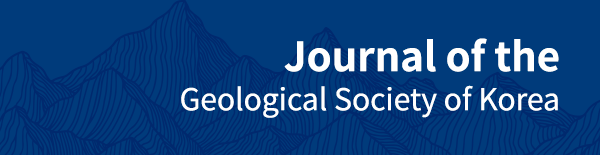
울산단층 중부 원원사단층의 운동특성과 시기



초록
활성단층의 단층변수를 도출하는 것은 지진재해 대비에 중요한 자료로 활용될 수 있다. 본 연구에서는 중부 울산단층대의 동편에서 최근 확인된 원원사단층 제2지점을 상세히 기재하고, 활동시기 등의 단층변수를 보고하고자 한다. 이 단층노두는 흑운모 화강암을 염기성 암맥이 관입하고 있으며, 이 관입경계를 따라 역단층의 운동감각으로 전단되어, 기반암 상위에 퇴적된 제4기 하성퇴적층을 절단하고 있다. 변위량은 기반암과 제4기층의 부정합면을 기준으로 약 42 cm의 수직 낙차가 인지되나, 단층면과 단층조선 등을 활용하여 계산된 실변위량은 약 46 cm이다. 절단된 퇴적층에서 획득한 광여기루미네선스(Optically Stimulated Luminescence; OSL) 연대측정결과 상반의 퇴적층은 약 6천 년, 하반의 퇴적층에서는 약 3천 년의 매몰연대가 도출되었고, 우주선유발동위원소인 10Be을 활용한 지형면의 노출연대는 약 9천년으로 측정되었다. 연대측정법에 따라 결과 값의 차이는 있지만, 여러 분석결과를 종합할 때 이 단층이 홀로세에 활동한 것은 분명하다. 10Be의 유전량을 고려한다면, 노출연대는 최대연대를 지시하며 이보다 젊은 광여기루미네선스 연대가 실제 단층활동연대에 가까울 것이다. 따라서 약 3,600년 전인 홀로세에 지표파열을 수반한 단층활동이 있었을 것으로 추정된다. 이 결과는 지금까지 울산단층대에서 보고된 제4기 단층활동 중 가장 젊은 활동연대를 지시한다.
Abstract
Deriving paleoseismological fault parameters of active faults is essential for earthquake disaster provision. The purpose of this study is to document the fault characteristics of Wonwonsa fault, which was recently reported in the 2nd fault outcrop on the eastern side of the Ulsan fault, and to provide fault parameters, such as the timing of activity. The fault slipped along the boundary between biotite granite and andesitic dike, and cuts Quaternary fluvial deposits, which deposited above basement rock. Vertical separation of about 42 cm is recognized based on the unconformity surface between the bedrock and the Quaternary fluvial deposit, but net-slip using fault geometry is calculated to be 46 cm. The burial ages of the fluvial deposits are ca. 6 ka (hanging wall part) and ca. 3 ka (footwall part) based on the optically stimulated luminescence (OSL) dating. The boulder's exposure age, which is located on the terrace surface using 10Be, was ca. 9,000 years. Although there is a difference between two dating methods, it is interpreted that this fault slipped at least once during the Holocene. Because we cannot rule out the possibility of the inheritance although it is minimal considering the steep mountain stream, the 10Be exposure had better be taken as a maximum. So, the OSL age may be near to the real age. This fault seems to have been activated after 3,600 years ago, implying that an earthquake with the surface rupture occurred during the Holocene. This result indicates the youngest active faulting age among the Quaternary faults reported along the Ulsan fault.
Keywords:
Ulsan fault, Quaternary fault, OSL, 10Be, faulting age키워드:
울산단층, 제4기단층, 광여기루미네선스, 10Be, 단층활동연대1. 서 론
한반도는 판구조적으로 볼 때 유라시아판의 가장자리에 해당하여(그림 1a) 판의 경계지역들에 비해 상대적으로 지진의 발생빈도가 낮고 규모도 작다(e.g., Scholz et al., 1986). 하지만 다수의 고지진학(paleoseismology) 연구결과들(e.g., Kyung, 1997; Kyung et al., 1999a, 1999b; Yang, 2006; Kee et al., 2007; Choi, S.-J. et al., 2012, 2014; Lee et al., 2015; Choi, J.-H. et al., 2019; Song et al., 2020)과 고고지진학(archeoseismology) 및 역사지진자료(e.g., Jin et al., 2011; KMA, 2014; Jin and Kim, 2020)를 종합하면, 한반도에서 지표파열을 수반하거나 그에 준하는 중규모 이상의 지진이 간헐적으로 발생하였다. 특히 최근 발생한 두 차례의 중규모 지진인 경주지진(Mw 5.5; Kim et al., 2017)과 포항지진(Mw 5.4; Kim et al., 2018; Choi et al., 2019)은 한반도에서의 활성단층과 지진발생 메카니즘에 대한 관심을 증가시켰다(그림 1b; Kim, 2020; Kim et al., 2020).

(a) Tectonic map of East Asia and adjacent areas (EP: Eurasian Plate, PP: Pacific Plate, NAP: North American Plate, and PSP: Philippine Sea Plate). (b) Geologic map of the Gyeongsang and Yeonil basins. Quaternary fault sites (red circles) and focal mechanism solutions of two recent moderate earthquakes (Mw 5.5, 12 Sep. 2016 in Gyeongju / Mw 5.4, 15 Nov. 2017 in Pohang) are indicated. (c) Geomorphology around the study area, located in the eastern side of the Ulsan Fault (Mt. BI: Baegil Mountain, Mt. BS: Bongseo Mountain, Mt. GM: Gwanmun Mountain, and Res. MH: Mohwa Reservoir). (d) Topographic map and location of the Wonwonsa fault sites.
지진은 지각에 축적된 지구조적 응력이 단층의 전단운동을 통해 해소될 때 발생한다(e.g., Scholz, 2002). 하지만 큰 규모의 지진은 대부분 새로운 단층을 형성하기보다는 기존에 발달한 단층을 따라 다시 재활하는 경우가 많으며(e.g., McKenzie, 1972; Sykes, 1978; Sibson, 1985), 이러한 이유로 현생 응력조건에서 움직였던 단층은 다시 동일한 지구조적 응력조건에서 재활할 가능성이 높다. 한반도는 지역에 따라 약간의 차이는 있지만, 제4기 단층의 기하와 운동학적 특성(Kim et al., 2016), 수압파쇄(Haimson et al., 2003; Bae, 2005; Choi et al., 2008; Chang et al., 2010), 지진파 분석(Kim, 1980; Kim et al., 2006; Cho et al., 2006; Park et al., 2007; Hoe and Kyung, 2008; Jun and Jeon, 2010; Back et al., 2011; Jung and Kyung, 2013) 등을 종합하면 현재 한반도의 현생응력은 서북서-동남동 내지 동북동-서남서 방향으로 최대 주응력이 작용하는 것으로 해석되고 있다. 또한 다수의 고응력 및 구조발달사 연구들은 후기 마이오세부터 현재까지 거의 비슷한 조건이 유지되었을 가능성이 높은 것으로 판단하고 있다(Cheon et al., 2017). 활성단층은 ‘최근 지질시대에 활동했으며 가까운 미래에 다시 활동하여 지진을 유발할 수 있는 단층’으로 정의되며(Slemmons and McKinney, 1977), 국가나 사용목적에 따라 그 정의 및 시기의 범위가 상이하다(e.g., U.S. AEC to 10 CFR Part 100 Appendix A, 1971; RGAFJ, 2011). 한반도에서는 이러한 응력조건을 고려하여 제4기 동안 활동한 단층을 다시 재활할 가능성이 있는 단층으로 정의하고 있다(Kim et al., 2011, 2020).
제4기단층의 주향과 경사 등의 기하학적 정보, 운동감각과 변위량 등의 운동학적 정보, 그리고 시공간정보를 종합적으로 고려한다면, 미래 지진규모 및 발생시기를 예측하는데 도움이 될 것이다(McCalpin, 1996). 따라서 활성단층의 이러한 정보를 도출하는 것은 무엇보다 중요한 의미를 가진다. 이번 연구는 Gwon et al. (2018, 2019)에 의해 처음 보고된 경주시 외동읍 모화리 원원사 사찰 동편 계곡의 제4기층을 절단하는 단층(원원사단층 제2지점)을 면밀히 관찰하여, 이 단층의 기하학적 특징과 운동특성, 연대측정결과를 바탕으로 지진변수를 도출하고, 주변 단층들과의 관계를 해석하는데 그 목적이 있다.
2. 지형 및 지질개관
2.1 지형개관
연구지역은 경상북도 경주시 외동읍 모화리에 위치하고 있다(그림 1). 연구지역의 북쪽에는 백일산, 서쪽에는 봉서산이 있으며, 동쪽에는 관문산과 같은 해발고도 600 m 이상의 산들이 둘러싸고 있다(그림 1c). 북쪽과 동쪽의 산지는 전반적으로 고도가 높고 험준한 데 반해, 서쪽의 봉서산과 남쪽 산지들은 상대적으로 고도가 낮다. 이 지역의 수계는 산지의 곡부를 따라 북북동-남남서 방향의 직선형 하천이 약 2 km 길이로 발달하고 있으며, 이 하천은 모화저수지로 흐른 후 동천으로 유입된다(그림 1c). 이 하천의 양안에는 소규모 하안단구가 발달하고 있다(그림 1d).
2.2 지질개요
연구지역은 경상분지의 남동부에 위치하며, 백악기에서 고신기(paleogene) 사이에 관입한 불국사화강암류로 구성된다(그림 1b). 불국사화강암은 지역에 따라 다양한 광물조성을 보이지만, 흑운모화강암의 비중이 높다(Park et al., 2014). 이들의 상위에 분출 혹은 부정합으로 발달하는 암석들은 울산단층 동편에 주로 분포하며, 안산암 내지 현무암으로 구성되는 화산암류와 이들 기원의 화산쇄설물 또는 쇄설성 퇴적암류로 구성된다(Kim et al., 2015). 또한 일부 계곡부와 해안을 따라서는 선상지나 단구의 형태로 제4기 퇴적층이 발달하고 있다.
울산단층은 한반도에서 대규모 지진을 발생시킬 가능성이 가장 높은 단층 중 하나로 이들을 따라서는 다수의 제4기 지층을 절단하는 단층들이 보고되고 있다(그림 1b). 울산단층을 따라서 기 보고된 제4기단층은 대부분 동편의 지괴가 서편으로 충상하는 양상을 보인다(e.g., Choi et al., 2012).
기존 보고된 원원사단층 제1지점(구: 원원사단층)은 원원사 사찰 동편 계곡에 약 20 m 가량 노출되어있다(그림 2). 이 노두에서는 기반암인 흑운모화강암과 이를 관입한 중성질암맥이 관찰되며, 기반암 상위에는 제4기 자갈층이 부정합으로 덮고 있다. 제4기 자갈퇴적층의 특징은 원마도가 각형 내지 아각형이며, 잔자갈에서 거력의 입도를 가진다. 분급이 불량하며, 부분적으로 조립질의 사질퇴적물이 협재한다. 이 자갈퇴적층의 연대는 단층의 하반에서 획득한 광여기루미네선스 연대결과가 약 27,000년에서 41,000년 내외로 도출되었고, 상반에서는 약 46,000년에서 68,000년 내외로 도출된 바 있다(Gwon et al., 2019). 단층의 자세는 Lee and Schwarcz (2001)는 N51°E/14°NW, Yang (2006)은 NS/14°W, Kee et al. (2009)는 N5°E/12°SE, Choi et al. (2012)은 N16°W/52°SW로 측정하는 등 측정자들마다 단층의 주향과 경사가 차이가 있지만 대부분 서쪽으로 저각의 경사를 가지는 것으로 의견이 모아진다. Lee and Schwarcz (2001)는 단층암시료에 대한 전자회전공명(Electro Spin Resonance; ESR)법을 이용한 연대측정을 통해 제4기 동안 최소 4번 이상의 재활이 있었음을 주장하였다. 이를 종합하면 원원사단층 제1지점은 제4기동안 수 회 움직였으며, 가장 최근의 단층활동시기는 약 27,000년 전 이후일 것으로 판단된다.

Outcrop photographs of the first site of Wonwonsa fault. The fault (white arrows) is developed between basement rock(granite in hanging wall) and Quaternary deposit(in footwall)(3 m folding rule for scale). Note the sampling locations and OSL age dating results (Gwon et al., 2019).
원원사단층 제2지점은 기반암인 흑운모화강암을 안산암질의 암맥이 관입하고 있으며, 잔자갈에서 거력에 이르는 하안단구 퇴적층이 이들의 상위를 피복하고 있다(그림 3). 제4기층은 하성퇴적 기원으로 단구면과 하천의 비고차는 약 1.2 m 이며, 단구면에는 직경 30~100 cm 내외의 거력이 다수 분포한다. 제4기 퇴적층은 모래에서 굵은 모래의 입도를 가지는 하성퇴적층(Qa)과 자갈에서 왕자갈의 입도를 가지는 하성퇴적층(Qb)으로 대분 할 수 있다. 퇴적층 Qa의 구성입자는 아원형을 보이며, 분급이 좋다. 사층리가 거칠게 발달하며, 자갈들의 장축이 북쪽으로 침강하는 인편구조(imbrication)가 발달한다. 따라서 고수류의 방향은 현생하천의 유수방향과 같다. 퇴적층 Qb는 각형 내지 아각형의 원마도를 가지며, 입자지지 조직을 보인다. 분급은 보통 내지 불량한데, 자갈에서 왕자갈 입도의 자갈이 주를 이루나 일부 불규칙적으로 모래층이 협재되어 있다. 이 퇴적층의 고결정도는 매우 느슨하다(그림 3).

Outcrop photographs of the second site of Wonwonsa fault. (a) The basement rocks consist of granite and andesitic dike, and are covered by Quaternary fluvial deposits. The fault (white arrows) is developed along the boundary between dike and granite, and cuts fluvial deposits(hoe for scale). Note the sampling locations and age dating results (OSL and 10Be). (b) Sub-rounded, crudely cross-stratified sand-granule deposit (Qa) above the basement of the hanging wall side (lens cap for scale). (c) Sub-angular and poorly sorted pebble to cobble fluvial deposits (Qb) above the basement of the footwall side. Fault trace(white arrows) penetrates into the Qa and Qb deposits.
3. 연구방법
단층의 기하학적 특성 및 운동학적 특성을 파악하기 위해 노두를 정밀하게 관찰하고 분석하였다. 단층에 의해 절단된 퇴적층의 변위량은 겉보기 변위이기 때문에 단층면과 단층조선, 그리고 노출된 단면의 상관관계를 바탕으로 삼각함수에 기초해 실변위량을 계산할 수 있다(Xu et al., 2009). 여기서는 Nieto-Fuentes et al. (2014)가 개발한 JAVA소프트웨어(TruDisp 1.0)를 이용해 단층의 실변위량을 계산하였다.
단층에 의해 절단된 퇴적층의 연대를 도출하기 위해 광여기루미네선스(Optically Stimulated Luminescence; OSL) 연대측정을 실시하였다. 광여기루미네선스 연대측정법은 퇴적층 내에 포함된 석영입자(혹은 장석입자)에서 방출되는 루미네선스 신호를 이용한 측정법으로 주로 퇴적층의 퇴적시기를 규명하는데 활용된다. 단층 상반의 Qa 퇴적층은 사층리가 잘 발달하며, 입자가 상대적으로 세립질로 분급이 양호하고 원마도가 좋다. 해당 층의 동일 층준에서 두 개의 시료를 채취하였다(그림 3a; WWS_2-1, 2-1). 단층 하반의 Qb 자갈퇴적층의 경우, 분급이 매우 불량하고 자갈의 크기가 커서 파이프를 이용한 정상적인 시료채취가 불가능하였다. 따라서 해당 층에 대해서는 햇빛에 노출되어 있는 표면을 제거한 후 퇴적층 기질부의 사질 퇴적물을 긁어 벌크(bulk) 시료를 채취하였다. 시료가 햇빛에 노출되는 것을 방지하기 위해 시료채취는 야간에 실시하였다(그림 3a; WWS_2-3, 2-5).
시료채취에 사용된 파이프는 암실에서 절개하여 양 끝부분은 연간선량 및 수분함량 측정에 사용하였고, 햇빛에 최대한 노출되지 않은 가운데 부분에서 연대측정에 필요한 석영입자들을 분리하였다. 벌크시료는 일부 연간선량 측정 및 수분함량 측정에 사용할 부분을 제외한 나머지 부분을 이용하여 석영입자를 분리하였다. 등가선량 측정은 채취한 시료로부터 분리한 석영입자에 단일시료재현법(Single-Aliquot Regenerative-Dose protocol, SAR protocol)을 적용하였다. 전처리가 완료된 시료는 한국기초과학지원연구원(KBSI)의 루미네선스 자동측정장비(Risø TL/OSL-DA-20)를 이용하여 분석하였고, 연간선량 측정은 저준위 고분해능 감마스펙트로미터를 사용하였다.
광여기루미네선스 연대결과의 교차검증을 위해 우주선유발 동위원소를 이용한 연대측정 방법의 하나인 10Be을 이용하여 하안단구면 지형면 형성시기를 추정하였다. 10Be 연대 측정시로로 거력을 채취하였으며 이 거력은 하안단구면이 형성된 이후 현재의 자리에 위치하였으며, 현재의 자리에 위치한 이후 뒤집히거나 다른 외부의 영향을 받지 않았다고 가정하였다. 따라서 이러한 가정에 적합한 직경 약 1 m 이상의 거력을 획득하였으며, 신뢰도 확보를 위해 각기 다른 지점에서 2점을 채취하였다(그림 3a; WWS001, 002). 10Be 연대 측정시료의 전처리는 고려대학교 연대측정연구실에서 실시하고, 한국과학기술원(KIST)의 6MV AMS를 이용하여 10Be의 농도를 측정하였다(Seong et al., 2016). 이를 바탕으로 Cronus-Earth online calculator (Balco et al., 2008)를 이용하여 최종적인 지형면 노출연대를 계산하였다.
4. 연구결과
4.1 원원사단층
원원사단층 제2지점은 북북동-남남서 방향으로 발달하는 직선상 계곡의 동편에서 발견되었다(Gwon et al., 2018, 2019). 기반암을 관입한 안산암질 암맥은 암녹색 내지 담회색을 띠고 있으며 장석반정이 관찰된다. 암맥은 N41°E/38°NW의 자세로 관입하고 있으며, 폭은 약 40 cm이다. 기반암에는 최소 4매 이상의 단층이 발달하고 있다. 이 단층들의 자세는 북서-남동~북북서-남남동 방향으로 주향하거나 서북서-동남동~동북동-서남서 방향으로 주향하며, 이들은 대부분 북서쪽으로 20-53°로 경사한다. 이들 중 안산암질 암맥의 동쪽 경계면을 따라 단층이 발달하며, 이 단층은 기반암 상위에 발달하는 제4기 하안단구 퇴적층을 절단하여 상반의 기반암이 제4기층을 충상하는 역단층의 운동감각을 보인다. 단층면에 발달하는 단층조선(34°/280°)을 통해 추정된 단층의 운동감각은 우수향-역이동성 사교이동단층이다. 야외에서 측정된 겉보기 수직변위는 기반암과 제4기 퇴적층의 부정합면을 기준으로 약 42 cm이다.
4.2 연대측정결과
원원사단층 제2지점 상반의 퇴적층(Qa)에서 채취된 두 시료 WWS_2-1과 WWS_2-2의 광여기루미네선스 연대측정 결과는 각각 6.9±0.6 ka와 5.6±0.8 ka로 오차범위 내에서 일치한다(표 1; 그림 3a). 또한 단층 하반의 퇴적층(Qb)에서 채취한 두 시료 WWS_2-3과 WWS_2-5에 대한 광여기루미네선스 연대측정 결과도 각각 3.6±0.3 ka와 3.6±0.4 ka로 오차범위 내에서 일치한다(그림 3a). 이러한 결과는 각 퇴적층에서 실시한 광여기루미네선스 연대의 신뢰도가 높다는 것을 간접적으로 지시한다. 지표와 인접한 단층 상반에서 채취한 시료의 경우(WWS_2-1과 2-2), 시료채취 과정에서 현생 퇴적물이 유입되었을 가능성이 존재하지만 두 시료의 등가선량 분포와 연대결과가 유사한 것으로 보아, 현생 퇴적물의 오염으로 인한 영향은 크지 않을 것으로 판단된다(그림 4a, 4b). 더불어 WWS_2-3과 WWS_2-5는 Qb 퇴적층의 특성상 파이프로 획득하지 못해, 사질 퇴적물을 선별하여 얻어진 시료이나 두 시료의 등가선량 분포가 상당히 유사한 것을 확인할 수 있다(그림 4c, 4d). 이는 파이프를 이용할 때보다 넓은 영역에서 시료가 채취되었음을 고려한다면, 해당 퇴적층의 석영 입자들이 비슷한 시기에 퇴적되었음을 의미한다.
4.3 단층변수의 도출
부정합면의 낙차는 야외에서 42 cm로 측정되나, 실제로 단층의 운동은 주향이동성분이 일부 포함되어 있으므로, 단층면의 자세와 단층조선의 관계를 바탕으로 실변위량을 계산하였다(Xu et al., 2009; Nieto-Fuentes et al., 2014). 겉보기 변위를 바탕으로 단층조선과 단층면의 각도 관계를 이용해 계산한 실변위 값은 46.4 cm로 계산되었으며, 겉보기 수평변위는 33.1 cm, 겉보기 수직변위는 25.9 cm로 각각 계산되었다(표 3). 하지만 지표작용(침식 등)의 결과로 원 지형이 수평(flat)하지 않았을 가능성을 배제할 수 없으므로, 부정합을 이용한 변위량은 실변위량으로 판단하기에 불확실성이 따른다. 하지만 명확한 변위량으로 판단될 건층이 존재하지 않으므로, 이번 연구에서는 부정합면이 수평하였을 것으로 가정하고 변위량을 제시하였다. 단층에 의해 절단된 퇴적층은 퇴적상에 기초하여 크게 두 개의 단위층으로 구분되나, 층후가 10~30 cm로 얇고, 퇴적층 내에 발달한 단층면은 단일면이기 때문에 이 노두상에서 인지되는 단층활동 횟수는 1회의 운동으로 판단된다. 즉, 1회의 운동을 통해 최소 46 cm의 실변위량이 발생한 것으로 판단된다.
5. 토 의
5.1 울산단층을 따라 발달하는 제4기 단층들과의 특성비교
Okada et al. (1998)이 말방지 북쪽면에서 울산단층을 따른 제4기 단층(말방단층)을 최초로 보고하였으며, 이후 울산단층이 통과하는 경주시 천북면에서 울산시 매곡동에 이르는 구간에서 제4기에 재활한 단층이 고지진학적 조사를 통해 계속 보고되고 있다(그림 1b; e.g., Kyung, 1997; Okada et al., 1998; Son and Ryoo, 1999; Yoon and Hwang, 1999; Chwae et al., 2000; Chang, 2001; Lee and Schwarcz, 2001; Choi, P.-Y. et al., 2002; Lee et al., 2002; Ryoo et al., 2001, 2002, 2004; Choi, 2003; Kee et al., 2009; Ryoo, 2009; Choi, S.-J. et al., 2012, 2014; Kim et al., 2020). 일부 단층노두를 제외하고 울산단층을 따라 기 보고된 제4기 단층들은 대부분 다음과 같은 공통된 특성을 갖고 있다. 동측산지에서 쏟아져 내린 제4기 선상지 퇴적층 내지 하천퇴적층을 동쪽의 기반암(주로 화강암 내지 관입암)이 충상하고 있으며, 단층의 기하는 N-S, NNW-SSE, NE-SW의 주향을 가지며, 동쪽으로 30-70° 경사한다. 이는 울산단층의 동측지괴가 현생응력조건에서 서쪽으로 충상하는 양상(top-to-the-west)으로 해석할 수 있다. 단층에 의해 절단된 퇴적층은 연대측정 상한을 넘어서는 경우도 있지만, 제4기의 연대결과가 다수 도출되었다(Choi et al., 2012). 단층들의 단일변위량이 도출된 경우는 대부분 단일변위량이 1 m 내외이며, 누적변위량은 수 십 미터에서 백 미터 이상으로 해석된 사례도 있다(e.g., Choi et al., 2002).
반면, 원원사단층 제1지점과 이번 연구대상인 원원사단층 제2지점의 역단층은 NNW-SSE ~ NE-SW방향으로 주향하고, 서쪽으로 저각의 경사를 보인다. 단층 동측 지괴의 융기를 주도한 울산단층대 내 단층들이 주로 동쪽으로 경사하는 반면, 원원사단층 지점에서 발달하는 단층은 울산단층에서 보고된 다른 역단층들과 달리 서쪽으로 경사하고 있어, 울산단층의 반향 역단층(back thrust)으로 해석된다(Gwon et al., 2019). 반향 역단층은 주 단층에 수반되어 발달하는 부차적인 단층이기 때문에 일반적으로 그 규모가 상대적으로 작다. 따라서 원원사단층 제2지점의 단일 변위량이 다른 지점에서 보고된 단일변위량(1 m 내외)보다 작은 것은 반향단층으로 발달하기 때문인 것으로 해석할 수 있다.
원원사단층 제1지점과 제2지점은 동일한 하천에서 약 100 m 거리를 두고 발견되며, 두 단층의 주향과 발달고도 등을 고려했을 때, 동일한 단층일 가능성이 있다. 하지만 원원사단층 제1지점의 단층규모, 변위량 등이 원원사 제2지점과 비교해 크기 때문에, 원원사단층 제2지점은 원원사단층 제1지점에서 분기된 소단층일 가능성도 배제할 수 없다. 하지만 단층에 의한 지표변형의 지형학적 증거가 부족하며, 향후 연구에서 정밀한 지형분석과 굴착조사 결과 등 충분한 자료가 뒷받침 되어야만 이 두 지점의 관계를 이해할 수 있을 것으로 판단된다.
5.2 역전된 연대결과
단층 상하반의 부정합면 바로 직상부 퇴적층의 양상이 단층을 기준으로 급격하게 달라지는 데에는 두 가지 가능성을 생각할 수 있다. 첫째로는 주향이동성분에 의해 Qa와 Qb가 접했을 가능성이 있다. 하지만 이는 단층의 자세, 운동학적특성, 단층조선의 특성을 비춰봤을 때, 수평이동성분보다는 경사이동성분이 우세하고, 지형적으로 산지곡부를 흐르는 직선형하천이므로 하천의 만곡도(sinuosity)가 크지 않아 횡적인 변화가 급격하지 않을 것으로 판단되므로, 해석에 신뢰도가 떨어진다. 또 다른 가능성은 입도가 크고 분급이 불량한 퇴적층(Qb)이 기존의 퇴적층(Qa)를 침식한 후 단층작용이 일어났을 가능성이 있다. 유수에 의해 분급이 좋고 사층리가 잘 발달하는 퇴적층(Qa)이 퇴적된다(그림 5의 stage 1). 이러한 퇴적상은 유수에 의한 일반적인 퇴적상으로 해석된다(e.g., Spalletti and Pinol, 2019). 이후 대규모 홍수 등의 영향으로 입도가 크고 분급이 불량한 퇴적층(Qb)이 기존의 퇴적층의 일부를 침식하며 퇴적되었을 것이다(그림 5의 stage 2). 이러한 해석은 유수의 에너지에 따른 퇴적상의 변화를 반영하여 해석하였다(e.g., Hauer and Pulg, 2018). 이후 단층에 의해 변위되면서, 상대적으로 젊은 퇴적층(Qb)이 Qa층의 아래에 놓이게 되고(그림 5의 stage 3), 지속적인 침식작용으로 평탄한 면이 형성된다(그림 5의 stage 4). 마지막으로 하방침식에 의해 현재의 노두양상이 발달했을 것으로 해석된다. 본 연구에서는 후자의 가능성이 높을 것으로 평가하나, 단편적인 노두상황으로 해석하기에는 다소 무리가 있다.

Block diagrams illustrating a possible paleoseismological interpretation at the second site of the Wonwonsa fault.
단층의 최후기 활동시기를 규명하기 위해 실시한 광여기루미네선스 연대측정 결과는 Qb층에서 약 3.6 ka 내외, Qa층에서 약 6 ka 내외의 연대가 도출되었다. 제4기 단층을 기준으로 하반의 기반암 상위에 놓여있는 Qb층이 상반 기반암 상위에 발달하는 Qa 퇴적층보다 젊은 연대를 지시하고 있다. 이러한 연대결과는 역단층운동의 결과로 오래된 상반의 퇴적층이 충상하여 발생한 것으로 해석할 수 있다. 하지만 지형면의 형성연대인 9 ka 내외를 보이는 거력의 하부에 놓여있는 퇴적층의 매몰연대가 3.6 ka로 도출된 것은 논리적으로 설명하기 어려우며, 아래의 세 가지 가능성을 생각할 수 있다.
첫째는, 연대측정 결과의 오류 혹은 시료의 오염가능성이다. 지표와 인접하며 층후가 얇은 Qa 퇴적층에서 채취한 시료의 경우, 외부로부터 오염가능성을 배제할 수 없다. 또한 Qb 퇴적층에서 채취한 시료의 경우, 파이프를 이용한 시료채취보다 넓은 영역에서 시료채취가 이루어졌기 때문에 서로 다른 퇴적시기를 가지는 석영입자가 포함되었을 가능성이 크다. 하지만 이러한 한계점을 극복하기 위해 동일 층준에서 각 두 개의 시료를 획득하였고, 그 결과가 서로 일치한다(표 1; 그림 3). 즉, 기술적으로 시료의 오염여부는 확인할 수 없으나, 동일 층준에서 같은 연대가 도출되어, 광여기루미네선스 연대결과 자체의 신뢰도는 높은 것으로 판단된다.
두 번째 가능성은 우주선유발 동위원소 샘플인 거력이 마지막 퇴적 이전에 가지고 있던 기존의 노출 시에 형성된 유전량(inheritance)이 포함될 가능성도 있다. 연구지역이 급경사를 가진 산지하천이라는 점을 고려하면 거력의 공급은 고강도의 사건에 의해 유입되었으므로, 유전량이 크지 않을 것으로 추측되지만 소량의 유전량을 배제할 수는 없다. 즉, 거력이 기반암의 형태로 급경사의 사면에 있을 때, 노출되어 축적되고 있는 유전량에 의해 노출연대가 상대적으로 오래된 연대를 지시하였을 가능성이 있다. 이를 확인하기 위해서는 인접한 거력에서 많은 시료를 획득하여 유전량에 대한 불확실성을 좁히는 방법이 필요하였으나, 본 연구에서는 이를 수행하지 못했다. 추후 많은 시료에 대한 우주선유발 동위원소 연대측정 결과를 얻을 수 있다면, 보다 정확한 지형면의 형성시기를 획득할 수 있을 것으로 판단된다. 따라서, 10Be 노출연대는 단구면 형성의 최대(maximum) 연대로 여기는 것이 타당하다.
세 번째는 연대측정 방법 사이에서 발생하는 차이일 수 있다. 10Be을 이용한 연대측정 결과 두 시료가 오차범위 내에서 동일한 연대가 도출되었으며, 연대결과에 대한 신뢰도는 높은 것으로 판단된다. 광여기루미네선스 연대 결과 역시 동일층준에서 두 개씩 획득한 결과가 거의 오차없이 도출되었고, 광여기루미네선스 등가선량의 분포가 비슷하여 외부로부터의 오염이 거의 없이 퇴적시기를 지시할 것으로 판단된다. 연대측정법 자체는 확률론적 가정과 해석이 포함되는데, 매몰연대를 지시하는 광여기루미네선스 연대측정법과 노출연대를 지시하는 10Be 연대측정법의 근본적인 차이에서 발생하는 변수가 존재할 수 있다. 하지만 결과적으로 두 연대측정 결과가 모두 홀로세(1만 년 이내)를 지시하므로, 이 단층은 약 3천 년 전 이후 또는 약 9천 년 전 이후에 활동한 것으로 해석할 수 있다. 향후 단층의 연장부에서 굴착 조사 또는 추가적인 연대측정 결과가 뒷받침된다면, 보다 신뢰도 높은 단층활동 이력을 도출할 수 있을 것으로 기대된다.
6. 결 론
원원사단층 제2지점은 단층운동 연대가 상당히 젊은 역단층으로 비교적 신뢰도 높은 변위량과 연대가 도출되었다. 이 단층의 자세와 겉보기 변위를 통해 계산된 실변위량은 약 46 cm이나, 이는 건층으로 측정된 변위가 아니므로 불확실성이 존재한다. 절단된 퇴적층의 연대측정 결과에 기반하면, 단층의 활동시기는 약 3천 년 전 이후 또는 9천 년 전 이후로 추정되었다. 하지만 우주선유발 동위원소를 통한 노출연대는 기존의 유전량을 배제할 수 없으므로, 광여기루미네선스 연대결과를 활용한다면, 이 단층의 최후기 활동시기는 약 3,600년 전 이후로 해석할 수 있다. 이 결과는 울산단층을 따라 기존 보고된 제4기단층의 최후기 활동시기 중 가장 젊은 것이므로 그 의미가 있다(Choi et al., 2012). 하지만 절단되지 않은 퇴적층이 부재하여, 단층활동시기를 제한할 수 없으며, 연대측정간의 차이 혹은 유전량에 의한 연대차이가 발생하므로 불확실성이 있다. 추후 단층의 연장부에서 정밀한 굴착조사를 통해 연대결과를 보완할 필요가 있다. 신뢰도 높은 단층변수정보가 축적된다면, 향후 확률론적 지진재해도 평가 및 지진예측 연구에 큰 도움을 줄 수 있을 것으로 판단된다.
Acknowledgments
연구는 산업통상자원부(MOTIE)와 한국에너지기술평가원(KETEP)의 연구사업(No.20201510100020)으로 수행되었습니다. 이 논문을 투고하기까지 세심한 검토와 건설적인 조언을 해주신 두 분의 심사위원님과 편집위원장님께 진심으로 감사드립니다. 논문의 질적향상을 위해 건설적인 토의를 해주신 최진혁 박사님, 최이레 박사님, 부경대학교 지질구조재해연구실원들에게 감사의 뜻을 전합니다.
References
-
Back, J.J., Kyung, J.B. and Choi, H., 2011, Analysis on the source characteristics of the recent five-year earthquakes occurred in the central and western areas of the Korean Peninsula. Journal of Korean Earth Science Society, 32, 161-169 (in Korean with English abstract).
[https://doi.org/10.5467/JKESS.2011.32.2.161]
- Bae, S., 2005, Characteristics of initial rock stress state in Korean tectonic provices by hydro fracturing stress measurement. Ph.D. Thesis, Seoul National University, Korea, 223 p (in Korean with English abstract).
-
Balco, G., Stone, J.O., Lifton, N.A. and Dunai, T.J., 2008, A complete and easily accessible means of calculating surface exposure ages or erosion rates from 10Be and 26Al measurements. Quaternary geochronology, 3, 174-195.
[https://doi.org/10.1016/j.quageo.2007.12.001]
-
Chang, C., Lee, J.B. and Kang, T.-S., 2010, Interaction between regional stress state and faults: complementary analysis of borehole in situ stress and earthquake focal mechanism in southeastern Korea. Tectonophysics, 485, 164-177.
[https://doi.org/10.1016/j.tecto.2009.12.012]
- Chang, T.W., 2001, Quaternary Tectonic Activity at the Eastern Block of the Ulsan Fault. Journal of the Geological Society of Korea, 37, 431-444 (in Korean with English abstract).
-
Cheon, Y., Ha, S., Lee, S., Cho, H. and Son, M., 2017, Deformation features and history of the Yangsan Fault Zone in the Eonyang-Gyeongju area, SE Korea. Journal of the Geological Society of Korea, 53, 95-114 (in Korean with English abstract).
[https://doi.org/10.14770/jgsk.2017.53.1.95]
- Cho, H., Kang, T.-S. and Kyung, J.B., 2006, Focal mechanism solutions of microearthquakes in the southwestern part of the Korean Peninsula. Journal of Korean Earth Science Society, 27, 341-347 (in Korean with English abstract).
-
Choi, J.-H., Ko, K., Gihm, Y.S., Cho, C.S., Lee, H., Song, S.G., Bang, E.-S., Lee, H.-J., Bae, H.-K., Kim, S.W., Choi, S.-J., Lee, S.S. and Lee, S.R., 2019, Surface deformations and rupture processes associated with the 2017 Mw5.4 Pohang, Korea, earthquake. Bulletin of the Seismological Society of America, 109, 756-769.
[https://doi.org/10.1785/0120180167]
- Choi, P.-Y., Lee, C.-B., Ryoo, C.-R., Choi, Y.S., Kim, J.-Y., Hyun, H.-J., Kim, Y.-S., Kim, J.-Y. and Chwae, U., 2002, Geometric analysis of the Quaternary Malbang Fault: Interpretation of Borehole and Surface data. Journal of the Geological Society of Korea, 38, 163-174 (in Korean with English abstract).
- Choi, S.-J., Ghim, Y.S., Cheon, Y. and Ko, K., 2019, The first discovery of Quaternary fault in the Western part of the South Yangsan fault - Sinwoo site. Economic and Environmental Geology, 52, 251-258 (in Korean with English abstract).
-
Choi, S.-J., Jeon, J.-S., Choi, J.-H., Kim, B., Ryoo, C.-R., Hong, D.-G. and Chwae, U., 2014, Estimation of possible maximum earthquake magnitudes of Quaternary faults in the southern Korean Peninsula. Quaternary International, 344, 53-63.
[https://doi.org/10.1016/j.quaint.2014.05.052]
- Choi, S.-J., Jeon, J.-S., Song, K.-Y., Kim, H.-C., Kim, Y.-H., Choi, P.-Y., Chwae, U.C., Han, J.-G., Ryoo, C.-R., Sun, C.-G., Jeon, M.S., Kim, G.-Y., Kim, Y.-B., Lee, H.-J., Shin, J.S., Lee, Y.-S. and Kee, W.-S., 2012, Active faults and seismic hazard map. NEMA, Seoul, 882 p (in Korean with English abstract).
- Choi, S.-O., Park, C., Synn, J.-H. and Shin, H.-S., 2008, A decade's experiences on the hydrofracturing in-situ stress measurement for tunnel construction in Korea. Proceedings of the Korean Society for Rock Mechanics Conference Spring 2008, 79-88 (in Korean with English abstract).
- Choi, W.-H., 2003, Neotectonics of the Gyeongju-Ulsan area in the southeastern part of Korean Peninsula. Ph.D. thesis, Seoul National University, Korea, 205 p (in Korean with English abstract).
- Chwae, U., Choi, S.-J., Lee, B., Lee, Y., Ryoo, C.-R. and Song, M., 2000, Final report for Neotectonics research. Ministry of Science and ICT, N2-01-02-A-01, 590.
- Gwon, S., Cho, S.-I. and Choi, W.-H., 2018, Characteristics of the fault movement during Quaternary, Wonwonsa 2nd fault, Oedong-Eup, Gyeongju-Si. Proceedings of KSEG 2018 Fall Conference, 8-9th Nov, Gwangju, 129 p (in Korean).
- Gwon, S., Cho, S.-I. and Choi, W.-H., 2019, Determination of the last slip of the Wonwonsa fault through the age dating results. 2019 Joint Conference of the Geological Science & Technology of Korea, April 17-19, Jeju, 294 p (in Korean).
-
Haimson, B.C., Lee, M.Y. and Song, I., 2003, Shallow hydraulic fracturing measurements in Korea support tectonic and seismic indicators of regional stress. International Journal of Rock Mechanics and Mining Sciences, 40, 1243-1256.
[https://doi.org/10.1016/S1365-1609(03)00119-9]
-
Hauer, C. and Pulg, U., 2018, The non-fluvial nature of Western Norwegian rivers and the implications for channel patterns and sediment composition. Catena, 171, 83-98.
[https://doi.org/10.1016/j.catena.2018.06.025]
-
Hoe, S.Y. and Kyung, J.B., 2008, Fault Plane Solutions for the Recent Earthquakes in the Central Region of South Korea. Journal of Korean Earth Science Society, 29, 437-445 (in Korean with English abstract).
[https://doi.org/10.5467/JKESS.2008.29.5.437]
-
Jin, K. and Kim, Y.-S., 2020, Importance of the archaeoseismological study for earthquake geology in South Korea. Journal of the Geological Society of Korea, 56, 251-264 (in Korean with English abstract).
[https://doi.org/10.14770/jgsk.2020.56.2.251]
-
Jin, K., Lee, M., Kim, Y.-S. and Choi, J.-H., 2011, Archaeoseismological studies on historical heritage sites in the Gyeongju area, SE Korea. Quaternary International, 242, 158-170.
[https://doi.org/10.1016/j.quaint.2011.03.055]
- Jun, M.-S. and Jeon, J.S., 2010, Focal mechanism in and around the Korean Peninsula. Geophysics and Geophysical Exploration, 13, 198-202 (in Korean with English abstract).
-
Jung, M.K. and Kyung, J.B., 2013, Source characteristics of the recent earthquakes for seven years in the southwestern region of the Korean Peninsula. Journal of Korean Earth Science Society, 34, 59-68 (in Korean with English abstract).
[https://doi.org/10.5467/JKESS.2013.34.1.59]
- Kee, W.-S., Kim, B.C., Hwang, J.H., Song, K.-Y. and Kihm, Y.-H., 2007, Structural characteristics of Quaternary reverse faulting on the Eapcheon Fault, SE Korea. Journal of the Geological Society of Korea, 43, 311-333 (in Korean with English abstract).
- Kee, W.-S., Kim, Y.-H., Lee, H.-J., Choi, D.-L., Kim, B.-C., Song, K.-Y., Koh, H.-J., Lee, S.R., Gwang, Y.Y., Hwang, S.-H., Park, K.-G. and Sung, N.-H., 2009, South eastern fault variable research and DB Construction. KIGAM, Daejeon, 327.
-
Kim, K.-H., Ree, J.-H., Kim, Y.H., Kim, S., Kang, S.Y. and Seo, W., 2018, Assessing whether the 2017 MW 5.4 Pohang earthquake in South Korea was an induced event. Science, 360, 1007-1009.
[https://doi.org/10.1126/science.aat6081]
-
Kim, M.-C., Gihm, Y.S., Son, E.-Y., Son, M., Hwang, I.G., Shinn, Y.J. and Choi, H., 2015, Assessment of the potential for geological storage of CO2 based on structural and sedimentologic characteristics in the Miocene Janggi Basin, SE Korea. Journal of the Geological Society of Korea, 51, 253-271 (in Korean with English abstract).
[https://doi.org/10.14770/jgsk.2015.51.3.253]
-
Kim, M.-C., Jung, S., Yoon, S., Jeong, R.-Y., Song, C.W., and Son, M., 2016, Neotectonic Crustal Deformation and Current Stress Field in the Korean Peninsula and Their Tectonic Implications: A Review. The Journal of the Petrological Society of Korea, 25, 169-193 (in Korean with English abstract).
[https://doi.org/10.7854/JPSK.2016.25.3.169]
- Kim, S.G., 1980, Seismicity of the Korean Peninsula and its vicinity. Journal of the Korean Institute of Mining Geology, 13, 51-63 (in Korean with English abstract).
- Kim, S.K., Jun, M.-S. and Jeon, J.-S., 2006, Recent research for the seismic activities and crustal velocity structure. Economic and Environmental Geology, 39, 369-384 (in Korean with English abstract).
-
Kim, T., Shin, H.-C. and Kim, Y.-S., 2020, Characteristics of the topographical deformation the central part of the Ulsan fault. Journal of the Geological Society of Korea, 56, 193-209 (in Korean with English abstract).
[https://doi.org/10.14770/jgsk.2020.56.2.193]
-
Kim, Y.H., He, W.H., Ni, S.D., Lim, H. and Park, S.-C., 2017, Earthquake Source Mechanism and Rupture Directivity of the 12 September 2016 MW 5.5 Gyeongju, South Korea, Earthquake. Bulletin of the Seismological Society of America, 107, 2525-2531.
[https://doi.org/10.1785/0120170004]
-
Kim, Y.-S., 2020, Research trend of active fault and earthquake geology for the Korean Peninsula. Journal of the Geological Society of Korea, 56, 109-111 (in Korean with English abstract).
[https://doi.org/10.14770/jgsk.2020.56.2.109]
- Kim, Y.-S., Jin, K., Choi, W.-H. and Kee, W.-S., 2011, Understanding of active faults: A review for recent researches. Journal of the Geological Society of Korea, 47, 723-752 (in Korean with English abstract).
-
Kim, Y.-S., Son, M., Choi, J.-H., Choi, J.-H., Seong, Y.B., and Lee, J., 2020, Processes and challenges for the production of Korean active faults map. Journal of the Geological Society of Korea, 56, 113-134 (in Korean with English abstract).
[https://doi.org/10.14770/jgsk.2020.56.2.113]
- KMA, 2014, HIstorical Earthquake Records in Korea. Korean Meteorological Archives Series, 5, 291.
- Kyung, J.B., 1997, Paleoseismological study on the Mid-northern part of Ulsan Fault by trench method. The Journal of Engineering Geology, 7, 81-90 (in Korean with English abstract).
- Kyung, J.B., Lee, K. and Okada, A., 1999a, A paleoseismological study of the Yangsan fault - analysis of deformed topography and trench survey. Journal of the Korean Geophysical Society, 2, 155-168 (in Korean with English abstract).
- Kyung, J.B., Lee, K., Okada, A., Watanabe, M., Suzuki, Y. and Takemura, K., 1999b, Study of Fault Characteristics by Trench Survey in the Sangchon-ri Area in the Southern Part of Yangsan Fault, Southeastern Korea. Journal of Korean Earth Science Society, 20, 101-110 (in Korean with English abstract).
-
Lee, H.-K. and Schwarcz, H.P., 2001, ESR dating of the subsidiary faults in the Yangsan fault system, Korea. Quaternary Science Reviews, 20, 999-1003.
[https://doi.org/10.1016/S0277-3791(00)00055-X]
-
Lee, J., Rezaei, S., Hong, Y., Choi, J.-H., Choi, J.-H., Choi, W.-H., Rhee, K.-W. and Kim, Y.-S., 2015, Quaternary fault analysis through a trench investigation on the northern extension of the Yangsan fault at Dangu-ri, Gyungjusi, Gyeongsanbuk-do. Journal of the Geological Society of Korea, 51, 471-485 (in Korean with English abstract).
[https://doi.org/10.14770/jgsk.2015.51.5.471]
- Lee, Y.-H., Son, M., Ryu, C.-R., Kim, I.-S. and Choe, W.-C., 2002, Quaternary faults along the Ulsan fault: Gaegok, Hwalseongri, and Jinti. Proceedings of the Korean Earth Science Society spring meetings, 36 p (in Korean).
-
Lifton, N., Sato, T. and Dunai, T.J., 2014, Scaling in situ cosmogenic nuclide production rates using analytical approximations to atmospheric cosmic-ray fluxes. Earth and Planetary Science Letters, 386, 149-160.
[https://doi.org/10.1016/j.epsl.2013.10.052]
- McCalpin, J.P., 1996, Paleoseismology. Academic Press, San Diego, 588.
-
McKenzie, D., 1972, Active Tectonics of the Mediterranean Region. Geophysical Journal International, 30, 109-185.
[https://doi.org/10.1111/j.1365-246X.1972.tb02351.x]
-
Nieto-Fuentes, R., Nieto-Samaniego, A.F., Xu, S.-S. and Alaniz-Alvares, S.A., 2014, Software for determining the trud displacement of faults. Computers and Geosciences, 64, 35-40.
[https://doi.org/10.1016/j.cageo.2013.11.010]
-
Nishiizumi, K., Imamura, M., Caffee, M., Southon, J., Finkel, R. and McAninch, J., 2007, Absolute calibration of 10Be AMS Standards. Nuclear Instruments and Methods in Physical Research Section, B: Beam Interactions with Materials and Atoms, 258, 403-413.
[https://doi.org/10.1016/j.nimb.2007.01.297]
-
Okada, A., Watanabe, M., Suzuki, Y., Kyung, J.B., Jo, W.R., Kim, S.K., Oike, K. and Nakamura, T., 1998, Active fault topography and fault outcrops in the central part of the Ulsan fault system, southeast Korea. Journal of Geography, 107, 644-658 (in Japanese with English abstract).
[https://doi.org/10.5026/jgeography.107.5_644]
-
Park, J., Kim, T. and Kim, Y., 2014, Characteristics of natural gamma distribution in the southern Korean Peninsula. Journal of the Geological Society of Korea, 50, 783-796 (in Korean with English abstract).
[https://doi.org/10.14770/jgsk.2014.50.6.783]
-
Park, J.C., Kim, W., Chung, T.W., Baag, C.E. and Ree, J.H., 2007, Focal mechanism of recent earthquakes in the Southern Korean Peninsula. Geophysical Journal International, 169, 1103-1114.
[https://doi.org/10.1111/j.1365-246X.2007.03321.x]
- RGAFJ (The Research Group for Active Faults of Japan), 2011, Maps of Active Faults in Japan with an Explanatory Text. University of Tokyo Press.
- Ryoo, 2009, A Report for the Quaternary Gaegok 6 Fault Developed in the Mid-eastern Part of Ulsan Fault Zone, Korea. Economic and Environmental Geology, 42, 635-643 (in Korean with English abstract).
- Ryoo, C.-R., Lee, B.-J., Chwae, U., Choi, S.-J. and Son, M., 2001, Discussion of the newly reported Quaternary fault at Singye and Hwalseonri, Oedong-eup, Gyeongju, Eastern part of the Ulsan fault. Annual Conference of the Geological Society of Korea (Abstracts), Kyungpook National University, Daegu, October 26-27, 1 p (in Korean).
- Ryoo, C.-R., Lee, B.J., Son, M., Lee, Y.H., Choi, S.-J. and Chwae, U., 2002, Quaternary faults in Gaegok-ri, Oedongeup, Gyeongju, Korea. Journal of the Geological Society of Korea, 38, 309-323 (in Korean with English abstract).
- Ryoo, C.-R., Son, M., Lee, Y.H., Choi, S.-J. and Chwae, U., 2004, Quaternary faults around Bulguk-sa, Gyeongju, Korea. KIGAM Bulletin, 8, 3-17 (in Korean with English abstract).
-
Scholz, C.H., 2002, The mechanics of earthquakes and faulting. Cambridge University Press, Cambridge, UK, 471.
[https://doi.org/10.1017/CBO9780511818516]
- Scholz, C.H., Aviles, C.A. and Wesnousky, S.G., 1986, Scaling differences between large interplate and intraplate earthquakes. Bulletin of the Seismological Society of America, 76, 65-70.
- Slemmons, D.B. and McKinney, R., 1977, Definition of “Active fault”. U.S Army Engineer Waterways Experiment Station Soils and Pavements Laboratory miscellaneous paper S-77-8, 1-22.
-
Seong, Y.B., Dorn, R.I. and Yu, B.Y., 2016, Evaluating the life expectancy of a desert pavement. Earth-Science Reviews, 162, 129-154.
[https://doi.org/10.1016/j.earscirev.2016.08.005]
-
Sibson, R.H., 1985, A note on fault reactivation. Journal of Structural Geology, 7, 751-754.
[https://doi.org/10.1016/0191-8141(85)90150-6]
- Son, M. and Ryoo, C.-R., 1999, Quaternary faults in the northeastern part of the Ulsan Fault zone, Korea. Journal of the Korean Geomorphological Association, 6, 81 (in Korean with English abstract).
-
Song, W., Ha, S., Lee, S., Kang, H.-C., Choi, J.-H. and Son, M., 2020, Quaternary structural characteristics and paleoseismic interpretation of the Yangsan Fault at Dangu-ri, Gyeongju-si, SE Korea, through trench survey. Journal of the Geological Society of Korea, 56, 155-173 (in Korean with English abstract).
[https://doi.org/10.14770/jgsk.2020.56.2.155]
-
Spalletti, L.A. and Pinol, F.C., 2019, Architecture of intereruptive and syneruptive facies in an Andean Quaternary palaeovalley: the Huarenchenque Formation, western Argentina. Andean Geology, 46, 471-489.
[https://doi.org/10.5027/andgeoV46n3-3170]
-
Stone, J.O., 2000, Air pressure and cosmogenic isotope production. Journal of Geophysical Research: Solid Earth, 105(B10), 23753-23759.
[https://doi.org/10.1029/2000JB900181]
-
Sykes, L.R., 1978, Intraplate seismicity, reactivation of preexisting zones of weakness, alkaline magmatism, and other tectonism postdating continental fragmentation. Reviews of Geophysics, 16, 621-688.
[https://doi.org/10.1029/RG016i004p00621]
- U.S. AEC (U.S. Atomic Energy Commission) to 10 CFR Part 100 Appendix A, 1971, Nuclear power plants; seismic and geologic siting criteria: Federal Register, 36, 22-602.
-
Xu, S., Nieto-Samaniego, A.F. and Alaniz-Álvarez, S.A., 2009, Quantification of true displacement using apparent displacement along an arbitrary line on a fault plane. Tectonophysics, 467, 107-118.
[https://doi.org/10.1016/j.tecto.2008.12.004]
- Yang, J.S., 2006, Quaternary fault activity in the southeastern part of the Korean Peninsula. Ph.D. Thesis, Kangwon National University, Korea, 382 p (in Korean with English abstract).
- Yoon, S.-O. and Hwang, S.I., 1999, The Active Fault Topography of the Northern Part of the Bulguksa Fault System in Kyungju City , Southeastern Korea. Journal of the Korean Geographical Society, 34, 231-246 (in Korean with English abstract).