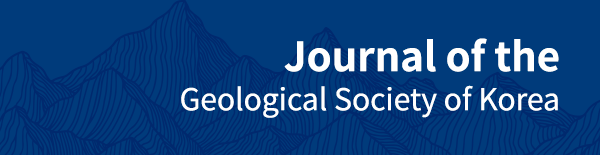
단양지역 층리평행전단구조의 운동학적 특성과 지체구조적 의미
부경대학교 지구환경시스템과학부 지질구조재해연구실초록
옥천대의 주요 구조선인 옥동단층과 각동충상단층 사이에 분포하는 태백형 조선누층군을 따라 층리평행 전단구조가 보고되어 있어 해당 구조에 대한 운동학적 특성을 파악하기 위해 연구를 수행하였다. 이를 위해 단양지역에서 이러한 전단구조가 인지되는 노두들에 대해 야외조사를 수행하였으며, 운동학적 지시자를 식별하여 운동감각을 알아내고 C′ 엽리를 이용한 소용돌이도 분석을 통해 운동학적 특성을 알아냈다. 그 결과 엽상규산염광물이 풍부한 묘봉층, 화절층, 두무골층에서 우수향 주향이동을 지시하는 C′ 엽리가 다수 관찰되었다. 또한 좌수향 주향이동을 지시하는 전단습곡이 일부 나타나는데 이는 우수향 C′ 엽리를 변형시키고 있어 우수향 전단 이후에 발생한 것으로 보인다. 운동특성을 이해하기 위하여 우수향을 지시하는 C′ 엽리에 대해 평균 운동학적 소용돌이수(Wm) 분석을 실시하였으며, 그 결과 옥동단층과 가까운 묘봉층은 0.738~0.813의 값이 나타나 단순전단이 우세하지만, 옥동단층과 먼 화절층과 두무골층은 0.170~0.466 사이의 값을 보여주어 순수전단이 우세하게 나타났다. 이는 옥동단층을 가로지르는 방향으로 변형분할(strain partitioning)이 발생하였음을 지시하는 것으로 보인다. 층리평행 전단구조의 운동감각과 Wm 변화양상은 해당구조가 옥동단층과 연관이 있음을 보여주며, 변형분할 양상과 최소순간신장축(minimum instantaneous stretching axes; ISA3)은 옥동단층이 전달압축 환경(WNW-ESE 압축)에 놓여있었음을 지시한다. 또한 Wm을 바탕으로 도출한 고수렴각(paleo-convergence angle)은 옥동단층으로부터 멀어질수록 ENE-WSW에서 WNW-ESE방향으로 회전하는 것으로 나타났으며, 이는 각동충상단층과의 운동학적 연관성을 시사하는 것으로 해석된다.
Abstract
Layer-parallel shearing has been reported along the Taebaek-type Joseon Supergroup, which is distributed between the Okdong Fault and the Gakdong Thrust, which are the major tectonic lines along the Okcheon Belt. We conducted a study to understand the kinematics of these structures. We identified several shear indicators through field surveys in the Danyang area, providing information on the sense of shear and the vorticity analysis using C′ foliations. As a result, a number of C′ foliations, indicating right-lateral sense, were observed in the Myobong Formation, Hwajeol Formation, and Dumugol Formation, which are phyllosilicate rich layers. In contrast, there are also some shear folds indicating left-lateral shear, which deformed the right-lateral C′ foliations, suggesting that it occurred after the right-lateral shear. The mean kinematic vorticity number (Wm) derived from the right-lateral C′ foliations, range from 0.738 to 0.813 in the Myobong Formation near the Okdong Fault, indicating prevalent simple shear. In contrast, they range from 0.170 to 0.466 in the Hwajeol and Dumugol Formations farther from the Okdong Fault, indicating predominance of pure shear. This may indicate stress change and strain partitioning between the Okdong fault. The kinematics and changes of the Wm across the Okdong fault indicate that they are strongly associated with the movement of the Okdong fault, and the minimum instantaneous stretching axes (ISA3) and the strain partitioning indicates that the Okdong fault experienced transpressional stress (WNW-ESE compression) condition. In addition, the paleo-convergence angle based on Wm was rotated clockwise from ENE-WSW toward the WNW-ESE with distance from the Okdong Fault, which may suggest a kinematic relation with the Gakdong Thrust.
Keywords:
layer-parallel shearing structures, C′ foliation, flow apophyses, mean kinematic vorticity number (Wm), strain partitioning키워드:
층리평행 전단구조, C′ 엽리, 유동아포피시스, 평균 운동학적 소용돌이수(Wm), 변형분할1. 서 론
한반도를 NE-SW방향으로 가로지르는 옥천대는 다중변형을 받아 구조적, 층서적으로 매우 복잡한 지체구조구이다(그림 1a; Kee et al., 2019). 이는 해당지역이 긴 시간에 걸쳐 형성되면서 많은 지질학적 사건들을 기록하고 있음을 의미하며, 따라서 한반도 지체구조 연구에 매우 중요한 지역이다. 한반도 지체구조의 형성과정에 대해서는 여러 가설이 제시되었으나(e.g., Chough et al., 2000; Kwon et al., 2009; Oh and Kim, 2021) 아직까지 대부분의 학자들이 동의하는 모델은 제시되지 않았다. 한반도 지체구조의 형성과정을 규명하기 위해서는 먼저 지체구조단위들의 시기와 발달특성, 그리고 주요 구조선(취성단층과 연성전단대)의 운동학적 특성에 대한 이해가 뒷받침되어야 한다.
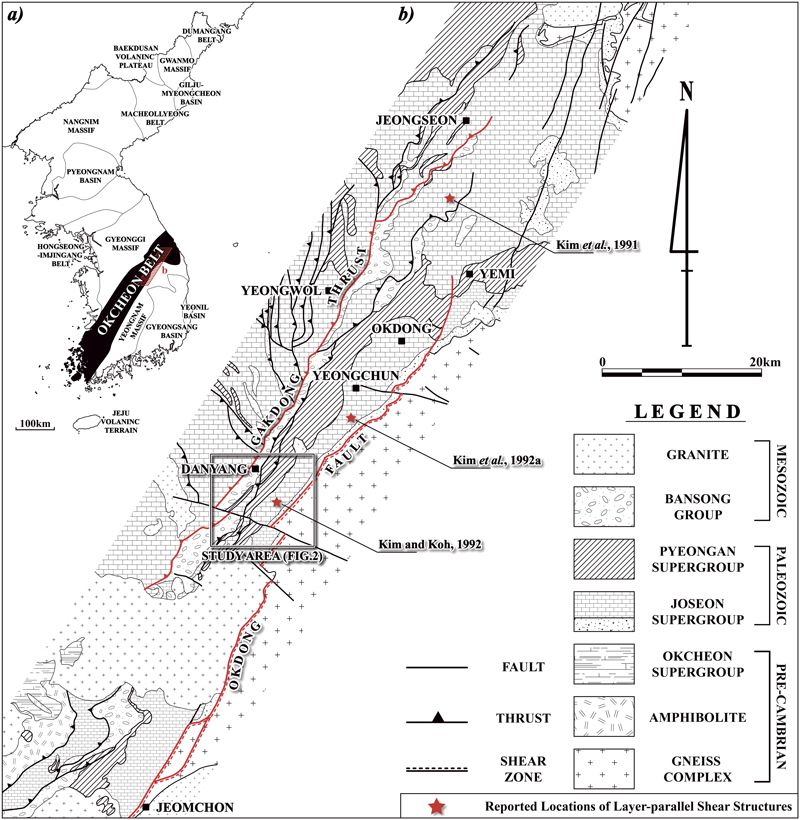
Location of the study area. (a) Tectonic province of the Korean Peninsula (highlighted in black: Okcheon Belt). (b) Fault trace map around the Okdong Fault and Gakdong Thrust. Red stars indicate reported areas of layer-parallel shear structures (modified from Kim et al., 1989 and Kee et al., 2019).
옥동단층은 옥천대의 주요 구조선 중 하나로, 옥천대와 영남육괴의 경계단층이다. 옥동단층은 예미지역부터 점촌지역까지 NE-SW방향으로 100여 km의 연장을 갖는 연성전단대이다(Kim et al., 1989). 각동충상단층은 덕포리충상단층, 또는 별곡충상단층이라고도 하며, 서북옥천대와 동남옥천대의 경계단층 중 하나로(Lim et al., 2005) 정선지역에서 단양지역까지 NE-SW방향으로 발달하고 있다(Kee et al., 2019). 단양지역은 이 두 구조선이 병치되어 있는 지역으로, 습곡을 비롯한 다양한 변형구조가 발달하고 있어 지체구조학 및 구조지질학적으로 중요한 지역이다. 하지만 그 중요성에 비해 연구가 부족하여 대부분의 연구가 1900년대에 머물러 있다(e.g., Kim and Koh 1992; Kim et al., 1994).
앞서 언급한 옥동단층과 각동충상단층 사이 지역을 따라 층리와 평행한 연성전단구조(이하 층리평행 전단구조)가 보고되어 있다(e.g., Kim and Lee, 1991; Kim and Koh, 1992; Kim et al., 1992a). 이러한 단층 주변 변형구조들은 단층의 운동학적 특성에 대한 정보를 담고 있어 중요하지만, 해당 구조의 운동학적 특성과 옥동단층 및 각동충상단층 등 주변 구조들과의 관계가 명확하게 규명되지 않았다.
지금까지 우리나라에서 수행된 연성전단대의 운동학적 특성에 대한 연구는 노두규모-미소규모에서 나타나는 전단지시자를 통해 전단감각을 알아내는 것을 위주로 수행되었다. 하지만 연성전단대는 일반적으로 취성단층에 비해 넓은 폭을 가지며 전단면을 따르는 전단변형뿐만 아니라 전단대를 가로지르는 방향의 변형을 동반한다(Fossen and Cavalcante, 2017). 따라서 전단대 내부의 변형특성을 파악할 필요가 있다.
물체가 변형될 때 순간적인 신장량에 대한 상대적 회전량을 나타내는 운동학적 소용돌이수(kinematic vorticity number)는 본래 유체역학에서 사용된 개념으로 지질학에서는 McKenzie (1979)와 Means et al. (1980)에 의해 처음 도입되었다. 이후 많은 연구를 통해 전단대에서의 운동학적 소용돌이수 활용에 대해 알려져 왔으며(e.g., Passchier, 1987; Tikoff and Fossen, 1995; Kurz and Northrup, 2008; Xypolias, 2010), 전단대 연구에서 순수전단(pure shear)과 단순전단(simple shear)의 비율을 결정하는 등 다양하게 활용되고 있다(e.g., Sullivan, 2009; Zhang et al., 2009; Li et al., 2010; Ni et al., 2019). 하지만 아직까지 우리나라에서는 운동학적 소용돌이수를 활용한 연성전단대 특성에 대한 연구가 진행된 바 없다.
따라서 본 논문에서는 야외조사에서 식별되는 여러 전단지시자들과 구조요소들을 이용하여 옥동단층 및 옥동단층 주변에서 관찰되는 층리평행 전단구조의 운동학적 소용돌이수를 측정하여 운동학적 특성을 기술하고, 연구결과의 지체구조적 의미에 대해 고찰한다.
2. 지질개요
연구지역인 단양지역의 지질은 고원생대 편마암복합체, 전기 고생대 태백형 및 영월형 조선누층군, 후기 고생대 평안누층군, 중생대 반송층군 등으로 구성되어 있다(Won and Lee, 1967; Kim and Koh, 1992). 연구지역의 남동측에는 기반암인 편마암복합체가 분포하고 있으며 북서방향으로 갈수록 상위(젊은) 지층이 나타나는 양상을 보이지만 일부 지층이 북동-남서 방향의 충상단층에 의해 반복되는 양상이 나타난다(그림 2; Kim and Koh, 1992). 연구지역의 태백형 조선누층군은 하부로부터 장산층, 묘봉층, 대기층, 화절층, 동점층, 두무골층, 막골층으로 구성되며 막골층의 상위에 만항층이 평행부정합 관계로 접하고 있다(Kim and Koh, 1992). 장산층과 묘봉층은 주로 쇄설성 퇴적암으로 구성되며, 장산층은 사암 및 규암, 묘봉층은 셰일 및 점판암으로 구성된다. 묘봉층 상위의 대기층에서부터 최상위 막골층까지는 주로 석회암으로 구성되어 있어 과거 대석회암통으로 분류된 바 있으나, 화절층과 두무골층은 석회암과 셰일이 엽층상으로 교호하는 양상을 보인다(Kobayashi, 1966).
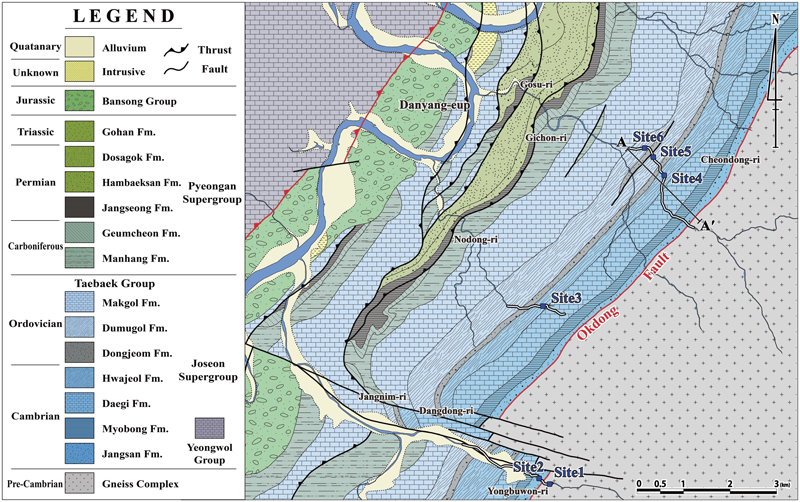
Geological map of the study area. The A-A′ section is for Fig. 10 (modified from Kim and Koh, 1992).
연구지역의 주요 구조선으로는 옥동단층과 각동충상단층이 있으며, 두 단층은 모두 북동-남서 주향에 북서로 경사하고 있다. 퇴적암류의 일반적인 층리방향은 두 주요 단층과 평행한 북동-남서 주향을 가지고 북서 경사를 하는 것으로 보고되어 있다(Kim and Koh, 1992).
3. 연구방법
본 연구에서는 전단구조의 운동학적 특징을 파악하기 위해 전단지시자를 통하여 전단감각을 알아내고 운동학적 소용돌이수 분석을 실시하였다. 운동학적 소용돌이수는 Wk로 표기하며, 물체가 변형될 때 응력변형타원체의 회전속도와 응력변형누적속도 간의 비율을 나타내는 무차원의 수로 정의된다. Wk = 0인 경우 응력변형타원체의 주응력변형축(principal strain axes)이 회전하지 않는 동축변형(coaxial deformation), 즉 순수전단을 의미한다. 0< Wk < 1인 경우는 비동축변형(non-coaxial deformation)의 준단순전단(subsimple shear), Wk = 1일 때는 단순전단을 나타낸다(Fossen, 2016).
자연상태에서 Wk를 적용하는 것은 몇가지 어려움이 따른다. Wk를 구하기 위해서는 외부 기준 프레임(external reference frame)에 대한 정보가 필요하지만 실제 노두에서 이를 고려하기 어렵다(Passchier, 1997; Graziani et al., 2021). 따라서 대부분의 소용돌이도 연구에서 내부 기준 프레임(internal reference frame)을 사용한다(e.g., Kurz and Northrup, 2008; Sullivan, 2009; Xypolias, 2010; Zhang et al., 2009; Li et al., 2010; Ni et al., 2019). 내부 기준을 사용한 운동학적 소용돌이수는 단면 운동학적 소용돌이수(sectional kinematic vorticity number; Wn; Graziani et al., 2021)로 표현되며, Wn은 단면 내에서 순간적인 소용돌이수를 나타낸다(Passchier, 1997; Graziani et al., 2021). 추가로 고려해야할 것은 변형이 정상상태유동이 아닌 경우 변형 이력 동안 Wn이 변할 수 있다. 실제 야외에서는 최종 변형 결과만 관찰가능하기 때문에 순간적인 변형을 나타내는 Wn을 구하는 것은 큰 어려움이 따른다. 따라서 실제적으로 구할 수 있는 운동학적 소용돌이수는 평균 운동학적 소용돌이수(mean kinematic vorticity number; Wm; Xypolias, 2010)이기 때문에 본 연구에서는 Wm을 사용하여 운동학적 특성을 규명하였다.
Wm을 산출하는 방법은 다양하나 본 연구지역의 암석에서는 C′ 엽리(C′ foliation)가 주로 나타나기 때문에 이를 이용한 산출방법을 적용하였다. C′ 엽리는 C′ 전단띠(C′ shear band), 전단띠 벽개(shear band cleavage) 또는, 확장성 파랑벽개(extensional crenulation cleavage)라고도 불리며 전단대를 따라 주 전단면에 대해 일정한 각도를 가지며 기존의 엽리를 절단하는 부수전단띠(subsidiary shear band)를 의미한다. 일반적으로 주 전단면의 운동감각과 동일한 정감각(synthetic)으로 나타나지만 일부 역방향(antithetic)을 보이는 경우도 있어 해석에 주의하여야 한다(Gillam et al., 2013).
그림 3a는 준단순전단(sub-simple shear 또는 일반전단, general shear)에서 정감각 C′ 엽리와 전단면, 그리고 세 개의 유동아포피시스(flow apophyses) 사이의 기하적 관계를 나타낸 모식도이다(Fossen and Tikoff, 1997; Kurz and Northrup, 2008). 해당 그림에서 세 축 X, Y, Z는 서로 수직이다. 전단은 XY 평면을 따라 발생하며 전단방향은 X축이다. Z축은 전단면에 수직인 축을 지시한다. 일반적으로 전단지시자의 관찰은 전단면과 수직이며 전단방향에 평행한 XZ 평면에서 수행된다. 유동아포피시스는 변형 중 물질이 넘을 수 없는 가상의 선을 의미하며 AP1, AP2, AP3의 세 가지 방향을 가진다. 세 방향의 유동아포피시스 중, XZ 평면 상에 존재하는 AP1과 AP2 사이의 예각을 ‘α’ 라고 한다. Wm은 α에 의해 정의되며 그 식은 다음과 같다.
(1) |
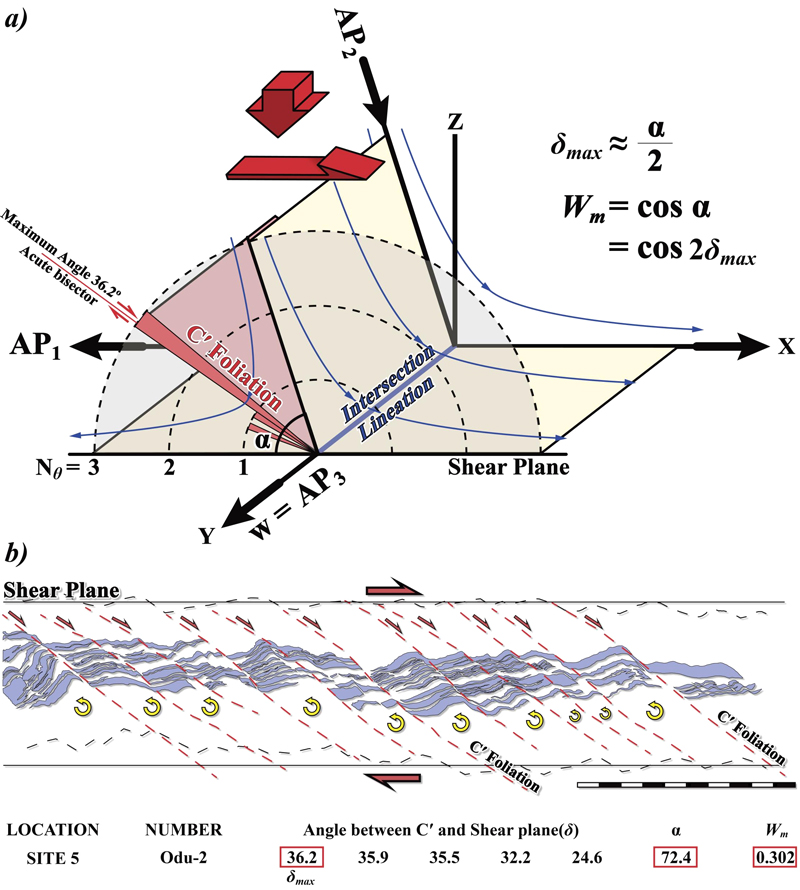
A method for calculating mean kinematic vorticity number (Wm) using the C′ foliation. (a) Conceptual illustration of the relationship between flow apophyses and C′ in sub-simple shear. α is acute angle between AP1 and AP2, δ is angle between C′ and shear plane. The C′ occurs along the acute bisector between AP1 and AP2 and rotates toward AP1. Thus, δ max ≊ α/2, Wm = cos α (modified from Fossen and Tikoff, 1997 and Kurz and Northrup, 2008). (b) Example of calculating Wm. Measure the attitude of C′ and the shear plane and use the largest value of the angle between the two planes.
Simpson and De Paor (1993)는 정상상태의 비동축 변형에서 정감각의 C′ 엽리는 AP1과 AP2의 예각 이등분선에서 형성되며, AP1방향을 향해 천천히 회전을 겪고 역감각 C′의 경우 둔각이등분선에서 형성된다고 주장하였다. Kurz and Northrup (2008)는 반상쇄정을 이용하여 Wm을 구한 뒤 C′과 Wm 사이의 관계를 분석하였고, 그 결과 전단면과 정감각 C′ 엽리가 이루는 각도가 α의 절반과 같거나 작은 값으로 나타났으며, 역감각 C′의 경우 둔각이등분선에 집중되어 나타났다. 이는 Simpson and De Paor (1993)의 주장과 잘 일치한다. 이러한 연구결과를 통해 다음과 같은 식을 얻을 수 있다.
(2) |
이때 δmax는 정감각 C′과 전단면이 이루는 최대 각도를 의미하며, AP1과 AP2의 예각 이등분으로 45°보다 클 수 없다. 그림 3b는 C′을 이용하여 Wm을 산출한 예시이다.
본 연구에서는 옥동단층과 층리를 가로지르는 하천을 따라 3개의 조사경로를 설정하였으며(그림 2), 조사경로를 따라 전단지시자들을 식별하였다. 또한 정밀한 자세측정을 통해 정확한 운동감각을 파악하였다. 자세 측정 시 오차를 최소화하기 위해 면구조가 3차원적으로 드러나 있는 구조만을 선별적으로 측정하였다. 본 연구에서 사용된 자세표기는 방위각(azimuth)법을 적용하였으며, 오른손법칙을 따랐다. 정밀하게 측정한 자세를 등면적투영망에 하반구 투영하여 δ를 도출하였으며 그 중 최댓값을 이용하여 Wm을 산출하였다.
α와 Wm은 변형기간 중 어떠한 시간에 최대와 최소의 신장방향을 지시하는 3개의 직교하는 순간신장축(instantaneous stretching axes; ISA)과 큰 관련이 있다. 그 중 ISA1은 최대 신장축이며 ISA3는 최소 신장축이다. ISA1과 전단면 사이의 각 θ와 α의 관계는 다음과 같다(Tikoff and Fossen, 1995).
(3) |
α ≈ 2δmax이므로 이를 바탕으로 식 3을 전개하면 다음과 같은 식을 얻을 수 있다.
(4) |
즉 δmax와 ISA1의 사이 각은 45º이며 이와 직교하는 ISA3 또한 45º의 각관계를 가진다. 본 연구에서는 위 관계를 바탕으로 ISA를 추론하였다.
Wm과 유동아포피시스 간의 기하학적 관계를 이용하면 지체구조운동에 대한 정보를 추가로 얻을 수 있다(그림 3). 두 유동아포피시스 상의 물질은 유동아포피시스를 따라 직선운동을 겪게 되는데 AP1은 전단방향에 따른 움직임을 나타내는 반면, AP2는 전단방향을 가로지르는 움직임을 나타낸다. 이는 전단대 양쪽 지괴가 AP2방향으로 수렴함을 의미한다. 따라서 전단대의 Wm을 이용하여 전단대 양쪽 지괴의 실제 운동방향인 고수렴각(paleo-convergence angle)을 알아낼 수 있다(Fossen and Tikoff, 1998).
4. 결 과
4.1 야외조사 결과
야외조사 결과 연구지역 내 6개 지점에서 원압쇄암과 층리평행 전단구조를 식별하였다(그림 2). 지점 1은 용부원리 일대 옥동단층 하반에 위치한 흑운모화강암질편마암으로 이루어진 노두로 약 50 cm의 비교적 좁은 구간에서 원압쇄암의 양상을 보인다. 흑운모화강암질편마암 내에 운모류가 밀집되어 있는 형태의 C′ 엽리를 관찰할 수 있으며, 이를 통해 우수향 주향이동 운동감각을 확인할 수 있다(그림 4). 해당 노두의 전단대 경계(236º, 63º)와 C′(257º, 66º)의 자세를 도시하여 운동감각을 확인한 결과 교차선구조는 63º, 318º, 미끌림 방향은 03º, 054º의 순수한 우수향 주향이동을 지시한다. 이는 기존의 옥동단층에 대한 연구결과와 잘 일치한다(Cluzel et al., 1991; Kwon et al., 1995).
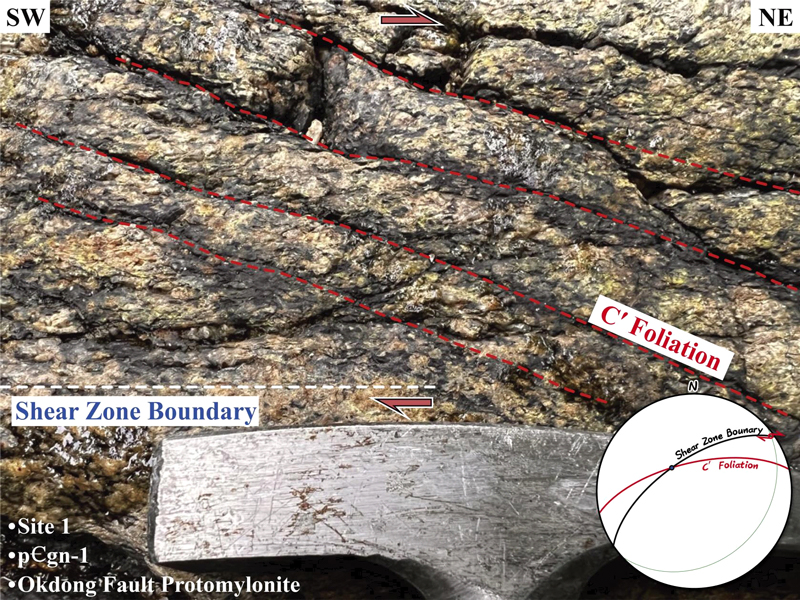
Protomylonite of the Okdong Fault at site 1 in Fig.2. Inset is stereo plot of shear zone boundary (black) and C′ foliation (red). The C′ foliation at this site indicates a right-lateral strike-slip movement.
그림 5는 지점 2~6에서 나타나는 우수향 전단지시자의 예시와 정밀 스케치를 통해 분석한 결과이다. 우수향을 지시하는 전단지시자는 대부분 C′ 엽리의 형태로 나타난다. C′ 엽리는 형태적으로 압축성 파랑벽개(compressional crenulation cleavage)와 유사하지만, 연장성이 좋은 압축성 파랑벽개에 비해 전단이 일어나는 좁은 영역에 한정되어 발달하는 차이점이 있다(Passchier and Trouw, 2005). C′에 의한 변형은 층 내 셰일이 우세한 부분에 집중되고 전단대의 경계는 곧은 형태를 보인다. 야외조사에서 획득한 δ가 45°보다 작기 때문에 이들은 모두 정감각 C′인 것으로 보인다(표 1). 우수향 C′ 엽리는 주로 묘봉층, 화절층, 두무골층에 분포한다. 그 중 엽층리가 잘 발달하는(5 mm 내외) 두무골층이 가장 우세하고 형태적으로 뚜렷한 전단면을 가진다(그림 5e). 반면 엽층리가 상대적으로 두껍거나 잘 발달하지 않는(5-30 mm) 화절층에서는 비교적 드물게 나타나며 전단면의 형태가 뚜렷하지 않다(그림 5c, 5d).
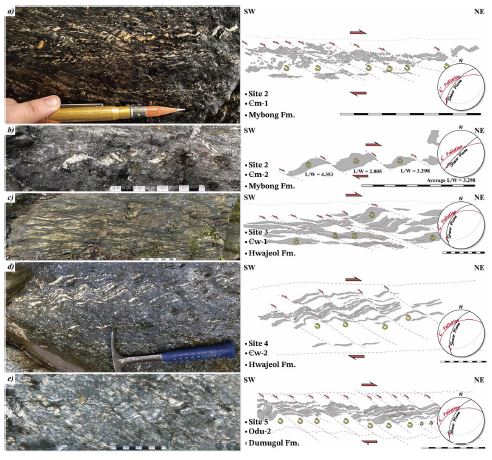
Examples of layer-parallel shear structures indicating right-lateral shear. (a) C′ foliation in Myobong Formation. (b) Shear band boudin in Myobong Formation. (c) C′ foliation in Hwajeol Formation. (d, e) C′ foliation in Dumugol Formation. Inset is stereo plot of shear zone boundary (black) and C′ foliation (red). All scale bars are 10 cm. The structures indicate extension and thinning of layers.
이외의 우수향 구조로는 단양군 용부원리 일대의 묘봉층 노두에서 관찰 가능한 비대칭 부딘(asymmetric boudin) 구조가 있다(그림 5b). 비대칭 부딘은 전단방향에 대해 반대방향으로 회전하는 전단띠 부딘(shear band boudin)과 동일한 방향으로 회전하는 도미노 부딘(domino boudin)이 있다. 부딘이 어떤 회전을 겪는지는 부딘의 종횡비에 따라 달라진다(Hanmer, 1986; Goscombe and Passchier, 2003). Goscombe and Passchier (2003)의 연구에 따르면 부딘의 평균 종횡비(L/W)는 전단띠 부딘의 경우 3.62, 도미노 부딘의 경우 2.12로 전단띠 부딘에서 높은 수치를 보인다. 연구지역에서 관찰된 비대칭 부딘의 경우 종횡비 평균이 3.298로 전단띠 부딘에 가까우며 우수향을 지시하는 것으로 보인다(그림 5b).
연구지역의 층리평행 전단구조는 우수향이 우세하지만 일부 좌수향을 지시하는 전단습곡(SW 전향을 가지는 S-Type 습곡)이 관찰되며(그림 6), 일부 좌수향 전단습곡은 우수향 C′ 엽리와 중복되어 나타난다(그림 7). 그림 7은 우수향 C′ 엽리와 좌수향 전단습곡이 중복되어 나타나는 노두로 두 변형 사이의 관계를 잘 나타낸다. 층리는 NNE-SSW방향으로 발달하고 있으며, C′ 엽리는 셰일의 함량이 상대적으로 높은 층에 규제되어 발달하고 있다. 층의 SSW측(그림 7a)은 C′이 발달하는 셰일 우세부의 두께가 상대적으로 얇다. 또한 일반적인 C′과 이에 수반되는 시그모이드 형태(sigmoidal-shape)와 C′ 사이 층의 반향회전 (반시계방향)이 잘 나타나고 있다.
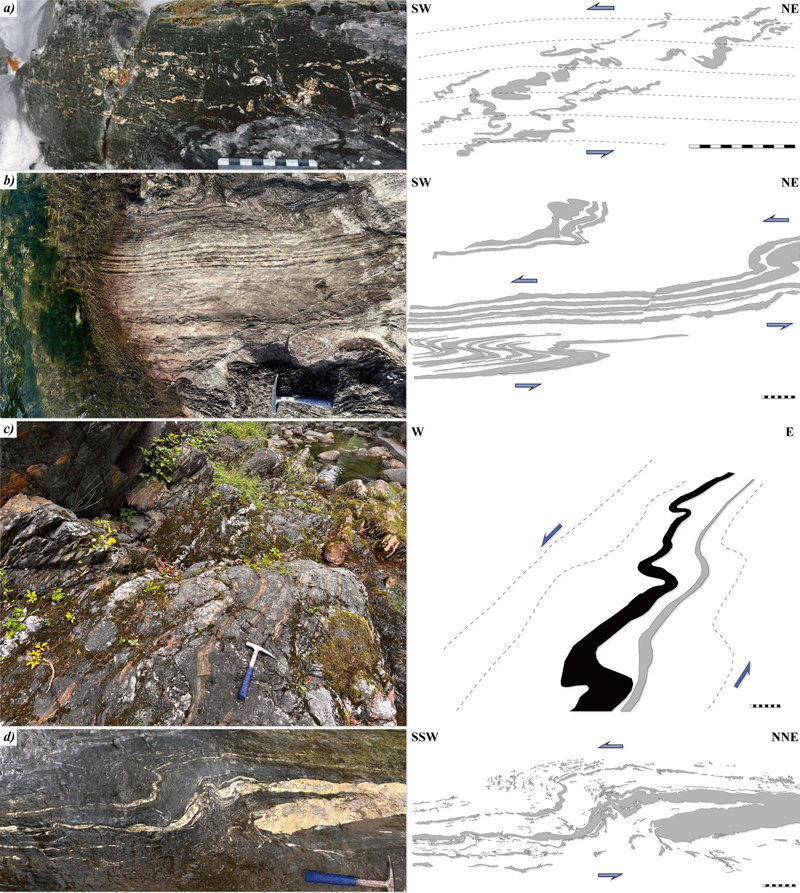
Examples of layer-parallel shear structures indicating left-lateral shear. (a) Shear fold in Myobong Formation. (b, c) Shear fold in Hwajeol Formation. (d) Shear fold in Dumugol Formation. All scale bars are 10 cm. The structures indicate contraction and thickening of layers.
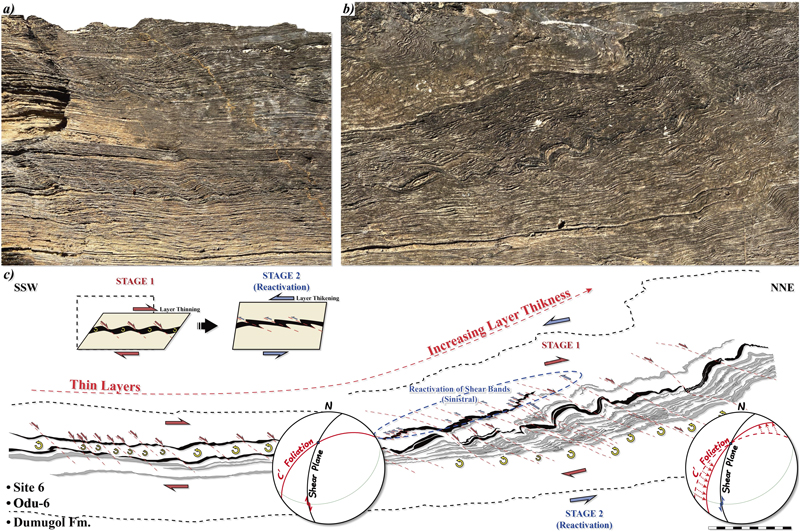
Overprinted structure with a left-lateral shear fold over a right-lateral C′ foliation. (a) SSW section with only right-lateral C′ foliation. (b) NNE section disturbed by left-lateral shear fold. (c) Detailed sketches and kinematic interpretation of a and b. The scale bar is 10 cm. Inset is stereo plot of shear zone boundary (black) and C′ foliation (red). The right-lateral C′ foliation results in extension and thinning of the layers, while the left-lateral shear fold results in contraction and thickening of the layers and counter-clockwise rotation of the C′ foliation. A partial reactivation along the C′ foliation has changed it into left-lateral sense. This structure indicates that the right-lateral shearing is followed by a left-lateral shearing.
반면 NNE측(그림 7b)의 경우 좌수향 전단습곡에 의해 셰일 우세부가 현저하게 변형되어 두꺼워진 양상을 보인다. 층의 형태는 7a에 비해 복잡한 형태를 띠고 있으며, C′ 엽리 또한 반시계방향의 회전을 겪어 상대적으로 낮은 δ 값을 보인다. 또한 우수향 C′ 엽리의 말단부에 좌수향의 변위를 기록하고 있다. 즉 그림 7a는 좌수향 전단습곡의 영향을 받지 않은 구역이며, 그림 7b는 우수향 C′ 엽리와 좌수향 전단습곡에 의한 변형이 중첩되어 있는 것으로 보인다. 전단대의 굴곡은 전단에 방해요소로 작용하기 때문에 C′ 엽리가 양쪽 모두 관찰되는 것은 전단습곡에 의한 두께변화 및 굴곡 이전에 발달한 구조임을 지시한다. 또한 C′ 엽리 말단부에서의 좌수향 변위에 대한 기록은 엽리가 우수향으로 발달한 이후 좌수향으로 재활성한 것을 지시한다. 이를 종합하면 좌수향 전단습곡은 우수향 C′ 엽리보다 후기에 발생한 변형이다.
4.2 운동학적 분석 결과
다른 변형사건(e.g., 좌수향 전단습곡)에 의한 오차를 최소화 하기 위해 다른 구조와 중첩되지 않는 우수향 전단지시자를 선별하여 이들의 자세를 통해 운동학적 특성을 정밀하게 분석하였다(그림 8). 그 결과 6개 지점(그림 2)에서 발견한 13개의 노두에 대해 δ를 측정하였으며 δ와 식 1을 이용하여 Wm을 산출하였다(표 1).
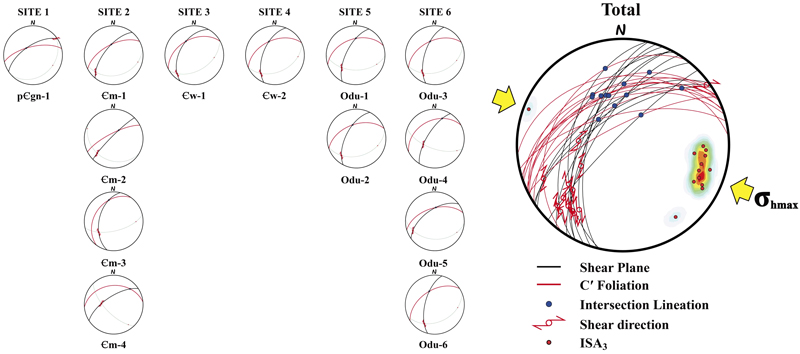
Summary of slip direction and ISA3 determined from C′ foliations and shear planes. The contour is for density of ISA3. The results indicate dominant right-lateral strike-slip movement with some reverse component in WNW-ESE compression.
연구지역 내 층리평행 전단구조의 전반적인 운동학적 특성 분석결과, C′과 전단면의 교차선구조는 주로 북쪽으로 침강하는 자세가 우세하게 나타났다. 교차선구조와 변형의 회전축인 소용돌이 벡터는 동일하므로(그림 3), 이를 통해 전단방향을 추론한 결과, 일부 역이동 성분을 포함하는 우수향 주향이동 전단이 우세한 것으로 나타났다(그림 8).
각 지점별 운동학적 특성은 다음과 같다. 지점 1은 옥동단층 하반의 고원생대 편마암으로 구성된 곳으로 Wm은 0.743으로 산출되었다. 지점 2는 묘봉층으로 구성되어 있으며 Wm은 0.738~0.813 사이로 나타났다. 지점 3 및 4는 화절층 분포지역으로 Wm은 0.235, 0.345로 산출된다. 지점 5, 6은 두무골층 분포지역으로 Wm은 0.170~0.466 사이 값을 보인다. 각 층별 평균 Wm은 묘봉층 - 0.768, 화절층 - 0.290, 두무골층 0.263이다.
두 유동아포피시스가 사교하는 준단순전단에서 순수전단의 비율은 α/90º로 정의된다(그림 9; Law et al., 2004). 연구지역의 Wm 산출결과를 바탕으로 순수전단의 비율을 구한 결과 화절층과 두무골층의 경우 순수전단의 비율이 69~89%이며, 고원생대 편마암과 묘봉층의 경우 40~47%로 나타났다(그림 9).
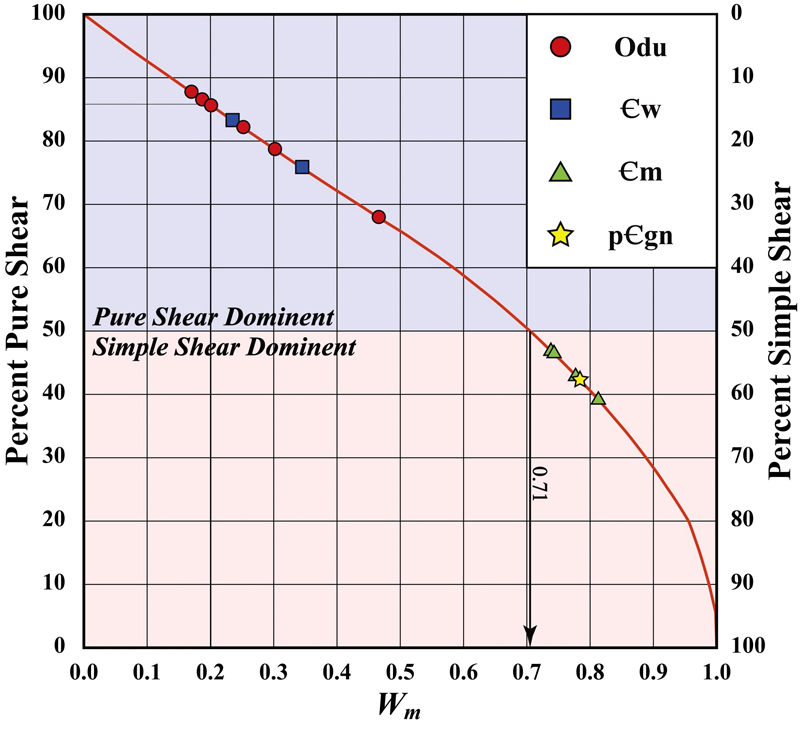
Plot of percent pure and simple shear as a function of Wm. Odu, Dumugol Formation; Єw, Hwajeol Formation; Єm, Myobong Formation; pЄgn, Pre-cambrian gneiss; Percent of pure shear is α/90º. For α=45º (Wk.=0.71), the ratio of pure to simple shear is the same. Pure shear ranges from 69% to 89% for the Dumugol Formation and Hwajeol Formation, where pure shear is dominant. The Myobong Formation and the Pre-cambrian Gneiss, where simple shear is dominant, also contain a high percentage of pure shear, ranging from 40% to 47%. This indicates that layer-parallel shear occurred in a transpressional environment (modified from the Law et al., 2004).
α를 이용한 고수렴각 산출결과는 다음과 같다. 옥동단층과 먼 화절층과 두무골층은 62.2~80.2º의 고각의 고수렴각을 가지며, 옥동단층과 가까운 묘봉층은 35.6~42.4º의 고수렴각을 갖는다(그림 10).
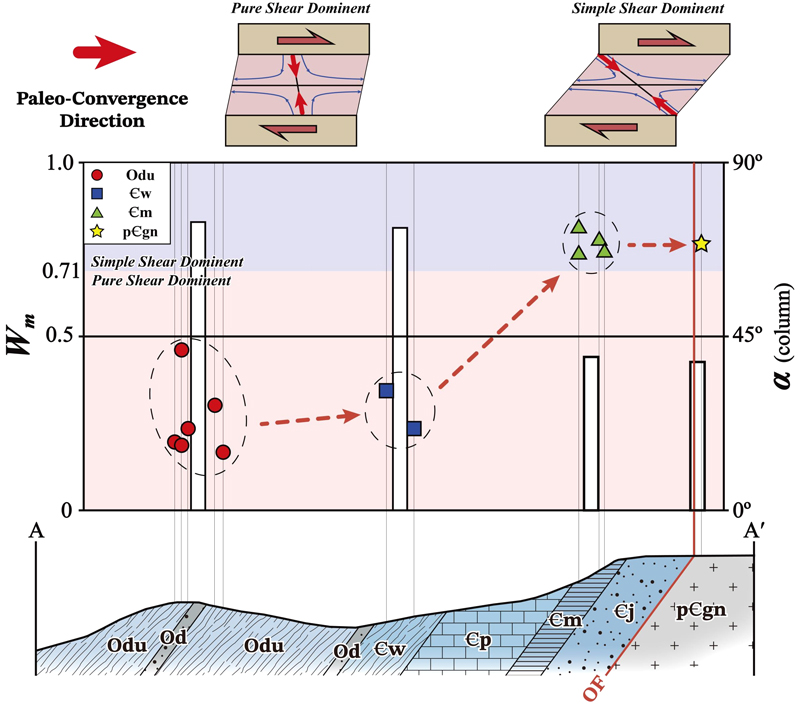
Plot of Wk and α by distance from the Okdong Fault. The dot plot is Wm and the column plot is the average α for each formation. The geological profile is A-A′ section in Figure 2. Odu, Dumugol Formation; Єw, Hwajeol Formation; Єp, Daegi Formation; Єm, Myobong Formation; Єj, Jangsan Formation; pЄgn, Pre-cambrian gneiss; Wm has low values in the Hwajeol and Dumugol Formation, and high values in the Pre-cambrian Gneiss and the Myobong Formation. In contrast, the α is high in the Hwajeol Formation and Dumugol Formation, and low in the Pre-cambrian Gneiss and Myobong Formation. α means the paleo-convergence angle. This result indicates that strain partitioning has occurred along the Okdong Fault.
5. 토 론
5.1 층리평행 전단구조의 성인
일반적으로 층리와 평행하게 전단운동이 일어나는 경우는 좌굴습곡의 요굴전단(flexural shear)이나, 단층이나 전단대가 층리에 규제되는 경우(예: 충상단층의 플랫-램프-플랫 구조; flat-ramp-flat), 그리고 중력에 의한 사태성 미끌림 등이 있다. 요굴전단의 경우 습곡의 양쪽 날개가 반대방향의 운동감각을 가지기 때문에 습곡 내 위치에 따라 다른 전단감각을 가지게 되며, 단층 및 전단대의 경우 단층의 전단방향을 따라 하나의 운동감각을 가지게 된다. 중력에 의한 사태성 미끌림의 경우 단층과 유사한 성질을 띄나 국부적으로 나타날 가능성이 높다. 연구지역의 경우 층리평행 전단구조가 넓은 지역에 분포하고 있으며 일관성 있는 자세와 운동감각을 지시하기 때문에 중력에 의한 사태는 배제하였다. 우수향과 좌수향 운동감각이 모두 나타나지만 두 변형구조의 기작이 다르고(우수향 - C′, 층의 박층화; 좌수향 - 전단습곡, 층의 두께증가), 두 구조를 제어하는 큰 습곡구조가 관찰되지 않은 점, 우수향 C′ 엽리를 좌수향 전단습곡이 변형시키고 있는 점으로 볼 때, 이 두 변형구조를 별개의 변형사건으로 해석하는 것이 타당하다(그림 7; 단락 4.1 참조). 즉 우수향을 지시하는 C′ 엽리가 발달한 이후 좌수향을 지시하는 전단습곡이 발달하였으며, 각각의 독립된 변형은 단층 또는 전단대에 의한 변형으로 해석된다.
Kim et al. (1989)은 옥동단층의 운동감각에 대해 좌수향 운동감각을 보이는 것으로 보고하였으나 현재 지배적인 견해는 우수향을 지시하는 전단대이다(e.g., Cluzel et al., 1991; Kim and Koh, 1992; Kwon et al., 1995). 본 연구 결과 옥동단층의 원압쇄암 노두에서 C′ 엽리의 배열을 통해 옥동단층의 운동감각이 우수향임을 재확인하였으며, 연구지역의 층리평행 전단구조 또한 우수향이 우세하게 나타나고 있음을 확인하였다. 즉, 옥동단층과 층리가 동일한 자세를 가지며 동일한 우수향 운동을 기록하고 있다.
연구지역의 Wm은 순수전단에 가까운 0.170에서 단순전단에 가까운 0.813 사이에서 다양하게 나타났다. 이를 옥동단층과의 거리에 따라 도시한 결과, 단층에서 멀리 떨어진 두무골층, 화절층의 경우 0.170~0.466 사이의 낮은 Wm 값을 보이고, 단층에 가까운 묘봉층은 0.783-0.813 사이의 높은 Wm 값을 보였다(표 1; 그림 10). 즉 두무골층과 화절층은 순수전단을 더 많이 수용하고, 옥동단층에 가까운 묘봉층은 단순전단 성분을 더 많이 수용하는 일종의 변형분할(strain partitioning) 양상을 보여주는 것으로 해석된다. 이러한 변형분할은 야외조사와 수치모델을 통해 보고된 바 있다(e.g., Jones and Tanner, 1995; Lin et al.,1998; Ježek et al., 2002; Zhang et al., 2009; Kuiper et al., 2011). Ježek et al. (2002)은 수치모델을 통해 두 판이 사교하며 수렴하는 전달압축 환경에서 변형영역이 판경계에 가까운 단순전단 우세영역(wrench dominated, WD)과 판경계에서 비교적 떨어진 위치의 순수전단 우세영역(pure shear dominated, PSD)으로 분리되는 변형분할 양상이 일반적으로 나타날 수 있다고 제안하였다. 전단대 내에서 단순전단은 변형연화(strain softening)를 겪지만, 순수전단은 전단대 방향과 평행한 신장을 일으키기 때문에 공간문제를 발생시키고 이로 인해 변형경화(strain hardening)를 겪는다(Lin et al., 1998; Kuiper et al., 2011). 변형연화작용은 변형을 좁은 구역에 집중시키고 변형경화작용은 변형을 넓은 영역으로 분산시킨다(Fossen and Cavalcante, 2017). 즉 변형연화를 겪는 단순전단 우세영역은 전단대의 중심부에 좁은 영역으로 발달하게 되고 변형경화를 겪는 순수전단 우세영역은 전단대의 가장자리에 분포하게 된다. 따라서 층리평행 전단구조의 Wm가 옥동단층과 가까워질수록 증가하는 양상을 보이는 것은 전단변형의 중심부가 옥동단층이라는 것을 지시한다.
연구지역에서 관찰된 좌수향 전단습곡의 경우 Kim et al. (1989)이 보고한 옥동단층의 좌수향 운동감각과 관련되어 있을 가능성이 있으나 이에 대해서는 추가적인 연구가 필요할 것으로 사료된다.
5.2 층리평행 전단구조의 지체구조적 의미
층리평행 전단구조가 옥동단층의 우수향 전단운동에 의한 것이라면, 해당구조의 운동학적 특성은 지체구조적으로 중요한 의미를 가질 수 있다. 먼저 C′ 엽리와 전단면의 교차선구조를 통해 도출한 전단방향은 우수향-역이동성 사교이동을 지시하고 있으며, 앞선 토론에서 언급하였듯이 옥동단층을 따라 단순전단 구역과 순수전단 구역이 나뉘는 변형분할양상이 나타난다. 특히 순수전단 구역에 해당하는 화절층과 두무골층의 경우 순수전단 비율이 69~89%에 달하며, 단순전단 구역에 해당하는 고원생대 편마암과 묘봉층 또한 40~47%의 높은 순수전단 비율을 보였다. 이러한 높은 순수전단 비율은 전단면에 수직한 압축성분이 높았음을 지시한다.
또한 C′, 전단면 및 교차선구조의 자세와 식 4를 통해 ISA3를 도출할 수 있다. 그 결과 층리평행 전단구조의 평균 ISA3 자세는 26º, 109º로 도출되었다(그림 8). 실제로 응력변형의 방향을 지시하는 ISA와 응력의 방향을 지시하는 주응력축(principal stress axis)은 정확히 일치하지 않으며 두 용어를 동일하게 취급하기 위해서는 정상상태유동(steady-state rheology)이 가정되어야 한다(Tikoff and Fossen, 1995). 그럼에도 불구하고 ISA와 주응력축 간 유사성을 바탕으로 ISA를 통해 주응력축을 대략적으로 유추할 수 있다. 다시 말해, ISA3와 최대주응력(σ1)은 유사한 방향일 가능성이 높다. 이를 토대로 당시 고응력장을 추론한 결과 옥동단층의 우수향 전단운동과 층리평행 전단구조는 서북서-동남동 압축응력 하에서 발달하였음을 지시한다. 이러한 결과는 옥동단층 및 연구지역의 일반적인 층리자세(북동-남서)와 60º 이상의 고각을 이루며, 전달압축환경을 지시한다.
5.3 옥동단층의 우수향 전단운동 시기 및 각동충 상단층과 옥동단층의 변형분할에 대한 고찰
옥동단층 우수향 전단운동의 활동연대는 Yun (1983)이 보고한 옥동지역 장산층과 고원생대 편마암 사이에 위치한 견운모 편암(이후 압쇄암으로 재해석됨; Kim et al., 1989)에 대한 세 개의 K-Ar 연대 562±5, 266±5, 235±5 Ma로 알려져 있다. Kim et al. (1989)은 위 연대를 바탕으로 옥동단층이 여러 번 재활성을 겪었으며, 이 중 최후기인 235±5 Ma가 옥동단층 연성전단운동의 최후기 사건을 지시한다고 해석하고 이를 송림조산운동에 대비하였다. 옥동단층과 각동충상단층에 대한 후속연구는 위 해석에 바탕을 두고, 옥동단층이 송림조산운동 시기 우수향 주향이동을 겪은 후 대보조산운동 시기에 충상단층으로 재활성한 것으로 해석되어 왔다(e.g., Kim and Koh, 1992; Kim et al., 1994).
하지만 Kwon et al. (1995)은 Yun et al. (1983)의 연대결과가 Ar 과잉의 가능성이 있기 때문에 해당 연대로 옥동단층의 운동을 해석하는 것에 대해 의문을 제기하였으며, Kim and Park (2018)은 위의 견해에 바탕을 두고 Yun et al. (1983)의 자료를 재검토하였다. Kim and Park (2018)에 따르면 Yun et al. (1983)이 도출한 세 연대는 하나의 시료에서 나온 결과이기 때문에 세 번의 옥동단층 활동연대를 지시하는 것으로 보기 힘들고, 세 연대가 170 Ma의 연대를 지시하는 선상에 배치되기 때문에 Yun et al. (1983)의 시료는 약 170 Ma를 지시한다고 주장하였다. 해당 연대는 쥐라기 중기 대보조산운동 시기에 해당하며, 즉, Kim and Park (2018)의 수정연대를 신뢰한다면 옥동단층의 우수향 연성전단운동은 반송층군 퇴적(187 Ma; Han et al., 2006) 전후에 해당하는 대보조산운동시기로 해석할 수 있다.
이러한 기존 옥동단층 우수향 전단운동에 대한 연대측정결과의 낮은 신뢰성과 오차는 옥동단층 및 옥천대의 변형사 해석에 혼란을 줄 수 있다. 따라서 주요 구조선에 대한 운동학적 해석이 중요하다. 따라서 본 연구를 통해 도출한 옥동단층의 운동학적 특성을 이용하여 옥동단층의 시기와 다른 구조선과의 관계에 대해 고찰하고자 한다.
옥동단층과 각동충상단층은 유사한 자세를 가지고 병치되어 있는 구조이기 때문에 두 단층이 동일한 대보조산운동시기에 활동하였다면 동일한 응력장에서 발생하였을 가능성이 높다. Kim et al. (1992b)은 연구지역 인근에 위치한 영춘지역의 각동충상단층 및 대보조산운동에 의한 구조들에 대해 응력텐서 분석을 실시하였다. 그 결과 응력텐서는 WNW-ESE의 압축응력장을 지시하는 것으로 나타났다. 이는 본 논문에서 도출한 옥동단층 및 층리평행 전단구조에 대한 응력방향과 동일하다. 옥동단층과 각동충상단층이 유사한 고응력장 내에서 발달하였음은 이 두 구조선이 유사한 시기에 발달하였을 가능성이 높음을 지시한다.
각동충상단층의 기 보고된 운동감각은 역이동(Top-to-the-southeast; Kim et al., 1994)으로 기존 연구와 본 연구결과에서 도출된 옥동단층의 운동감각인 우수향 주향이동과 차이가 있다. 하지만 본 연구 결과, 옥동전단대의 우수향 전단구조가 전달압축환경에서 변형분할의 특성을 보여주며, 옥동단층과 멀어질수록 고수렴각이 각동충상단층의 운동감각과 유사한 WNW-ESE방향으로 회전하는 양상을 보여준다. 이는 옥동단층과 각동충상단층이 운동학적으로 연관성이 있을 가능성을 보여주며, 비록 추가적인 연구가 필요하겠지만 두 단층이 동시기에 변형분할을 통해 활동하였을 가능성을 시사한다.
두 단층이 유사한 시기에 다른 운동학적 특성을 가지게 된 원인은 두 단층의 기하학적 특성 차이 때문일 수 있다. Kim et al. (1994)은 각동충상단층이 옥동단층에 비해 저각의 경사를 가지는 것으로 보고하였다. 일반적으로 역단층은 저각의 경사를 가지며 발생하기 때문에 상대적으로 경사각이 낮은 각동충상단층은 역단층으로 활성화될 수 있지만, 경사각이 큰 옥동단층은 역단층으로 활성화되기 힘들다. 실제로 지구상의 여러 전달압축환경에서 변형분할에 의해 동일한 주향을 가지며, 저각의 경사를 가지는 충상단층과 고각의 경사를 가지는 주향이동단층이 동시에 발달하는 경우가 다수 보고되어 있다(e.g., 산 안드레아스 단층, Lettis and Hanson, 1991; 뉴질랜드 알파인 단층, Jarrard, 1988; 알류샨 열도, Ryan and Scholl, 1989, 1993; 타이완, Ho et al., 2022 등). 따라서 옥동단층과 각동충상단층에 대해 동일한 해석을 적용하는 것은 큰 무리가 아닐 것이다.
따라서 우리는 본 연구 결과를 토대로 옥동단층과 각동충상단층의 변형분할 모델을 제시하고자 한다(그림 11). 해당 모델에서 NE-SW방향의 경계를 따라 WNW-ESE방향의 압축응력이 작용하여, 경사각이 작은 북서측의 각동충상단층은 역단층으로, 경사각이 큰 남동측의 옥동단층은 우수향 주향이동 전단대로 활성화되었던 것으로 해석된다. 이 과정에서 WNW-ESE방향의 압축응력은 NW-SE방향의 단축(shortening)과 NE-SW방향의 전단응력변형으로 분할되며, NW-SE방향의 단축은 각동충상단층과 옥동단층의 순수전단 우세영역에서 수용되고, NE-SW방향의 전단응력변형은 옥동단층의 단순전단 우세영역에서 수용되는 것으로 해석된다(그림 11).
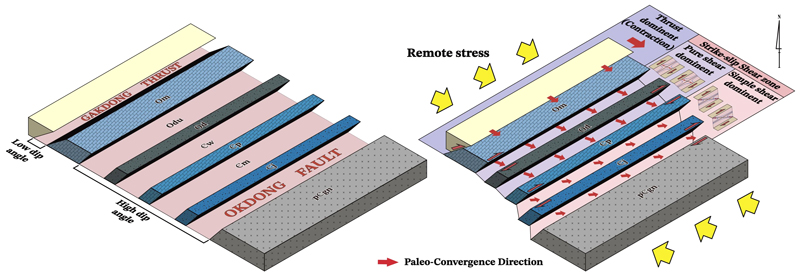
Block diagram of strain partitioning across the Okdong Fault and Gakdong Thrust. Om, Makgol Formation; Odu, Dumugol Formation; Єw, Hwajeol Formation; Єp, Daegi Formation; Єm, Myobong Formation; Єj, Jangsan Formation; pЄgn, Pre-cambrian gneiss; The Okdong Fault has a high dip angle, and the northwestern Gakdong Thrust has a low dip angle. Under a WNW-ESE compressional stress field, the low-angle thrusts moved with a reverse slip sense, and the high-angle Okdong Fault moved with a right-lateral strike-slip sense.
해당 모델의 검증을 위해 더 많은 운동학적, 암석학적 연구가 수반되어야 하겠지만 해당모델은 기존에 복잡하게 설명되어 온 단양지역의 변형사를 단순하고 명료하게 설명할 수 있다는 장점을 가진다. 또한 이러한 접근과 해석은 옥천대 내에 분포하고 있는 다른 구조선들의 해석에도 적용할 수 있을 것으로 생각된다.
6. 결 론
본 연구는 단양지역 층리평행 전단구조의 운동학적 특성과 해당 구조의 지체구조적 특징을 규명하기 위해 수행되었다. 이를 위해 야외조사를 통해 전단지시자를 식별하고, 층리평행 전단구조에 대해 운동학적 특성을 분석하였으며, 그 결과는 다음과 같다.
1) 단양지역 옥동단층의 상반에 위치한 묘봉층, 화절층, 두무골층에는 우수향 및 좌수향 전단을 지시하는 층리평행 전단구조가 발달하고 있으며, 옥동단층의 전단운동에 의한 변형을 일부 수용하고 있는 것으로 판단된다.
2) 우수향 층리평행 전단구조에 대해 운동학적 특성 분석결과 Wm의 경우 옥동단층과 가까운 묘봉층은 0.738~0.813으로 단순전단이 우세하지만, 옥동단층과 먼 화절층과 두무골층은 0.170~0.466 사이의 값으로 순수전단이 우세하다. 또한 Wm을 통해 파악한 층리평행 전단구조의 평균 ISA3 자세는 26º, 109º로 서북서-남남동 압축을 지시한다. 이는 옥동단층의 우수향 전단운동 시 주 전단대에 사교하는 응력(WNW-ESE 압축)에 대한 변형분할을 지시하는 것으로 보이며, 옥동단층의 우수향 전단운동과 해당구조가 전달압축환경에서 발달하였음을 지시하는 것으로 해석된다.
3) Wm을 통해 파악한 옥동전단대 내 고수렴각은 옥동단층과 멀어질수록 WNW-ESE에서 NW-SE방향으로 회전하는 것으로 나타났다. 이는 각동충상단층과 옥동단층의 전단운동에 의한 지괴의 수렴방향을 잘 반영하는 것으로 해석되며, 옥동단층의 우수향 전단운동과 각동충상단층이 변형분할을 통해 운동학적으로 긴밀한 연관성을 가지며 발달하였을 가능성을 시사한다.
그러나 본 연구에서 밝혀진 옥동단층의 변형분할 양상이 다른 구조선들과 어떤 관계를 보이는지는 아직 확실하지 않으며 이에 대한 추가적인 연구가 필요할 것으로 사료된다.
옥동단층을 비롯한 여러 대형구조선의 운동학적 특성 규명은 한반도 지체구조운동의 비밀을 푸는 중요한 열쇠가 될 수 있다. 그러나 2차구조인 단층의 운동시기에 대한 절대연대측정에는 많은 어려움이 따른다. 다양한 방법에 의해 단층이나 전단대의 형성연대를 추정하는 방법들이 제시되었지만, 일반적으로 단층운동의 연대는 단층에 의해 절단된 지층에 의한 상대연령에 의존하게 된다. 따라서 단층운동의 해석을 연대측정에 의존하는 데는 많은 주의가 필요하며, 측정된 연대가 지시하는 지질학적 의미에 대해서도 신중한 고민이 필요하다. 따라서 단층의 해석에는 야외자료를 바탕으로 한 운동학적 특성에 대한 명확한 규명이 선행되어야 하며, 운동학적 특성을 기반으로 절대연대를 활용한 적절한 해석이 수반되어야 한다. 본 연구가 한반도 대형구조선의 운동학적 특성을 해석하는데 새로운 도전과 시도가 되기를 희망한다.
Acknowledgments
이 논문은 부경대학교 자율창의학술연구비(2021년)에 의하여 연구되었습니다. 논문을 쓰는데 많은 도움을 주신 GSGR 식구들과 서울대학교 김정환 명예교수님 및 남호현 사장님께 감사드립니다. 그리고 논문에 좋은 조언을 주신 두 분의 심사위원분께 감사드립니다.
References
-
Chough, S.K., Kwon, S.-T., Ree, J.H. and Choi, D.K., 2000, Tectonic and sedimentary evolution of the Korean Peninsula-a review and new view. Earth Science Reviews, 52, 175-235.
[https://doi.org/10.1016/S0012-8252(00)00029-5]
-
Cluzel, D., Lee, B.J. and Cadet, P.J., 1991, Indosinian dextral ductile fault system and synkinematic plutonism in the southwest of the Ogcheon Belt (South Korea). Tectonophysics, 194, 131-151.
[https://doi.org/10.1016/0040-1951(91)90277-Y]
-
Fossen, H., 2016, Structural Geology. Cambridge University Press, Cambridge.
[https://doi.org/10.1017/9781107415096]
-
Fossen, H. and Cavalcante, G.C.G., 2017, Shear zones - a review. Earth Science Reviews, 171, 434-455.
[https://doi.org/10.1016/j.earscirev.2017.05.002]
-
Fossen, H. and Tikoff, B., 1997, Forward modeling of non-steady-state deformations and the ‘minimum strain path’. Journal of Structural Geology, 19, 987-996.
[https://doi.org/10.1016/S0191-8141(97)00021-7]
-
Fossen, H. and Tikoff, B., 1998, Extended models of transpression/ transtension and application to tectonic settings. Geological Society Special Publication, 135, 15-33.
[https://doi.org/10.1144/GSL.SP.1998.135.01.02]
-
Gillam, B.G., Little, T.A., Smith, E. and Toy, V.G., 2013, Extensional shear band development on the outer margin of the Alpine mylonite zone, Tatare Stream, Southern Alps, New Zealand. Journal of Structural Geology, 54, 1-20.
[https://doi.org/10.1016/j.jsg.2013.06.010]
-
Goscombe, B.D. and Passchier, C.W., 2003, Asymmetric boudins as shear sense indicators-an assessment from field data. Journal of Structural Geology, 25, 575-589.
[https://doi.org/10.1016/S0191-8141(02)00045-7]
-
Graziani, R., Larson, K.P., Law, R.D., Vanier, M.-A. and Thigpen, J.R., 2021, A refined approach for quantitative kinematic vorticity number estimation using microstructures. Journal of Structural Geology, 153, 104459.
[https://doi.org/10.1016/j.jsg.2021.104459]
-
Han, R.H., Ree, J., Cho, D., Kwon, S. and Armstrong, R., 2006, SHRIMP U-Pb zircon ages of pyroclastic rocks in the Bansong Group, Taebaeksan Basin, South Korea and their implication for the Mesozoic tectonics. Gondwana Research, 9, 106-117.
[https://doi.org/10.1016/j.gr.2005.06.006]
-
Hanmer, S., 1986, Asymmetrical pull-aparts and foliation fish as kinematic indicators. Journal of Structural Geology, 8, 111-122.
[https://doi.org/10.1016/0191-8141(86)90102-1]
-
Ho, G.R., Byrne, T.B., Lee, J.C., Mesalles, L., Lin, C.W., Lo, W. and Chang, C.P., 2022, A new interpretation of the metamorphic core in the Taiwan orogen: A regional-scale, left-lateral shear zone that accommodated highly oblique plate convergence in the Plio-Pleistocene. Tectonophysics, 833, 229-332.
[https://doi.org/10.1016/j.tecto.2022.229332]
-
Jarrard, R.D., 1986, Relations among subduction parameters: Reviews of Geophysics. 24, 217-284.
[https://doi.org/10.1029/RG024i002p00217]
-
Ježek, J., Schulmann, K. and Thompson, A.B., 2002, Strain partitioning in front of an obliquely convergent indenter. In Bertotti, G., Schulmann, K. and Cloethingh, S., editors, Continental collision and the tectonosedimentary evolution of forelands: European Geophysical Society, Stephan Mueller Special Publication Series, 1, 93-104.
[https://doi.org/10.5194/smsps-1-93-2002]
-
Jones, R.R. and Tanner, P.W.G., 1995, Strain partitioning in transpression zones. Journal of Structural Geology, 17, 793-802.
[https://doi.org/10.1016/0191-8141(94)00102-6]
- Kee, W.-S., Kim, S.W., Kim, H., Hong, P., Kwon, C.W., Lee, H.-J., Cho, D.-L., Koh, H.J., Song, K.-Y. and Byun, U.H., 2019, Geological Map of Korea (1:1,000,000). Korea Institute of Geoscience and Mineral Resources.
- Kim, J.H. and Koh, H.-J., 1992, Structural analysis of the Danyang area, Danyang coalfield, Korea. Economic and Environmental Geology, 25, 61-72.
- Kim, J.H., Koh, H.-J. and Kee, W.S., 1989, The Okdong Fault. Journal of Korean Institute of Mining Geology, 22, 285-291 (in Korean with English abstract).
- Kim, J.H. and Lee, J.D., 1991, Geological Structures of the Yemi Area, Kangweon-do, Korea. Journal of the Geological Society of Korea, 27, 500-514 (in Korean with English abstract).
- Kim, J.H., Lee, J.Y. and Nam, K.H., 1992a, Geological Structures of the Yeongchun Area, Danyang Coalfield, Korea. Journal of Korean Institute of Mining Geology, 25, 179-190.
- Kim, J.H., Lee, J.Y. and Nam, K.H., 1994, Pre-Jurassic Thrust Movement in Danyang Coalfield, Korea. Journal of the Geological Society of Korea, 30, 35-40 (in Korean with English abstract).
- Kim, J.H., Lee, J.Y., Nam, K.H. and Choi, P.-Y., 1992b, Paleostress Analysis within the Thrust Zone, Yeongchun, the Danyang Coalfield, Korea. Journal of the Geological Society of Korea, 28, 184-195.
- Kim, M.J. and Park, K.-H., 2018, A review on the K-Ar Ages of Quartz Schist in the Okdong Fault Zone - Robust Enough for the Evidence for the Precambrian Deposition of the Jangsan Formation?. Journal of the Petrological Society of Korea, 27, 67-72 (in Korean with English abstract).
- Kobayashi, T., 1966, Stratigraphy of the Chosen Group in Korea and South Manchuria and its relation to the Cambro-Ordovician formation of other areas, Section A, The Chosen Group of South Korea. Journal of the Faculty Science (University of Tokyo), Section II, 16, 1-84.
-
Kuiper, Y.D., Lin, S. and Jiang, D., 2011, Deformational partitioning in transpressional shear zones with an along-strike shear component: an example from the superior boundary zone, Manitoba, Canada. Journal of Structural Geology, 33, 192-202.
[https://doi.org/10.1016/j.jsg.2010.07.003]
-
Kurz, G.A. and Northrup, C.J., 2008, Structural analysis of mylonitic fault rocks in the Cougar Creek Complex, OregoneIdaho using the porphyroclast hyperbolic distribution method, and potential use of SC′-type extensional shear bands as quantitative vorticity indicators. Journal of Structural Geology, 30, 1005-1012.
[https://doi.org/10.1016/j.jsg.2008.04.003]
-
Kwon, S., Sajeev, K., Mitra, G., Park, Y., Kim, S.W. and Ryu, I.-C., 2009, Evidence for Permo-Triassic collision in Far East Asia: The Korean collisional orogen. Earth and Planetary Science Letters, 279, 340-349.
[https://doi.org/10.1016/j.epsl.2009.01.016]
- Kwon, S.-T., Lee, J.-H., Park, K.-H. and Jeon, E.-Y., 1995, Nature of contact between the Ogcheon belt and Yeongnam massif and the Pb-Pb age of granitic gneiss in Cheondong-ri Danyang. Journal of the Petrological Society of Korea, 4, 144-152 (in Korean with English abstract).
-
Law, R.D., Searle, M.P. and Simpson, R.L., 2004, Strain, deformation temperatures and vorticity of flow at the top of the Greater Himalayan Slab, Everest Massif, Tibet. Journal of the Geological Society, London, 161, 305-320.
[https://doi.org/10.1144/0016-764903-047]
-
Lettis, W.R. and Hanson, K.L., 1991, Crustal strain partitioning: Implications for seismic hazard assessment in western California. Geology, 19, 559-562.
[https://doi.org/10.1130/0091-7613(1991)019<0559:CSPIFS>2.3.CO;2]
-
Li, J., Wang, T. and Ouyang, Z., 2010, Strain and kinematic vorticity analysis of the Louzidian low-angle ductile shear detachment zone in Chifeng, Inner Mongolia, China. Science China Earth Sciences, 53, 1611-1624.
[https://doi.org/10.1007/s11430-010-4041-9]
- Lim, S.-B., Chun, H.Y., Kim, Y.B., Kim, C.B. and Cho, D.-L., 2005, Geologic ages, stratigraphy and geological structures of the metasedimentary strata in Bibong∼Yeonmu area, NW Okcheon belt, Korea. Journal of the Geological Society of Korea, 41, 335-368 (in Korean with English abstract).
-
Lin, S., Jiang, D. and Williams, P.F., 1998, Transpression (or transtension) zones of triclinic symmetry: Natural example and theoretical modelling. Continental transpressional and transtensional tectonics: Geological Society, London, Special Publication, 135, 41-57.
[https://doi.org/10.1144/GSL.SP.1998.135.01.04]
-
McKenzie, D., 1979, Finite deformation during fluid flow. Geophysical Journal International, 58, 689-715.
[https://doi.org/10.1111/j.1365-246X.1979.tb04803.x]
-
Means, W.D., Hobbs, B.E., Lister, G.S. and Williams, P.F., 1980, Vorticity and non-coaxiality in progressive deformations. Journal of Structural Geology, 2, 371-378.
[https://doi.org/10.1016/0191-8141(80)90024-3]
-
Ni, J.L., Liu, J.L., Sun, Y., Shi, X. and Shen, L.I., 2019, The characteristics of the structure, strain and kinematic vorticity of the Wulian detachment fault zone, Shandong peninsula, China. Science China Earth Sciences, 62, 1399-1415.
[https://doi.org/10.1007/s11430-018-9319-9]
-
Oh, C.H. and Kim, Y.H., 2021, The geological correlation between the Korean Peninsula and China from Paleoproterozoic to Triassic and the comparative evaluation among the Permo-Triassic collision models in the Northeast Asia. Journal of the Geological Society of Korea, 57, 369-412 (in Korean with English abstract).
[https://doi.org/10.14770/jgsk.2021.57.4.369]
-
Passchier, C.W., 1987, Stable positions of rigid objects in non-coaxial flow-a study in vorticity analysis. Journal of Structural Geology, 9, 679-690.
[https://doi.org/10.1016/0191-8141(87)90152-0]
-
Passchier, C.W., 1997, The fabric attractor. Journal of Structural Geology 19, 113-127.
[https://doi.org/10.1016/S0191-8141(96)00077-6]
- Passchier, C.W. and Trouw, R.A.J., 2005, Microtectonics. Springer Science & Business Media.
-
Ryan, H.F. and Scholl, D.W., 1989, The evolution of forearc structures along an oblique convergent margin, central Aleutian arc. Tectonics, 8, 497-516.
[https://doi.org/10.1029/TC008i003p00497]
-
Ryan, H.F. and Scholl, D.W., 1993, Geologic implications of great interplate earthquakes along the Aleutian arc. Journal of Geophysical Research, 98, 22135-22146.
[https://doi.org/10.1029/93JB02451]
-
Simpson, C. and De Paor, D.G., 1993, Strain and kinematic analysis in general shear zones. Journal of Structural Geology, 15, 1-20.
[https://doi.org/10.1016/0191-8141(93)90075-L]
-
Sullivan, W.A., 2009, Kinematic significance of L tectonites in the footwall of a major terrane-bounding thrust fault, Klamath Mountains, California, USA. Journal of Structural Geology, 31, 1197-1211.
[https://doi.org/10.1016/j.jsg.2009.06.016]
-
Tikoff, B. and Fossen, H., 1995, The limitations of three-dimensional kinematic vorticity. Journal of Structural Geology, 17, 1771-1784.
[https://doi.org/10.1016/0191-8141(95)00069-P]
- Won, C.-G. and Lee, H.-Y., 1967, Geological map of the Danyang sheet (1:50,000). Korea Institute of Geoscience and Mineral Resources, Daejeon.
-
Xypolias, P., 2010, Vorticity analysis in shear zones: a review of methods and applications. Journal of Structural Geology, 32, 2072-2092.
[https://doi.org/10.1016/j.jsg.2010.08.009]
- Yun, H.-S., 1983, K/Ar ages of micas from Precambrian and Phanerozoic rocks in the northeastern part of the Republic of Korea. Schweizerische Mineraligusche und Petrogrphische Mitteilungen (Swiss Bulletin of Mineralogy and Petrology), 63, 295-300.
-
Zhang, B., Zhang, J., Zhong, D. and Guo, L., 2009, Strain and kinematic vorticity analysis: An indicator for sinistral transpressional strain-partitioning along the Lancangjiang shear zone, western Yunnan, China. Science in China Series D: Earth Sciences, 52, 602-618.
[https://doi.org/10.1007/s11430-009-0065-4]