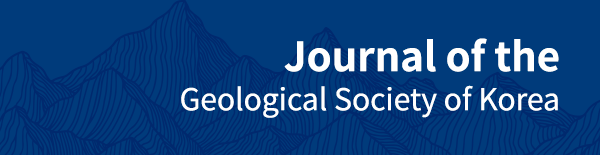
다검출기 유도결합 플라즈마 질량분석기를 이용한 미량시료의 황 동위원소 분석
초록
황은 다양한 생지구화학 반응에 전자 수용체 혹은 공여체로 참여하며, 그 과정에서 발생하는 안정 동위원소비의 변화는 지구물질 순환을 이해하기 위한 도구로 폭넓게 이용된다. 황 동위원소 분석에는 기체 시료를 이용한 측정이 주류를 이뤄왔으나 최근 다검출기 유도결합 플라즈마 질량분석기(MC-ICP-MS)가 액상 혹은 고체상 미량시료 분석을 중심으로 주목받고 있는데, 현재까지 국내에서 관련 분석 사례는 보고되지 않았다. 이번 연구에서는 서울대학교 공동기기원에 운영 중인 MC-ICP-MS를 이용해 황 동위원소 분석을 수행하였다. 시료 내 황산이온은 음이온 교환수지를 이용해 분리하였으며, 분자이온의 교란효과를 줄이고자 탈용매화장치를 통해 MC-ICP-MS에 주입하였다. 황 동위원소는 질량간섭의 영향을 받지 않는 S+ 피크의 가벼운 질량 말단부에서 측정되었으며, 표준물질과 시료의 교차분석을 통한 보정이 이루어졌다. 자체 표준물질의 동위원소 조성은 IAEA-SO-5와 비교를 통해 결정하였고, 이를 이용해 측정한 IAEA-SO-6와 NBS-127의 동위원소 조성은 보고된 값과 오차범위 내에서 일치하였다. 이번 연구를 통해 확인한 δ34S와 Δ33S 분석의 재현성은 각각 0.44‰과 0.52‰ 이하였으며, 최소 14 nmol의 황을 함유하고 있는 시료에서 안정적인 결과를 획득할 수 있었다. 미량시료의 황 동위원소 분석이 국내에서 가능해짐에 따라, 전통적인 분석을 위한 시료 확보에 어려움이 있었던 빙하나 미세먼지 등에 대한 국내 황 동위원소 연구가 활발해질 것으로 기대된다.
Abstract
Sulfur is a versatile element with valance states ranging from +6 to -2 and thereby involved in diverse geochemical and biological processes as an electron donor or acceptor. Since these reactions are often accompanied by fractionation of its four stable isotopes (32S, 33S, 34S, 36S), their relative abundance has been used to trace the sulfur cycle at a range of temporal and spatial scales. While the use of Multi Collector Inductively Coupled Plasma Mass Spectrometer (MC-ICP-MS) has emerged as a promising approach for measuring the triple sulfur isotope ratios of samples with small amounts of sulfate, MC-ICP-MS has never been used for sulfur isotope analysis in South Korea. Here we first present the results of triple sulfur isotope analysis using a MC-ICP-MS installed in the Inter-university Research Facilities at Seoul National University. To minimize isobaric interferences, sulfate is separated from matrix cations by anion exchange resin and introduced into MC-ICP-MS via desolvator, and sulfur isotopes are measured as S+ ions on the low-mass shoulder of the aligned peaks in medium resolution mode (M/ΔM* ~ 8000). Standard-sample bracketing is used to correct instrumental mass bias, and the sulfur isotope ratio of the in-house bracketing standard is evaluated by comparing it with the international reference material IAEA-SO-5. Typical reproducibility (2σ) for δ34S and Δ33S analyses is better than 0.44‰ and 0.57‰, respectively, and the sulfur isotope ratios of the reference materials IAEA-SO-6 and NBS-127, determined against the in-house bracketing standard, agree well with the reported values within errors. Although purification and isotope analysis of the samples containing down to 14 nmol sulfate achieves consistent results, great care to avoid contamination is needed when the sample contains only a few nmols of sulfate. With the new methodology being available, sulfur isotope studies in South Korea could be further extended to samples with lower sulfur contents, such as meteoric water, ice cores, and aerosols.
Keywords:
sulfur isotope, sulfate, MC-ICP-MS, isotope fractionation키워드:
황 동위원소, 황산이온, 다검출기 유도결합 플라즈마 질량분석기, 동위원소 분별작용1. 서 론
황은 지구를 구성하는 원소 중 아홉 번째로 풍부한 원소로서 황화수소(-II)에서 황산염(VI)에 이르는 넓은 범위의 산화상태를 지닌다. 황을 기반으로 하는 산화환원 반응은 미생물의 혐기성 호흡에서부터 황철석의 매장에 따른 대기 중 산소 농도 증가까지, 다양한 시간과 규모에서 지구물질 순환에 중요한 역할을 담당한다(Fike et al., 2015). 황은 32S (95%), 33S (0.75%), 34S (4.2%), 36S (0.017%)의 네 가지 안정 동위원소를 지니는데, 자연에서 황을 포함하는 물질의 동위원소 비율은 미생물 대사작용을 비롯한 물리화학적 반응을 통해 변화하며 황의 거동을 이해하기 위한 중요한 정보를 제공해 준다. 가장 풍부한 안정 동위원소인 32S와 34S의 분포는 자연계에서 70‰을 넘어서는 넓은 범위에서 변화하는데(Sim et al., 2011a), 이는 동위원소 간 질량 차이와 원자량의 상대적인 비율을 고려했을 때 수소나 탄소와 같은 원소를 넘어서는 가장 큰 규모의 동위원소 분별작용에 해당한다(Johnston and Fischer, 2012). 안정 동위원소 연구의 태동기인 1950년대부터 넓은 범위의 34S/32S 동위원소 분별작용을 이해하고 이를 지질학에 응용하는 연구가 지속되어 왔는데, 2000년대 초 황 동위원소 사이의 질량 차에서 예상되는 관계를 크게 벗어나는 다중 황 동위원소 분별작용이 시생누대의 퇴적암에서 보고된 이후 미량으로 분포하는 동위원소인 33S와 36S에 대한 연구 또한 활기를 띄게 되었다(Farquhar et al., 2000).
황 동위원소 분석에는 열이온화 질량분석기(Mann and Kelley, 2005), 레이저분광기(Christensen et al., 2007) 등을 활용한 다양한 질량분석법이 보고되었고 이차이온질량분석기를 이용한 표면분석(in-situ) 분석 또한 활발해지고 있지만(LaFlamme et al., 2016), 전통적으로 기체시료를 이용한 동위원소 질량분석법(gas-source IRMS)이 그 중심을 이뤄왔다. 기체시료를 이용한 황 동위원소 분석에는 시료를 이산화황(SO2) 혹은 육불화황(SF6)으로 변환시키는 전처리 과정이 요구된다. 이산화황을 이용한 분석은 현재 가장 대중적으로 이용되며 34S/32S를 0.2‰ 수준의 정확도로 측정할 수 있지만 이산화황 내 산소 동위원소의 영향을 보정하는 과정에서 발생하는 오차로 인해 33S와 같은 미량 동위원소 연구에는 한계가 존재한다(Rees et al., 1978). 이와 비교해 육불화황은 불소가 단일 동위원소로 구성된 원소이기 때문에 다른 동위원소의 간섭 없이 미량으로 존재하는 33S와 36S의 분석에서 장점을 지니지만(Hulston and Thode, 1965), 불화과정에 소요되는 시간과 유독성 물질을 이용한 전처리 과정 등의 요인으로 다중 황 동위원소 분석에 특화된 소수의 연구실에서 이용되고 있다. 최근 황 동위원소 연구에서 유도결합 플라즈마 질량분석기(ICP-MS)를 이용한 분석이 많은 주목을 받고 있는데, 수백 나노 몰 이상의 시료가 요구되는 기체시료 기반의 동위원소 분석법에 비해, 수십 나노 몰 혹은 그 이하 수준의 미량시료에서도 신뢰도 있는 분석이 가능하기 때문이다. 유도결합 플라즈마 질량분석기를 이용한 황 동위원소 분석의 정확성은 다검출기의 사용과 자기장과 전기장 분석기를 이용한 이중 초점 방식, 표준물-시료 교차분석법 등을 통해 향상되어 왔으며(Paris et al., 2013), 34S/32S의 경우 기체시료를 이용한 동위원소 분석과 유사한 수준의 재현성이 보고되었다(Yu et al., 2017). 미량 황 동위원소인 33S의 경우 육불화황 기반의 분석과 비교해 다소 큰 오차를 보여, 광분해 반응과 같은 비질량종속 분별작용(mass-independent isotope fractionation, MIF) 연구에는 충분하지만 서로 다른 질량종속 분별작용 간의 미세한 차이로 인한 변화를 확인하기에는 부족한 수준이다(Johnston et al., 2008; Sim et al., 2011b; Ono et al., 2012). 또한 36S의 경우는 아르곤 (36Ar+)과의 간섭으로 분석이 어렵다. 이러한 한계에도 불구하고 적은 시료 요구량으로 인해 다검출기 유도결합 플라즈마 질량분석기(MC-ICP-MS)를 이용한 황 동위원소 분석법은 지속적으로 개선되고 있으며, 레이저삭박(laser ablation) 분석을 통한 표면 분석(Pribil et al., 2015) 등 향후 그 응용범위가 더 확대될 것으로 기대된다.
국내 황 동위원소 분석 또한 기체시료를 이용한 동위원소 분석을 중심으로 발전해 왔으며, 최근 이산화황을 기반으로 원소분석기와 결합한 황산염의 황 동위원소와 산소 동위원소 분석법이 보고되기도 하였다(Kim et al., 2017). MC-ICP-MS의 경우 전처리 과정을 통해 다양한 동위원소 분석에 응용이 가능한 장점으로 인해, 국내에도 한국기초과학지원연구원, 한국원자력연구원, 국립환경과학원 등 다수의 연구 기관에 도입되어 스트론튬, 아연, 구리, 납, 플루토늄 등 주로 미량원소의 동위원소에 연구에 이용되고 있지만(Yim et al., 2010; Choi, J.-W. et al., 2013; Park et al., 2016; Choi, H.-B. et al., 2018; Lee et al., 2018), 아직까지 MC-ICP-MS를 이용한 황 동위원소 연구는 전무한 실정이다. 최근 국내에서도 빙하 내 황산이온의 동위원소 분석, 황 동위원소를 이용한 미세먼지의 기원지 연구 등을 중심으로 미량시료의 황 동위원소 분석에 대한 요구가 높아지는 상황으로, 이번 연구에서는 서울대학교 공동기기원에 설치된 Nu-plasma 3 MC-ICP-MS를 이용해 미량 시료의 황 동위원소 분석법을 정립하고 국내 황 동위원소 연구를 활성화하고자 하였다.
2. 실험 방법
2.1 시료 처리
분석에 이용된 자체 표준물질(in-house standard material)은 고순도 Na2SO4 (≥99.99%, trace metals basis, Sigma-Aldrich)을 이용하였으며, 20 mM 저장용액(stock solution)을 0.8 M 초고순도 질산(ODLAB)을 이용해 20 μM로 희석시켜 사용하였다. 자체 표준물질의 황 동위원소 조성은 공인된 BaSO4 표준물질인 IAEA-SO-5와의 교차분석을 통해 측정하였으며, 자체 표준물질을 이용한 분석의 정확도는 또 다른 BaSO4 표준물질인 IAEA-SO-6와 NBS-127의 동위원소 조성을 자체 표준물질을 기준으로 측정하여 확인하였다. 물에 대한 용해도가 낮은 BaSO4를 표준용액을 제조하기 위해 과량의Na2CO3을 사용하였는데(Breit et al., 1985) 초순수 증류수 10 ml에 BaSO4 0.05 g과 Na2CO3 0.5 g을 넣고 80℃에서 5시간 가열한 뒤, 잔류 침전물을 원심분리와 초순수 증류수 50 ml를 5회 통과시켜 세척된 멤브레인 필터를 이용해 제거하였다. 바륨이 BaCO3 침전물로 제거된 Na2CO3-Na2SO4 용액에 초고순도 염산과 질소가스를 사용해 탄산이온을 이산화탄소로 제거하여 최종적으로 NaCl-Na2SO4 용액의 형태로 준비하였다. 이 과정에서 황산이온의 회수율은 90% 이상으로, 동위원소 조성이 알려진 시료 간의 교차분석을 통해 BaSO4 용해과정에서 동위원소 분별작용이 발생하지 않았음을 확인하였다. 이번 연구에서 이용한 해수 시료인 남극해 심층수는 0.2 μM 멤브레인 필터를 이용해 여과한 뒤 실험에 이용하였다. 시료 내 황산이온은 AG1-X8 음이온 교환수지(Bio-Rad)를 이용해 분리 회수하였으며(Paris et al., 2014), 각 단계별 분리법은 그림 1과 같다. 먼저 4 M 염산을 이용해 세척된 칼럼(Poly-Prep columns, 0.8 × 4 cm, Bio-Rad)에 음이온 교환수지 AG1-X8(100-200 mesh, Chloride form, BIO-RAD, Richmond, CA, USA) 0.6 ml를 채우고, 수지 부피의 20배에 해당하는 1.6 M 질산, 4 M 염산, 초순수 증류수를 차례로 이용해 불순물을 제거한 뒤, 수지 부피의 10배에 해당하는 0.2 M 초고순도 염산(ODLAB)을 통과시켜 칼럼을 준비하였다. 황산이온을 포함하는 시료를 음이온 교환수지가 채워진 칼럼에 주입한 후, 수지 부피 10배의 초순수 증류수를 통과시켜 양이온을 제거하였고, 황산이온은 수지 부피 6배의 0.5 M 초고순도 질산을 이용해 테플론 용기에 회수하였다. 시료에 포함된 이온 농도가 높은 경우 이용하는 음이온 교환수지의 부피는 이온교환용량을 고려해 조정하며, 이번 연구에 이용된 AG1-X8의 경우 1.2 meq/ml의 교환용량을 지닌다. 회수된 시료는 흑연 가열판을 이용해 105℃에서 12시간 이상 건조한 뒤 0.8 M 초고순도 질산을 이용해 자체 표준물질 농도에 맞춰 희석하여 준비하였으며, 표준물질과 시료 사이의 Na+ 농도차가 동위원소 분석에 미치는 영향을 줄이기 위해 고순도 NaOH용액(IC grade, Sigma-Aldrich)을 이용해 시료 내 Na+의 농도를 40 μM로 조정하였다(Paris et al., 2014; Yu et al., 2017). 황산이온의 회수와 희석에 이용되는 테플론 용기는 6 M 염산에서 80℃로 하룻밤 가열한 뒤 초순수 증류수로 5회 세척하고, 다시 8 M 질산을 이용해 동일한 과정을 반복하였다. 이에 더해, 0.08 M 초고순도 질산 50 μl이 담긴 테플론 용기를 160℃도의 흑연 가열판에서 12시간 가열한 뒤 초순수 증류수로 5회 세척 후 재사용하여 시료간 오염을 최대한 방지하였다. 자동시료공급기(ASX-112FR, Cetac)에 이용되는 0.5 ml 혹은 2 ml 폴리프로필렌 용기는 재사용하지 않았으며, 4 M 염산에 80℃로 하룻밤 혹은 상온에서 일주일 담가 둔 후, 초순수로 3회 세척, 건조하여 이용하였다.
2.2 황 동위원소 분석
시료 내 황 동위원소 32S, 33S, 34S의 비율은 서울대학교 공동기기원에 설치된 Nu Plasma 3 MC-ICP-MS를 이용하여 분석하였다. 산소와 수소에서 기인하는 분자이온의 동중이온 효과를 줄이기 위해 시료는 ESI PFA-100 분무기(nebulizer)와 Cetac Aridus II 탈용매화장치(desolvator)를 통해 MC-ICP-MS에 주입되었고, 3개의 패러데이 검출기(Faraday de-tector) L4, H1, H6를 이용하여 32S+, 33S+, 34S+를 질량 분해능(M/ΔM*; Weyer and Schwieters, 2003) 8000 수준에서 분석하였다. 이번 연구에서 질량 분해능은 피크의 평탄한 정상 높이 5%와 95%에 해당하는 질량 대 전하의 비(m/z)를 기준으로 측정하였다(Weyer and Schwieters, 2003). MC-ICP-MS를 이용한 동위원소 측정의 감도와 성능은 탈용매화장치의 상태, 공급되는 기체의 속도, 이온 빔의 경로 등 다양한 요소에 의해 결정되기 때문에, 분석 시 각 조건들에 대한 최적화과정을 수행하였으며 이번 연구의 일반적인 분석조건은 표 1과 같다. 분석 과정에서 발생할 수 있는 동위원소 분별작용의 영향을 제거하기 위해 5초간 50회 연속 측정으로 구성된 동위원소 분석이 시료와 내부 표준물질에 대해 교차 진행되었고, 시료와 표준물질 분석 이후 이들과 동일한 양의 Na+이 포함된 0.8 M 질산을 이용해 바탕값(blank) 분석이 이루어졌다. 바탕값-표준물질-바탕값-시료-바탕값-표준물질-바탕값의 순서로 진행된 분석에서, 시료와 내부 표준물질 분석 전후에 측정된 바탕값의 평균치를 분석 결과에서 제거하고, 시료 전후에 분석된 내부 표준물질의 동위원소 비를 이용해 시료의 황 동위원소 비를 보정하였다. 앞선 분석으로부터 발생한 기억효과(memory effect)를 제거하고자 매 분석 전 탈용매화 장치에 0.8 M 초고순도 질산을 60초간 주입하여 세척하고, 분석은 시료를 90초 동안 주입한 이후부터 수행하였다. 측정된 황 동위원소 조성은 국제 표준물질인 Vienna-Cañon Diablo Troilite (VCDT)를 기준으로 델타 표시법(δ-notation)을 적용해 보고하였다.
(1) |
다중 황 동위원소 연구에서 미량 동위원소의 분포는 일반적으로 δ33S 값이 질량종속 분별작용 상관관계에서 벗어난 정도를 이용해 Δ33S으로 표기하며, 이번 연구에서는 다음과 같은 식으로 Δ33S 값을 보고하였다(Ono et al., 2006; Ueno et al., 2015).
(2) |
3. 실험 결과 및 토의
MC-ICP-MS를 이용한 황 동위원소 분석은 산소 및 수소에 기인한 분자이온의 질량 간섭이 분석의 정확도를 저해하는 주요한 요소로 작용하며, 대표적인 동중 분자이온에 의한 간섭효과로는 32S+와 16O-16O+, 33S+와 32S-H+, 34S+와 16O-18O+를 들 수 있다(Paris et al., 2013; Pribil et al., 2015). 탈용매화장치는 이들 분자이온에 의한 질량 간섭효과를 경감시켜 주지만 완벽히 제거하지는 못하는데, 주요 동중 분자이온은 대응하는 S+에 비해 약간 무거운 질량을 지니기 때문에 피크의 가벼운 질량 말단에 간섭효과가 존재하지 않는 부분이 발생하게 된다(그림 2a). MC-ICP-MS를 이용한 황 동위원소 분석은 피크의 중앙부가 아닌 간섭효과가 없는 피크의 어깨 부분에서 이뤄지게 되며, 간섭효과의 분리를 위해 높은 질량 분해능이 요구된다. 이러한 측정 방식은 아르곤에서 기인한 분자이온 간섭효과가 발생하는 철 동위원소 분석 등의 사례와 유사하다(He et al., 2015). 탈용매화장치를 이용한 분석에서 산소와 수소로 인한 분자이온의 간섭효과는 각 황 동위원소에 해당하는 신호의 강도(voltage)에서는 뚜렷하지 않지만, 34S+와 33S+에 대응하는 검출기 신호와 32S+에 해당하는 신호의 비를 질량의 증가에 따라 확인할 때 명확히 드러난다. 가장 먼저 나타나는 간섭효과는 황 동위원소의 대부분을 차지하는 32S와 수소가 결합한 32S-H+ 이온과 33S+와의 간섭으로, 3 mDa (milli-Dalton) 정도의 구간에서 간섭효과 없이 분석이 가능함을 알 수 있다(그림 2b). 다중 황 동위원소 분석을 위해서는 이 영역에서 반드시 분석이 이뤄져야 하지만, 34S/32S 측정만 요구되는 경우 32S+ 분석에 교란을 주는 16O-16O+는 상대적으로 더 무거운 질량에서 나타나기 때문에 보다 넓은 질량 구간에서 분석을 수행할 수 있다(그림 2c).

Isobaric interferences on sulfur isotopes from molecular ions. Schematic illustration of the major isobaric interferences (a) and mass scan of the low mass shoulder showing interference-free flat shoulder plateaus for 34S/32S (b) and 33S/32S (c) measurements.
실험실 자체 표준물질의 다중 황 동위원소 조성은 공인된 표준물질인 IAEA-SO-5와의 30회 이상의 상호분석을 통해 측정하였다. IAEA-SO-5의 δ34S와 δ33S, Δ33S 값은 각각 0.68‰, 0.46‰, 0.097‰을 이용하였으며(Geng et al., 2018), 자체 표준물질의 δ34S와 δ33S, Δ33S 값은 -0.21±0.36‰ (2σ, n = 32), -0.10±0.44‰ (2σ, n = 32), 0.01±0.44‰ (2σ, n = 32)로 측정되었다(그림 3). 자체 표준물질을 이용해 측정한 BaSO4 인증표준물질의 IAEA-SO-6와 NBS-127의 δ34S 값은 -33.80±0.44‰ (2σ, n = 6)과 21.03±0.43‰ (2σ, n = 8)로 기존에 보고된 값과 오차 범위 내에서 일치하는 결과를 보여주며(표 2), 황 동위원소 분석이 넓은 범위의 δ34S 값을 지니는 시료에 대해 성공적으로 수행되었음을 의미한다. 이번 연구 δ34S 분석의 재현성은 탈용매화장치와 MC-ICP-MS를 이용한 선행 연구에서 보고된 0.1 ~ 0.5‰ (2σ)와 유사한 수준으로 서울대학교 공동기기원 MC-ICP-MS를 이용한 δ34S 동위원소 분석의 신뢰도를 확인시켜준다(Paris et al., 2013; Hanousek et al., 2016; Yu et al., 2017). 측정된 IAEA-SO-6와 NBS-127의 Δ33S값 또한 0.03±0.52‰ (2σ, n = 6)과 0.10±0.34‰ (2σ, n = 8)로 육불화황을 이용한 다중 동위원소 분석을 통해 보고된 값과 오차 범위 내에서 일치하지만, MC-ICP-MS를 이용한 이번 분석의 불확실성이 상대적으로 큰 것을 확인할 수 있다(표 2). 이는 앞서 논의한 것처럼 MC-ICP-MS를 이용한 황 동위원소 분석이 다양한 동중 이온과 S+ 사이의 간섭효과에 영향을 받는 반면 32SF5+, 33SF5+, 34SF5+ 이온과 간섭을 일으킬 수 있는 동중 이온은 드물기 때문이며, 육불화황 기체를 이용한 분석의 경우에도 극미량으로 존재하는 36S의 경우 36SF5+와 C3F5+ 등 동중 이온의 간섭효과에 대한 주의가 필요한 것으로 알려져 있다(Ono et al., 2006; Ueno et al., 2015). 본 연구에서 확인된 Δ33S의 분석 재현성은 0.3 ~ 0.5‰ (2σ) 수준으로, 탈용매화장치와 MC-ICP-MS를 이용한 선행 연구(Paris et al., 2013)에서 보고된 0.1 ~ 0.3‰ 보다는 다소 큰 범위를 보이지만, 33S 지시자를 이용한 실험이나, 황화합물의 광분해 반응, 시생누대의 퇴적암에 기록된 수 ‰에 이르는 비질량종속 분별작용 연구에 유용한 분석능력이 확보되었음을 확인시켜준다(Johnston, 2011; Whitehill et al., 2013; Sim et al., 2017). 향후 Δ33S 분석의 재현성은 질량분해능(M/ΔM*)이 ~10000인 고분해능 조건(high resolution mode)에서의 분석(Paris et al., 2013), Ni2+ 이온으로 인한 교란을 방지하기 위한 알루미늄 콘의 사용 등을 통해 향상시키고자 한다. 다만, 미량시료에서 질량종속 분별작용에 따른 다중 황 동위원소 거동 연구는 미지의 영역으로 남아있는데, 이는 33S 지시자나 비질량종속 분별작용을 제외한 다중 황 동위원소 연구에 요구되는 Δ33S 분석 오차는 0.01‰ 수준으로(Johnston et al., 2008; Sim et al., 2011b; Ono et al., 2012) 이번 연구를 포함해 현재까지 보고된 MC-ICP-MS 분석 오차의 1/10 혹은 그 이하에 불과해 큰 차이를 보이기 때문이다.

Measurement of δ34S, δ33S, and Δ33S for in-house Na2SO4 standard. Sulfur isotope ratios were determined against the IAEA-SO-5 standard.
MC-ICP-MS를 이용한 분석은 매우 적은 양의 시료에서 정밀한 황 동위원소 비 측정을 가능케하지만, 나노 몰 수준의 시료는 주변 환경으로부터의 오염에 취약하며 황산염과 같이 실험실 혹은 지표환경에 흔히 존재하는 물질을 분석대상으로 하는 경우 그 어려움이 두드러진다. 시료에 포함된 황산이온의 양이 분석결과의 미치는 영향을 시험하기 위해, 해수 시료를 희석하여 각각 1.4 μmol, 140 nmol, 14 nmol, 7 nmol의 황산이온을 포함하도록 준비한 뒤, 칼럼분리 작업을 진행하고 동위원소 분석을 수행하였다. 측정된 δ34S 값은 1.4 μmol에서 14 nmol까지는 오차 범위 내에서 일치하고 선행연구에서 보고된 황 동위원소 조성에 부합하는 결과를 보인 반면, 7 nmol 황산이온을 포함한 시료는 다른 해수 분석 값보다 1‰ 이상 가벼운 황 동위원소 조성을 보였다(그림 4). 제한된 시료의 양으로 인해 최종적으로 분석되는 이용되는 농도가 표준물질의 50%에 미치지 못하는 경우 그 차이가 더욱 두드러진다. 이는 시료 내 황산이온의 양이 10 nmol에 미치지 못하는 경우 신뢰도 있는 분석결과를 얻기 위해 각별한 주의가 필요함을 의미하며, 33S 지시자(Δ33S ≈ 200‰)를 이용한 미생물 대사작용 연구에서도 10 nmol 미만의 대사물질 대한 MC-ICP-MS 분석결과에서 Δ33S 값이 희석되는 경향이 보고된 사례가 있다(Sim et al., 2017). δ34S와 반대로, 이번 연구에서 측정된 해수 시료의 Δ33S 값은 모두 유사한 결과를 보여주는데(그림 3), 가장 적은 양의 황산이온을 포함한 시료에서도 Δ33S의 차이가 두드러지지 않은 이유는 MC-ICP-MS를 이용해 측정된 Δ33S 값이 δ34S와 비교해 상대적으로 큰 오차 범위를 지니기 때문이다. 또한, 시료 처리 과정에서 사용된 산을 비롯한 시약 및 실험실 환경에서 기원한 황산이온 불순물이 해수와 비교해 δ34S 값에서 큰 차이를 보이더라도 질량종속 분별작용 관계을 벗어나지 않는 한 해수와 마찬가지로 0‰에 매우 가까운 Δ33S 값을 지니기 때문에, MC-ICP-MS 분석 결과에서 오차 범위를 넘어서는 Δ33S 값 변동을 기대하기는 어렵다. 다만 32S+를 측정하는 검출기 기준으로 신호가 1.2 V에 미치지 못하는 경우에는 Δ33S 값이 오차범위를 벗어나 정상적인 33S의 검출이 수행될 수 없음을 확인하였다(그림 5).

Precision and accuracy of MC-ICP-MS sulfur isotope analysis depending on sample size. Error bars represent the analytical uncertainty estimated by repeated measurements of the standard materials. Reported δ34S and Δ33S values of seawater sulfate typically range from 20.4 to 21.3 and 0.05 to 0.08‰, respectively (Kampshulte et al., 2001; Böttcher et al., 2007; Craddock et al., 2008; Peters et al., 2010; Das et al., 2012, Ono et al., 2012; Paris et al., 2013; Tostevin et al., 2014).
4. 결 론
이번 연구에서는 서울대학교 공동기기원에 설치된 MC-ICP-MS를 이용해 다중 황 동위원소 분석을 수행하였다. 시료에 포함된 황산이온은 음이온 교환수지를 이용해 분리하였으며, 탈용매화장치를 통해 산소와 수소에서 기인한 동중 분자이온의 질량간섭을 최소화하고, S+ 피크의 중앙이 아닌 간섭에서 자유로운 가벼운 질량 말단부에서 동위원소 비를 측정하였다. 표준시료를 이용해 확인한 δ34S와 Δ33S 분석 오차(2σ)는 각각 0.44‰과 0.52‰ 이하였으며, 최소 14 nmol의 황산이온을 포함하고 있는 시료에서도 정밀한 분석이 가능함을 보였다. BaSO4, Na2SO4, 해수 시료 등 황산염을 중심으로 동위원소 분석을 수행하였지만, 황화물이나 유기물에 포함된 황, 혹은 아황산 등의 황 화합물도 정량적인 산화과정을 거쳐 황산이온의 형태로 동위원소 분석에 이용될 수 있어(Raven et al., 2016; Sim et al., 2017), 다양한 환경시료 및 지질시료에 대한 연구에 본 분석법의 응용 가능성 또한 큰 편으로 판단된다. 이번 연구를 통해 미량시료의 황 동위원소 분석이 국내에서 가능해짐에 따라 빙하에 포함된 황화합물(Burke et al., 2019), 미세먼지(Chung et al., 2019), 인체나 미생물 세포 내 대사물질(Albalat et al., 2016; Sim et al., 2017) 등 전통적인 황 동위원소 분석을 위한 시료 확보에 어려움이 있었던 분야의 국내 연구 활성화에 이바지할 수 있을 것으로 기대된다.
Acknowledgments
본 연구는 극지연구소의 국내 학·연 극지연구진흥프로그램(PE20900) 사업과 한국연구재단의 신진연구지원사업(2019R1C1C1005550)의 지원을 받아 수행되었습니다. 심사과정에서 유익한 조언을 주신 두 분의 심사위원과 편집위원께 감사드립니다.
References
-
Albalat, E., Telouk, P., Balter, V., Fujii, T., Bondanese, V.P., Plissonnier, M.L., Vlaeminck-Guillem, V., Baccheta, J., Thiam, N., Miossec, P. and Zoulim, F., 2016, Sulfur isotope analysis by MC-ICP-MS and application to small medical samples. Journal of Analytical Atomic Spectrometry, 31, 1002-1011.
[https://doi.org/10.1039/C5JA00489F]
-
Böttcher, M.E., Brumsack, H.J. and Dürselen, C.D., 2007, The isotopic composition of modern seawater sulfate: I. Coastal waters with special regard to the North Sea. Journal of Marine Systems, 67, 73-82.
[https://doi.org/10.1016/j.jmarsys.2006.09.006]
-
Breit, G.N., Simmons, E.C. and Goldhaber, M.B., 1985, Dissolution of barite for the analysis of strontium isotopes and other chemical and isotopic variations using aqueous sodium carbonate. Chemical Geology, 52, 333-336.
[https://doi.org/10.1016/0168-9622(85)90043-0]
-
Burke, A., Moore, K.A., Sigl, M., Nita, D.C., McConnell, J.R. and Adkins, J.F., 2019, Stratospheric eruptions from tropical and extra-tropical volcanoes constrained using high-resolution sulfur isotopes in ice cores. Earth and planetary science letters, 521, 113-119.
[https://doi.org/10.1016/j.epsl.2019.06.006]
-
Choi, H.-B., Ryu, J.-S., Park, S. and Lee, J., 2018, Lead isotope measurement using a multi-collector inductively coupled plasma mass spectrometry (MC-ICP-MS). Journal of the Geological Society of Korea, 54, 311-318 (in Korean with English abstract).
[https://doi.org/10.14770/jgsk.2018.54.3.311]
- Choi, J.-W., Yoo, E., Kim, J., Lee, K., Lee, W.-S. and Han, J., 2013, Study on performance evaluation of MC-ICP/MS for measuring stable isotope ratios. Journal of the Korean Society of Environmental Analysis, 16, 49-56 (in Korean with English abstract).
-
Christensen, L.E., Brunner, B., Truong, K.N., Mielke, R.E., Webster, C.R. and Coleman, M., 2007, Measurement of sulfur isotope compositions by tunable laser spectroscopy of SO2. Analytical Chemistry, 79, 9261-9268.
[https://doi.org/10.1021/ac071040p]
-
Chung, C.H., You, C.F., Hsu, S.C. and Liang, M.C., 2019, Sulfur isotope analysis for representative regional background atmospheric aerosols collected at Mt. Lulin, Taiwan. Scientific reports, 9, 1-9.
[https://doi.org/10.1038/s41598-019-56048-z]
-
Craddock, P.R., Rouxel, O.J., Ball, L.A. and Bach, W., 2008, Sulfur isotope measurement of sulfate and sulfide by high-resolution MC-ICP-MS. Chemical Geology, 253, 102-113.
[https://doi.org/10.1016/j.chemgeo.2008.04.017]
-
Das, A., Chung, C.H., You, C.F. and Shen, M.L., 2012, Application of an improved ion exchange technique for the measurement of δ34S values from microgram quantities of sulfur by MC-ICPMS. Journal of Analytical Atomic Spectrometry, 27, 2088-2093.
[https://doi.org/10.1039/c2ja30189j]
-
Farquhar, J., Bao, H. and Thiemens, M., 2000, Atmospheric influence of Earth's earliest sulfur cycle. Science, 289, 756-758.
[https://doi.org/10.1126/science.289.5480.756]
-
Fike, D.A., Bradley, A.S. and Rose, C.V., 2015, Rethinking the ancient sulfur cycle. Annual Review of Earth and Planetary Sciences, 43, 593-622.
[https://doi.org/10.1146/annurev-earth-060313-054802]
-
Geng, L., Savarino, J., Savarino, C.A., Caillon, N., Cartigny, P., Hattori, S., Ishino, S. and Yoshida, N., 2018, A simple and reliable method reducing sulfate to sulfide for multiple sulfur isotope analysis. Rapid Communications in Mass Spectrometry, 32, 333-341.
[https://doi.org/10.1002/rcm.8048]
-
Halas, S. and Szaran, J., 2001, Improved thermal decomposition of sulfates to SO2 and mass spectrometric determination of δ34S of IAEA SO-5, IAEA SO-6 and NBS-127 sulfate standards. Rapid Communications in Mass Spectrometry, 15, 1618-1620.
[https://doi.org/10.1002/rcm.416]
-
Hanousek, O., Berger, T.W. and Prohaska, T., 2016, MC ICP-MS δ34S VCDT measurement of dissolved sulfate in environmental aqueous samples after matrix separation by means of an anion exchange membrane. Analytical and bioanalytical chemistry, 408, 399-407.
[https://doi.org/10.1007/s00216-015-9053-z]
-
He, Y., Ke, S., Teng, F.Z., Wang, T., Wu, H., Lu, Y. and Li, S., 2015, High-precision iron isotope analysis of geological reference materials by high‐resolution MC-ICP-MS. Geostandards and Geoanalytical Research, 39, 341-356.
[https://doi.org/10.1111/j.1751-908X.2014.00304.x]
-
Hulston, J.R. and Thode, H.G., 1965, Variations in the S33, S34, and S36 contents of meteorites and their relation to chemical and nuclear effects. Journal of Geophysical Research, 70, 3475-3484.
[https://doi.org/10.1029/JZ070i014p03475]
-
Johnston, D.T., 2011, Multiple sulfur isotopes and the evolution of Earth's surface sulfur cycle. Earth-Science Reviews, 106, 161-183.
[https://doi.org/10.1016/j.earscirev.2011.02.003]
-
Johnston, D.T., Farquhar, J., Habicht, K.S. and Canfield, D.E., 2008, Sulphur isotopes and the search for life: strategies for identifying sulphur metabolisms in the rock record and beyond. Geobiology, 6, 425-435.
[https://doi.org/10.1111/j.1472-4669.2008.00171.x]
-
Johnston, D.T. and Fischer, W.W., 2012, Stable isotope geobiology. In: Knoll, A.H., Canfield, D.E. and Konhauser, K.O. (eds.), Fundamentals of geobiology. Wiley-Blackwell, Chichester, 250-268.
[https://doi.org/10.1002/9781118280874.ch14]
-
Kampschulte, A., Bruckschen, P. and Strauss, H., 2001, The sulphur isotopic composition of trace sulphates in Carboniferous brachiopods: implications for coeval seawater, correlation with other geochemical cycles and isotope stratigraphy. Chemical Geology, 175, 149-173.
[https://doi.org/10.1016/S0009-2541(00)00367-3]
-
Kim, J., Lee, J., Choi, S., Bong, Y.-S., Choi, U., Yang, I.-J., Choi, H.-B. and Shin, W.-J., 2017, An on-line analytical method for sulfur and oxygen isotopes of sulfate using EA-IRMS and HT-EA-IRMS. Journal of the Geological Society of Korea, 53, 477-485 (in Korean with English abstract).
[https://doi.org/10.14770/jgsk.2017.53.3.477]
-
LaFlamme, C., Martin, L., Jeon, H., Reddy, S.M., Selvaraja, V., Caruso, S., Bui, T.H., Roberts, M.P., Voute, F., Hagemann, S. and Wacey, D., 2016, In situ multiple sulfur isotope analysis by SIMS of pyrite, chalcopyrite, pyrrhotite, and pentlandite to refine magmatic ore genetic models. Chemical Geology, 444, 1-15.
[https://doi.org/10.1016/j.chemgeo.2016.09.032]
- Lee, S.-W., Park, J., Park., H.-W., Hwang, J.Y., Kim, K., Chung, H.-M. and Choi, J.-W., 2018, Improving strontium isotope ratio analysis using MC-ICP-MS. Journal of the Korean Society for Environmental Analysis, 21, 237-242 (in Korean with English abstract).
-
Mann, J.L. and Kelly, W.R., 2005, Measurement of sulfur isotope composition (δ34S) by multiple‐collector thermal ionization mass spectrometry using a 33S-36S double spike. Rapid Communications in Mass Spectrometry, 19, 3429-3441.
[https://doi.org/10.1002/rcm.2213]
-
Ono, S., Keller, N.S., Rouxel, O. and Alt, J.C., 2012, Sulfur-33 constraints on the origin of secondary pyrite in altered oceanic basement. Geochimica et Cosmochimica Acta, 87, 323-340.
[https://doi.org/10.1016/j.gca.2012.04.016]
-
Ono, S., Wing, B., Rumble, D. and Farquhar, J., 2006, High precision analysis of all four stable isotopes of sulfur (32S, 33S, 34S and 36S) at nanomole levels using a laser fluorination isotope-ratio-monitoring gas chromatography-mass spectrometry. Chemical Geology, 225, 30-39.
[https://doi.org/10.1016/j.chemgeo.2005.08.005]
-
Paris, G., Adkins, J.F., Sessions, A.L., Webb, S.M. and Fischer, W.W., 2014, Neoarchean carbonate-associated sulfate records positive Δ33S anomalies. Science, 346, 739-741.
[https://doi.org/10.1126/science.1258211]
-
Paris, G., Sessions, A.L., Subhas, A.V. and Adkins, J.F., 2013, MC-ICP-MS measurement of δ34S and Δ 33S in small amounts of dissolved sulfate. Chemical Geology, 345, 50-61.
[https://doi.org/10.1016/j.chemgeo.2013.02.022]
-
Park, S., Ryu, J.-S., Shin, H.S., Kil, Y. and Jo, Y., 2016, Copper isotope measurements using a Neptune MC-ICP-MS. Journal of the Mineralogical Society of Korea, 29, 221-227 (in Korean with English abstract).
[https://doi.org/10.9727/jmsk.2016.29.4.221]
-
Peters, M., Strauss, H., Farquhar, J., Ockert, C., Eickmann, B. and Jost, C.L., 2010, Sulfur cycling at the Mid-Atlantic Ridge: A multiple sulfur isotope approach. Chemical Geology, 269, 180-196.
[https://doi.org/10.1016/j.chemgeo.2009.09.016]
-
Pribil, M.J., Ridley, W.I. and Emsbo, P., 2015, Sulfate and sulfide sulfur isotopes (δ34S and δ33S) measured by solution and laser ablation MC-ICP-MS: An enhanced approach using external correction. Chemical Geology, 412, 99-106.
[https://doi.org/10.1016/j.chemgeo.2015.07.014]
-
Raven, M.R., Sessions, A.L., Fischer, W.W. and Adkins, J.F., 2016, Sedimentary pyrite δ34S differs from porewater sulfide in Santa Barbara Basin: Proposed role of organic sulfur. Geochimica et Cosmochimica Acta, 186, 120-134.
[https://doi.org/10.1016/j.gca.2016.04.037]
-
Rees, C.E., Jenkins, W.J. and Monster, J., 1978, The sulphur isotopic composition of ocean water sulphate. Geochimica et Cosmochimica Acta, 42, 377-381.
[https://doi.org/10.1016/0016-7037(78)90268-5]
-
Sim, M.S., Bosak, T. and Ono, S., 2011a, Large sulfur isotope fractionation does not require disproportionation. Science, 333, 74-77.
[https://doi.org/10.1126/science.1205103]
-
Sim, M.S., Ono, S., Donovan, K., Templer, S.P. and Bosak, T., 2011b, Effect of electron donors on the fractionation of sulfur isotopes by a marine Desulfovibrio sp. Geochimica et Cosmochimica Acta, 75, 4244-4259.
[https://doi.org/10.1016/j.gca.2011.05.021]
-
Sim, M.S., Paris, G., Adkins, J.F., Orphan, V.J. and Sessions, A.L., 2017, Quantification and isotopic analysis of intracellular sulfur metabolites in the dissimilatory sulfate reduction pathway. Geochimica et Cosmochimica Acta, 206, 57-72.
[https://doi.org/10.1016/j.gca.2017.02.024]
-
Tostevin, R., Turchyn, A.V., Farquhar, J., Johnston, D.T., Eldridge, D.L., Bishop, J.K. and McIlvin, M., 2014, Multiple sulfur isotope constraints on the modern sulfur cycle. Earth and planetary science letters, 396, 14-21.
[https://doi.org/10.1016/j.epsl.2014.03.057]
-
Ueno, Y., Aoyama, S., Endo, Y., Matsu'ura, F. and Foriel, J., 2015, Rapid quadruple sulfur isotope analysis at the sub-micromole level by a flash heating with CoF3. Chemical Geology, 419, 29-35.
[https://doi.org/10.1016/j.chemgeo.2015.10.032]
-
Whitehill, A.R., Xie, C., Hu, X., Xie, D., Guo, H. and Ono, S., 2013, Vibronic origin of sulfur mass-independent isotope effect in photoexcitation of SO2 and the implications to the early earth’s atmosphere. Proceedings of the National Academy of Sciences, 110, 17697-17702.
[https://doi.org/10.1073/pnas.1306979110]
-
Weyer, S. and Schwieters, J.B., 2003, High precision Fe isotope measurements with high mass resolution MC-ICPMS. International Journal of Mass Spectrometry, 226, 355-368.
[https://doi.org/10.1016/S1387-3806(03)00078-2]
- Yim, S.A., Han, E., Chae, J.S. and Yun, J.-Y., 2010, The improved method for precise determination of Pu isotope ratio using MC-ICP-MS. Journal of Radiation Protection and Research, 35, 117-123 (in Korean with English abstract).
-
Yu, T.L., Wang, B.S., Shen, C.C., Wang, P.L., Yang, T.F., Burr, G.S. and Chen, Y.G., 2017, Improved analytical techniques of sulfur isotopic composition in nanomole quantities by MC-ICP-MS. Analytica chimica acta, 988, 34-40.
[https://doi.org/10.1016/j.aca.2017.08.012]