 재광물화 등)을 종합적으로 파악할 수 있으며 이를 통해 육상-연안-해양 환경의 연결성을 파악하는 유용한 지표로서 활용될 수 있을 것이라 판단된다.
5. 요약 및 결론
본 연구에서 권역별 수층 물리화학적 인자들과 입자성 유기물의 안정동위원소 비 변동의 비교를 통해 육상-연안-해양 환경의 연결성 내 유·무기원소의 거동을 파악하고자 하였다. 육상권역의 농경지, 도심지 및 공단 내 비료(16±7%), 오폐수 및 연료 연소(34±18%)와 같은 인공적 활동은 수환경으로 다량의 유·무기 원소를 공급하는 주요 유입원으로 파악되었다. 이는 인접한 강·하천 및 연안에 과잉의 영양염류를 공급하여 수환경 내 자생기원(phytoplankton, 육상; 28±14%, 연안; 81±7%) 입자성 유기탄소의 증가를 유발하였다. 또한 해양에서는 수층을 통한 유·무기 원소의 공급은 감소하였으나, 대기를 통한 인공적(오폐수 및 연료 연소; 36±11%) 유입원의 영향이 해양 내에서 증가할 수 있는 것으로 파악됐다. 안정동위원소 비를 활용한 본 연구 결과 육상 내 과잉의 인간활동은 연안 및 해양환경 내 유·무기 원소 순환의 연결성에 있어 극심한 변화를 유발할 수 있는 중요한 요인으로 판단된다. 향후 기후변화(태풍, 온도 상승 등)와 급격하게 증가하는 인간활동의 영향을 정밀·정확하게 진단하기 위해서는 수층 및 대기 유입 경로를 통해 유입될 수 있는 육상 및 연안-해양 환경 내 다양한 유·무기 원소 유입원에 대한 안정동위원소 데이터베이스 구축이 요구된다. 환경 모니터링 연구를 통해 구축될 안정동위원소 데이터베이스를 활용하여 수환경 내 유·무기원소 거동을 지도화한다면 미래 기후변화와 인간활동에 의한 환경 변화 예측 모델 구축에 중요한 기초자료를 제공할 것이라 판단된다.
Acknowledgments
이 논문은 2023년도 정부(교육부)의 재원으로 한국연구재단의 G-램프(LAMP) 사업 지원을 받아 수행된 연구임(No. RS-2023-00301702). 부분적으로 부경대학교 신임교수 학술연구비(202303780001)에 의하여 연구되었습니다. 시료채집에 도움을 주신 부경대학교 나라호 승조원분들께 감사드립니다.
References
-
Aichner, B., Rittweg, T., Schumann, R., Dahlke, S., Duggen, S. and Dubbert, D., 2022, Spatial and temporal dynamics of water isotopes in the riverine-marine mixing zone along the German Baltic Sea coast. Hydrological Processes, 36, e14686.
[https://doi.org/10.1002/hyp.14686]
-
Altabet, M.A., 2006, Isotopic Tracers of the Marine Nitrogen Cycle: Present and Past. In Volkman, J.K. (Ed.), Marine Organic Matter: Biomarkers, Isotopes and DNA, 251-293.
[https://doi.org/10.1007/698_2_008]
-
Apitz, S.E., Elliott, M., Fountain, M. and Galloway, T.S., 2006, European environmental management: Moving to an ecosystem approach. Integrated Environmental Assessment and Management, Environ Assess Manag, 2, 80-85.
[https://doi.org/10.1002/ieam.5630020114]
-
Aufdenkampe, A.K., Mayorga, E., Raymond, P.A., Melack, J.M., Doney, S.C., Alin, S.R., Aalto, R.E. and Yoo, K., 2011, Riverine coupling of biogeochemical cycles between land, oceans, and atmosphere. Frontiers in Ecology and the Environment, 9, 53-60.
[https://doi.org/10.1890/100014]
-
Barros, G.V., Martinelli, L.A., Novais, T.M.O., Ometto, J.P.H.B. and Zuppi, G.M., 2010, Stable isotopes of bulk organic matter to trace carbon and nitrogen dynamics in a estuarine ecosystem in Babitonga Bay (Santa Catarina, Brazil). Science of the Total Environment, 408, 2226-2232.
[https://doi.org/10.1016/j.scitotenv.2010.01.060]
-
Bauer, J.E., Cai, W.J., Raymond, P.A., Bianchi, T.S., Hopkinson, C.S. and Regnier, P.A.G., 2013, The changing carbon cycle of the coastal ocean. Nature, 504, 61-70.
[https://doi.org/10.1038/nature12857]
-
Borja, A. and Dauer, D.M., 2008, Assessing the environmental quality status in estuarine and coastal systems: Comparing methodologies and indices. Ecological Indicators, 8, 331-337.
[https://doi.org/10.1016/j.ecolind.2007.05.004]
-
Boyer, J.N., Dailey, S.K., Gibson, P.J., Rogers, M.T. and Mir-Gonzalez, D., 2006, The role of dissolved organic matter bioavailability in promoting phytoplankton blooms in Florida Bay. Hydrobiologia, 569, 71-85.
[https://doi.org/10.1007/s10750-006-0123-2]
-
Brahim, N. and Ibrahim, H., 2018, Effect of Land Use on Organic Carbon Distribution in a North African Region: Tunisia Case Study. Soil Management and Climate Change: Effects on Organic Carbon, Nitrogen Dynamics, and Greenhouse Gas Emissions, 15-24.
[https://doi.org/10.1016/B978-0-12-812128-3.00002-1]
-
Branco, P., Egas, M., Elser, J.J. and Huisman, J., 2018, Eco-Evolutionary Dynamics of Ecological Stoichiometry in Plankton Communities. The American Naturalist, 192, E1-E20.
[https://doi.org/10.1086/697472]
-
Cachada, A., Pereira, M.E., Ferreira da Silva, E. and Duarte, A.C., 2012, Sources of potentially toxic elements and organic pollutants in an urban area subjected to an industrial impact. Environmental Monitoring and Assessment, 184, 15-32.
[https://doi.org/10.1007/s10661-011-1943-8]
-
Chen, X., Jiang, C., Zheng, L., Dong, X., Chen, Y. and Li, C., 2020, Identification of nitrate sources and transformations in basin using dual isotopes and hydrochemistry combined with a Bayesian mixing model: Application in a typical mining city. Environmental Pollution, 267, 115651.
[https://doi.org/10.1016/j.envpol.2020.115651]
-
Chen, F., Lu, X., Song, Z., Huang, C., Jin, G., Chen, C., Zhou, X., Lao, Q. and Zhu, Q., 2021, Coastal currents regulate the distribution of the particulate organic matter in western Guangdong offshore waters as evidenced by carbon and nitrogen isotopes. Marine Pollution Bulletin, 172, 112856.
[https://doi.org/10.1016/j.marpolbul.2021.112856]
-
Choi, J.D., Lim, K.J. and Won, C.H., 2013, Catchment water quality management planning for sustainable development of tidal areas. Irrigation and Drainage, 62, 18-23.
[https://doi.org/10.1002/ird.1778]
-
Cloern, J.E., Foster, S.Q. and Kleckner, A.E., 2013, Review: phytoplankton primary production in the world’s estuarine-coastal ecosystems. Biogeosciences Discuss, 10, 17725-17783.
[https://doi.org/10.5194/bgd-10-17725-2013]
-
Darnell, R.M. and Soniat, T.M., 1979, The Estuary/Continental Shelf as an Interactive System. Ecological Processes in Coastal and Marine Systems, 10, 487-525.
[https://doi.org/10.1007/978-1-4615-9146-7_21]
-
Derrien, M., Kim, M.S., Ock, G.Y., Hong, S.J., Cho, J.W., Shin, K.H. and Hur, J., 2018, Estimation of different source contributions to sediment organic matter in an agricultural-forested watershed using end member mixing analyses based on stable isotope ratios and fluorescence spectroscopy. Science of the Total Environment, 618, 569-578.
[https://doi.org/10.1016/j.scitotenv.2017.11.067]
-
Dubinsky, Z. and Berman-Frank, I., 2001, Uncoupling primary production from population growth in photosynthesizing organisms in aquatic ecosystems. Aquatic Sciences, 63, 4-17.
[https://doi.org/10.1007/PL00001343]
-
Falkowski, P., Scholes, R.J., Boyle, E., Canadell, J., Canfield, D., Elser, J., Gruber, N., Hibbard, K., Högberg, P., Linder, S., Mackenzie, F.T., Moore III, B., Pedersen, T., Rosenthal, Y., Seitzinger, S., Smetacek, V. and Steffen, W., 2000, The Global Carbon Cycle: A Test of Our Knowledge of Earth as a System. Science, 290, 291-296.
[https://doi.org/10.1126/science.290.5490.291]
-
Fernandes, A.M., Hissler, C., da Conceição, F.T., Junior, E.P.S. and Mortatti, J., 2016, Combined analysis of trace elements and isotopic composition of particulate organic matter in suspended sediment to assess their origin and flux in a tropical disturbed watershed. Environmental Pollution, 218, 844-854.
[https://doi.org/10.1016/j.envpol.2016.08.008]
-
Frigstad, H., Andersen, T., Hessen, D.O., Naustvoll, L.J., Johnsen, T.M. and Bellerby, R.G.J., 2011, Seasonal variation in marine C:N:P stoichiometry: can the composition of seston explain stable Redfield ratios?. Biogeosciences, 8, 2917-2933.
[https://doi.org/10.5194/bg-8-2917-2011]
-
Galloway, J.N., Schlesinger, W.H., Levy II, H., Michaels, A. and Schnoor, J.L., 1995, Nitrogen fixation: Anthropogenic enhancement-environmental response. Global Biogeochemical Cycles, 9, 235-252.
[https://doi.org/10.1029/95GB00158]
-
Gao, X., Yang, Y. and Wang, C., 2012, Geochemistry of organic carbon and nitrogen in surface sediments of coastal Bohai Bay inferred from their ratios and stable isotopic signatures. Marine Pollution Bulletin, 64, 1148-1155.
[https://doi.org/10.1016/j.marpolbul.2012.03.028]
-
Garber, J.H., 1984, Laboratory study of nitrogen and phosphorus remineralization during the decomposition of coastal plankton and seston. Estuarine, Coastal and Shelf Science, 18, 685-702.
[https://doi.org/10.1016/0272-7714(84)90039-8]
-
Gómez, M.J., Herrera, S., Solé, D., García-Calvo, E. and Fernández-Alba, A.R., 2012, Spatio-temporal evaluation of organic contaminants and their transformation products along a river basin affected by urban, agricultural and industrial pollution. Science of The Total Environment, 420, 134-145.
[https://doi.org/10.1016/j.scitotenv.2012.01.029]
-
Hedges, J.I., Clark, W.A., Quay, P.D., Richey, J.E., Devol, A.H. and Santos, M., 1986, Compositions and fluxes of particulate organic material in the Amazon River. Limnology and Oceanography, 31, 717-738.
[https://doi.org/10.4319/lo.1986.31.4.0717]
-
Hedges, J.I., Keil, R.G. and Benner, R., 1997, What happens to terrestrial organic matter in the ocean?. Organic Geochemistry, 27, 195-212.
[https://doi.org/10.1016/S0146-6380(97)00066-1]
-
Heredia, C., Guédron, S., Point, D., Perrot, V., Campillo, S., Verin, C., Espinoza, M.E., Fernandez, P., Duwig, C. and Achá, D., 2022, Anthropogenic eutrophication of Lake Titicaca (Bolivia) revealed by carbon and nitrogen stable isotopes fingerprinting. Science of The Total Environment, 845, 157286.
[https://doi.org/10.1016/j.scitotenv.2022.157286]
-
Hong, S., Lee, Y., Yoon, S.J., Lee, J., Kang, S., Won, E.J., Hur, J., Kim, J.S. and Shin, K.H., 2019, Carbon and nitrogen stable isotope signatures linked to anthropogenic toxic substances pollution in a highly industrialized area of South Korea. Marine Pollution Bulletin, 144, 152-159.
[https://doi.org/10.1016/j.marpolbul.2019.05.006]
-
Hopkinson, C.S., Vallino, J.J. and Nolin, A., 2002, Decomposition of dissolved organic matter from the continental margin. Deep Sea Research Part II: Topical Studies in Oceanography, 49, 4461-4478.
[https://doi.org/10.1016/S0967-0645(02)00125-X]
-
Huang, C., Chen, F., Zhang, S., Chen, C., Meng, Y., Zhu, Q. and Song, Z., 2020, Carbon and nitrogen isotopic composition of particulate organic matter in the Pearl River Estuary and the adjacent shelf. Estuarine, Coastal and Shelf Science, 246, 107003.
[https://doi.org/10.1016/j.ecss.2020.107003]
-
Huang, H.J., Hwang, D.W., Lee, G.R., Kim, H.C. and Kwon, J.N., 2018, Distributions of Organic Matter and Heavy Metals in the Surface Sediment of Jaran Bay, Korea. Journal of the Korean Society of Marine Environment & Safety, 24, 78-91 (in Korean with English abstract).
[https://doi.org/10.7837/kosomes.2018.24.1.078]
-
Jung, J.H., Choi, W.J., Leem, H.H. and Shon, B.H., 2010, Health and Environmental Risk Assessment of Pollutants in Pohang, Occurrence Characteristics and Concentration Distribution of PM10. Journal of the Korea Academic-Industrial cooperation Society, 11, 2719-2726 (in Korean with English abstract).
[https://doi.org/10.5762/KAIS.2010.11.7.2719]
-
Jung, S., Kwon, S.Y., Hong, Y., Yin, R. and Motta, L.C., 2021, Isotope investigation of mercury sources in a creek impacted by multiple anthropogenic activities. Chemosphere, 282, 130947.
[https://doi.org/10.1016/j.chemosphere.2021.130947]
-
Kamjunke, N., Brix, H., Flöser, G., Bussmann, I., Schütze, C., Achterberg, E.P., Ködel, U., Fischer, P., Rewrie, L., Sanders, T., Borchardt, D. and Weitere, M., 2023, Large-scale nutrient and carbon dynamics along the river-estuary-ocean continuum. Science of the Total Environment, 890, 164421.
[https://doi.org/10.1016/j.scitotenv.2023.164421]
-
Kang, M., Yang, F., Ren, H., Zhao, W., Zhao, Y., Li, L., Yan, Y., Zhang, Y., Lai, S., Zhang, Y., Yang, Y., Wang, Z., Sun, Y. and Fu, P., 2017, Influence of continental organic aerosols to the marine atmosphere over the East China Sea: Insights from lipids, PAHs and phthalates. Science of the Total Environment, 607-608, 339-350.
[https://doi.org/10.1016/j.scitotenv.2017.06.214]
-
Ke, Z., Chen, D., Liu, J. and Tan, Y., 2020, The effects of anthropogenic nutrient inputs on stable carbon and nitrogen isotopes in suspended particulate organic matter in Jiaozhou Bay, China. Continental Shelf Research, 208, 104244.
[https://doi.org/10.1016/j.csr.2020.104244]
-
Kim, C.S., Kim, S.H., Lee, W.C. and Lee, D.H., 2022, Spatial variability of water quality and sedimentary organic matter during winter season in coastal aquaculture zone of Korea. Marine Pollution Bulletin, 182, 113991.
[https://doi.org/10.1016/j.marpolbul.2022.113991]
- Kim, H.S., Kim, T.H., Park, T.G. and Ki, J.S., 2024a, Identification of Environmental and Phytoplankton Fluctuation Patterns in Jinhae Bay, South Sea: Focusing on Harmful Plankton from 2017 to 2022. Ocean and Polar Research, 46, 43-53.
-
Kim, S.H., Kim, M.S., Lee, D.H. and Shin, K.H., 2024b, Impact of typhoons on anthropogenic nitrogen sources in Lake Sihwa, South Korea. Marine Pollution Bulletin, 202, 116324.
[https://doi.org/10.1016/j.marpolbul.2024.116324]
-
Kim, Y.G., Kwon, S.Y., Washburn, S.J., Hong, Y., Han, S.H., Lee, M. and Park, J.H., 2024c, Environmental forensics approach to source investigation in a mercury contaminated river: Insights from mercury stable isotopes. Journal of Hazardous Materials, 461, 132559.
[https://doi.org/10.1016/j.jhazmat.2023.132559]
-
Lee, H., Kwon, S.Y., Kam, J., Lee, K., Fu, X., Cho, I.G., and Choi, S.D., 2023, Isotopic investigation of sources and processes affecting gaseous and particulate bound mercury in the east coast, South Korea. Science of the Total Environment, 891, 164404.
[https://doi.org/10.1016/j.scitotenv.2023.164404]
-
Lee, H.S., Shim, J.Y., Shin, W.J., Choi, S.H., Bong, Y.S. and Lee, K.S., 2021, Dietary homogenization and spatial distributions of carbon, nitrogen, and sulfur isotope ratios in human hair in South Korea. PLOS ONE, 16, e0256404.
[https://doi.org/10.1371/journal.pone.0256404]
-
Lee, S.A., Kim, T.H. and Kim, G., 2020, Tracing terrestrial versus marine sources of dissolved organic carbon in a coastal bay using stable carbon isotopes. Biogeosciences, 17, 135-144.
[https://doi.org/10.5194/bg-17-135-2020]
-
Lee, Y., Hong, S., Kim, M.S., Kim, D., Choi, B.H., Hur, J., Kim, J.S. and Shin, K.H., 2017, Identification of sources and seasonal variability of organic matter in Lake Sihwa and surrounding inland creeks, South Korea. Chemosphere, 177, 109-119.
[https://doi.org/10.1016/j.chemosphere.2017.02.148]
-
Liang, S., Li, S., Guo, J., Yang, Y., Xu, Z., Zhang, M., Li, H., Yu, X., Ma, H. and Wang, X., 2023, Source, composition, and reactivity of particulate organic matter along the Changjiang Estuary salinity gradient and adjacent sea. Marine Chemistry, 252, 104245.
[https://doi.org/10.1016/j.marchem.2023.104245]
-
Liu, Q., Liang, Y., Cai, W.J., Wang, K., Wang, J. and Yin, K., 2020, Changing riverine organic C:N ratios along the Pearl River: Implications for estuarine and coastal carbon cycles. Science of the Total Environment, 709, 136052.
[https://doi.org/10.1016/j.scitotenv.2019.136052]
-
Lobbes, J.M., Fitznar, H.P. and Kattner, G., 2000, Biogeochemical characteristics of dissolved and particulate organic matter in Russian rivers entering the Arctic Ocean. Geochimica et Cosmochimica Acta, 64, 2973-2983.
[https://doi.org/10.1016/S0016-7037(00)00409-9]
-
Lutz, B.D., Bernhardt, E.S., Roberts, B.J. and Mulholland, P.J., 2011, Examining the coupling of carbon and nitrogen cycles in Appalachian streams: The role of dissolved organic nitrogen. Ecology, 92, 720-732.
[https://doi.org/10.1890/10-0899.1]
-
Malagó, A. and Bouraoui, F., 2021, Global anthropogenic and natural nutrient fluxes: from local to planetary assessments. Environmental Research Letters, 16, 054074.
[https://doi.org/10.1088/1748-9326/abe95f]
-
Mayer, B., Boyer, E.W., Goodale, C., Jaworski, N.A., Van Breemen, N., Howart, R.W., Seitzinger, S., Billen, G., Lajtha, K., Nadelhoffer, K., Vam Dam, D., Hetling, L.J., Nosal, M. and Paustian, K., 2002, Sources of nitrate in rivers draining sixteen watersheds in the northeastern U.S.: isotopic constraints. Biogeochemistry 57, 171-197.
[https://doi.org/10.1023/A:1015744002496]
-
McGovern, M., Poste, A.E., Oug, E., Renaud, P.E. and Trannum, H.C., 2020, Riverine impacts on benthic biodiversity and functional traits: A comparison of two sub-Arctic fjords. Estuarine, Coastal and Shelf Science, 240, 106774.
[https://doi.org/10.1016/j.ecss.2020.106774]
-
Meyers, P.A., 1994, Preservation of elemental and isotopic source identification of sedimentary organic matter. Chemical Geology, 114, 289-302.
[https://doi.org/10.1016/0009-2541(94)90059-0]
-
Mie, A., Novak, V., Franko, M.A., Bügel, S.G. and Laursen, K.H., 2022, Fertilizer Type Affects Stable Isotope Ratios of Nitrogen in Human Blood Plasma-Results from Two-Year Controlled Agricultural Field Trials and a Randomized Crossover Dietary Intervention Study. Journal of Agricultural and Food Chemistry, 70, 3391-3399.
[https://doi.org/10.1021/acs.jafc.1c04418]
-
Milliman, J.D., Hsueh, Y., Hu, D.X., Pashinski, D.J., Shen, H., Yang, Z. and Hacker, P., 1984, Tidal phase control of sediment discharge from the Yangtze River. Estuarine, Coastal and Shelf Science, 19, 119-128.
[https://doi.org/10.1016/0272-7714(84)90056-8]
- Ministry of Oceans and Fisheries (MOF), Korea Marine Environment Management Corporation (KOEM), 2024, Annual Report on Marine Environment Monitoring in Korea 2023. Vol. 28.
- Ministry of Environment (MOE), 2024, Installation and operation plan of monitoring network for water environmental measurement, Notification Num: 2024-292.
-
Moe, S.J., Stelzer, R.S., Forman, M.R., Harpole, W.S., Daufresne, T. and Yoshida, T., 2005, Recent advances in ecological stoichiometry: insights for population and community ecology. Oikos, 109, 29-39.
[https://doi.org/10.1111/j.0030-1299.2005.14056.x]
-
Mompeán, C., Bode, A., Latasa, M., Fernández-Castro, B., Mouriño-Carballido, B. and Irigoien, X., 2016, The influence of nitrogen inputs on biomass and trophic structure of ocean plankton: a study using biomass and stable isotope size-spectra. Journal of Plankton Research, 38, 1163-1177.
[https://doi.org/10.1093/plankt/fbw052]
-
Nriagu, J.O. and Pacyna, J.M., 1988, Quantitative assessment of worldwide contamination of air, water and soils by trace metals. Nature, 333, 134-139.
[https://doi.org/10.1038/333134a0]
-
Paczkowska, J., Brugel, S., Rowe, O., Lefébure, R., Brutemark, A. and Andersson, A., 2020, Response of Coastal Phytoplankton to High Inflows of Terrestrial Matter. Frontiers in Marine Science, 7, 80.
[https://doi.org/10.3389/fmars.2020.00080]
-
Rabouille, C., Mackenzie, F.T. and Ver, L.M., 2001, Influence of the human perturbation on carbon, nitrogen, and oxygen biogeochemical cycles in the global coastal ocean. Geochimica et Cosmochimica Acta, 65, 3615-3641.
[https://doi.org/10.1016/S0016-7037(01)00760-8]
-
Rastogi, N., Agnihotri, R., Sawlani, R., Patel, A., Babu, S.S. and Satish, R., 2020, Chemical and isotopic characteristics of PM10 over the Bay of Bengal: Effects of continental outflow on a marine environment. Science of The Total Environment, 726, 138438.
[https://doi.org/10.1016/j.scitotenv.2020.138438]
-
Regnier, P., Friedlingstein, P., Ciais, P., Mackenzie, F.T., Gruber, N., Janssens, I.A., Laruelle, G.G., Lauerwald, R., Luyssaert, S., Andersson, A.J., Arndt, S., Arnosti, C., Borges, A.V., Dale, A.W., Gallego-Sala, A., Goddéris, Y., Gooseens, N., Hartmann, J., Heinze, C., Ilyina, T., Joos, F., LaRowe, D.E., Leifeld, J., Meysman, F.J.R., Munhoven, G., Raymond, P.A., Spahni, R., Suntharalingam, P. and Thullner, M., 2013, Anthropogenic perturbation of the carbon fluxes from land to ocean. Nature Geoscience, 6, 597-607.
[https://doi.org/10.1038/ngeo1830]
-
Regnier, P., Resplandy, L. and Najjar, R.G., 2022, The land-to-ocean loops of the global carbon cycle. Nature, 603, 401-410.
[https://doi.org/10.1038/s41586-021-04339-9]
-
Sáez, J.A., Flores, P., Bustamante, M.Á., Sanchez-Hernandez, J.C., Moral, R. and Pérez-Murcia, M.D., 2020, Nitrogen Isotope Fractionation during Composting of Sewage and Agri-Food Sludge with Pruning Waste. Agronomy, 10, 1954.
[https://doi.org/10.3390/agronomy10121954]
-
Schlesinger, W.H. and Melack, J.M., 1981, Transport of organic carbon in the world’s rivers. Tellus, 33, 172-187.
[https://doi.org/10.3402/tellusa.v33i2.1070]
-
Schlünz, B. and Schneider, R., 2000, Transport of terrestrial organic carbon to the oceans by rivers: re-estimating flux- and burial rates. International Journal of Earth Sciences, 88, 599-606.
[https://doi.org/10.1007/s005310050290]
-
Seo, S.H., 2022, Risk Assessment of Inhalation Exposure to Per-and Polyfluoroalkyl Substances (PFASs) in Industrial, Urban-residential and Rural Areas Near Hyeongsan River, Pohang. Journal of Environmental Analysis, Health and Toxicology, 25, 51-57 (in Korean with English abstract).
[https://doi.org/10.36278/jeaht.25.2.51]
-
Simoneit, B.R.T., 2006, Atmospheric Transport of Terrestrial Organic Matter to the Sea. Marine Organic Matter: Biomarkers, Isotopes and DNA, 165-208.
[https://doi.org/10.1007/698_2_006]
-
Smittenberg, R.H., Eglinton, T.I., Schouten, S. and Damsté, J.S.S., 2006, Ongoing Buildup of Refractory Organic Carbon in Boreal Soils During the Holocene. Science, 314, 1283-1286.
[https://doi.org/10.1126/science.1129376]
-
Song, K., Shang, Y., Wen, Z., Jacinthe, P.A., Liu, G., Lyu, L. and Fang, C., 2019, Characterization of CDOM in saline and freshwater lakes across China using spectroscopic analysis. Water Research, 150, 403-417.
[https://doi.org/10.1016/j.watres.2018.12.004]
-
Stock, B.C., Jackson, A.L., Ward, E.J., Parnell, A.C., Phillips, D.L. and Semmens, B.X., 2018, Analyzing mixing systems using a new generation of Bayesian tracer mixing models. PeerJ, 6, e5096.
[https://doi.org/10.7717/peerj.5096]
-
Thayalakumaran, T., Bristow, K.L., Charlesworth, P.B. and Fass, T., 2008, Geochemical conditions in groundwater systems: Implications for the attenuation of agricultural nitrate. Agricultural Water Management, 95, 103-115.
[https://doi.org/10.1016/j.agwat.2007.09.003]
-
Thornton, S.F. and McManus, J., 1994, Application of Organic Carbon and Nitrogen Stable Isotope and C/N Ratios as Source Indicators of Organic Matter Provenance in Estuarine Systems: Evidence from the Tay Estuary, Scotland. Estuarine, Coastal and Shelf Science, 38, 219-233.
[https://doi.org/10.1006/ecss.1994.1015]
-
Tian, Y., Liu, X., Huo, R., Shi, Z., Sun, Y., Feng, Y. and Harrison, R.M., 2021, Organic compound source profiles of PM2.5 from traffic emissions, coal combustion, industrial processes and dust. Chemosphere, 278, 130429.
[https://doi.org/10.1016/j.chemosphere.2021.130429]
-
Torres, I.C., Inglett, P.W., Brenner, M., Kenney, W.F. and Ramesh Reddy, K., 2012, Stable isotope (δ13C and δ15N) values of sediment organic matter in subtropical lakes of different trophic status. Journal of Paleolimnology, 47, 693-706.
[https://doi.org/10.1007/s10933-012-9593-6]
- U.S. Environmental Protection Agency (EPA), 2012, Guidelines for Water Reuse.
-
van der Lee, G.H., Vonk. J.A., Verdonschot, R.C.M., Kraak, M.H.S., Vendonschot, P.F.M. and Huisman, J., 2021, Eutrophication induces shifts in the trophic position of invertebrates in aquatic food webs. Ecology, 102, e03275.
[https://doi.org/10.1002/ecy.3275]
-
Walters, W.W., Tharp, B.D., Fang, H., Kozak, B.J. and Michalski, G., 2015, Nitrogen Isotope Composition of Thermally Produced NOx from Various Fossil-Fuel Combustion Sources. Environmental Science & Technology, 49, 11363-11371.
[https://doi.org/10.1021/acs.est.5b02769]
-
Wang, C., Lv, Y. and Li, Y., 2018, Riverine input of organic carbon and nitrogen in water-sediment system from the Yellow River estuary reach to the coastal zone of Bohai Sea, China. Continental Shelf Research, 157, 1-9.
[https://doi.org/10.1016/j.csr.2018.02.004]
- Woo, J.S., Lee, H.J., Park, J.K., Park, K.K., Cho, D.J., Jang, D.J., Park, S.J., Choi, M.S. and Yoo, J.K., 2019, Background concentration and contamination assessment of heavy metals in Korean coastal sediments. Journal of the Korean Society of Oceanography, 24, 64-78 (in Korean with English abstract).
-
Wymore, A.S., Johnes, P.J., Bernal, S., Brookshire, E.N.J., Fazekas, H.M., Helton, A.M., Argerich, A., Barnes, R.T., Coble, A.A., Dodds, W.K., Haq, S., Johnson, S.L., Jones, J. B., Kaushal, S.S., Kortelainen, P., López-Lloreda, C., Rodríguez-Cardona, B.M., Spencer, R.G.M., Sullivan, P.L. and McDowell, W.H., 2021, Gradients of Anthropogenic Nutrient Enrichment Alter N Composition and DOM Stoichiometry in Freshwater Ecosystems. Global Biogeochemical Cycles, 35, e2021GB006953.
[https://doi.org/10.1029/2021GB006953]
-
Xie, L., Gao, X., Liu, Y., Yang, B., Yuan, H., Li, X., Song, J., Zhao, J. and Xing, Q., 2023, Atmospheric deposition as a direct source of particulate organic carbon in region coastal surface seawater: Evidence from stable carbon and nitrogen isotope analysis. Science of the Total Environment, 854, 158540.
[https://doi.org/10.1016/j.scitotenv.2022.158540]
-
Yang, H.J., Jeong, H.J., Bong, K.M., Jin, D.R., Kang, T.W., Ryu, H.S., Han, J.H., Yang, W.J., Jung, H., Hwang, S.H. and Na, E.H., 2020, Organic matter and heavy metal in river sediments of southwestern coastal Korea: Spatial distributions, pollution, and ecological risk assessment. Marine Pollution Bulletin, 159, 111466.
[https://doi.org/10.1016/j.marpolbul.2020.111466]
-
Yates, C.A., Johnes, P.J., Owen, A.T., Brailsford, F.L., Glanville, H.C., Evans, C.D., Marshall, M.R., Jones, D.L., Lloyd, C.E.M., Jickells, T. and Evershed, R.P., 2019, Variation in dissolved organic matter (DOM) stoichiometry in UK freshwaters: Assessing the influence of land cover and soil C: N ratio on DOM composition. Limnology and Oceanography, 64, 2328-2340.
[https://doi.org/10.1002/lno.11186]
-
Yunker, M.B., Belicka, L.L., Harvey, H.R. and Macdonald, R.W., 2005, Tracing the inputs and fate of marine and terrigenous organic matter in Arctic Ocean sediments: a multivariate analysis of lipid biomarkers. Deep-Sea Research Part II-Topical studies in oceanography, 52, 3478-3508.
[https://doi.org/10.1016/j.dsr2.2005.09.008]
-
Zendehbad, M., Cepuder, P., Loiskandl, W. and Stumpp, C., 2019, Source identification of nitrate contamination in the urban aquifer of Mashhad, Iran. Journal of Hydrology: Regional Studies, 25, 100618.
[https://doi.org/10.1016/j.ejrh.2019.100618]
-
Zhang, A., Lei, K., Lang, Q. and Li, Y., 2022, Identification of nitrogen sources and cycling along freshwater river to estuarine water continuum using multiple stable isotopes. Science of the Total Environment, 851, 158136.
[https://doi.org/10.1016/j.scitotenv.2022.158136]
-
Zhang, C., Li, W., Du, W., Cao, B., Wang, W., Pang, B., Dou, H., Ao, W., Liu, B. and Yao, S., 2023, A High Accumulation of Dissolved Organic Matter in the Water Resulting from Terrestrial Input into a Large, Shallow Steppe Lake. Water, 15, 1646.
[https://doi.org/10.3390/w15091646]
-
Zhang, Y., Shi, P., Li, F., Wei, A., Song, J. and Ma, J., 2018, Quantification of nitrate sources and fates in rivers in an irrigated agricultural area using environmental isotopes and a Bayesian isotope mixing model. Chemosphere, 208, 493-501.
[https://doi.org/10.1016/j.chemosphere.2018.05.164]