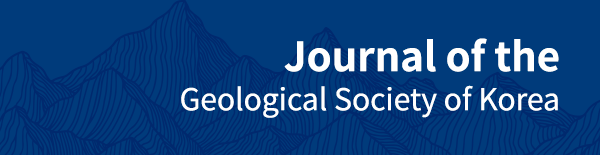
Qualities of groundwater source used for production of commercial bottled waters in Korea
Abstract
Due to skepticism among the population regarding the quality of municipal piped water, the production and consumption of commercial bottled water have been largely increasing in Korea since 1995. This is when commercial sale of bottled water (known as natural mineral water), sourced from groundwater, was first permitted by the Korean government. The Drinking Water Management Act has led to regular and intensive monitoring of original groundwater quality and environmental impact survey required for commercial bottled water production. The Act enforces regular quality analyses of groundwater source and bottled water every quater. To obtain an official permit for production of bottled water, an extremely intensive environmental impact survey is mandatory, including chemical analyses of the original groundwater and an in-depth analysis of sustainable groundwater quantity. A total of 630 data sets pertaining to original groundwater quality for bottled water production, surrounding groundwater, and nearby stream water at bottled water production sites in Korea revealed that arsenic, nitrate, and fluoride are the most frequently occurring contaminants, even though their values adhere to the Korean drinking water standards. Therefore, continued monitoring and management of these contaminants is required for sustainable production of bottled water.
초록
가정에 들어오는 상수도의 수질에 대한 불신 때문에 1995년 우리나라에서 상업적인 병입수의 판매가 허용된 이후 지하수로 만든 병입수(먹는샘물)의 생산과 소비가 크게 증가하고 있다. 먹는물관리법에 의하면 상업적으로 판매되는 병입수의 생산에 사용되는 지하수는 정기적인 수질모니터링은 물론 엄격한 환경영향조사를 받아야 한다. 즉 이 법에 따라 지하수 원수와 병입수는 매 3개월 마다, 즉 년간 네 번에 걸쳐 엄격한 수질검사를 받아야 하고 또 먹는샘물(병입수) 생산허가를 받기 위해서는 지하수 원수에 대한 엄격한 화학 및 수질분석과 더불어 지속가능한 지하수개발량 등에 대한 심도 깊은 분석 조사도 통과하여야 한다. 이 연구에서는 우리나라 북부에 위치한 18개 먹는샘물공장의 원수 지하수, 주변 이용지하수 그리고 인근 지표수에 대한 630개 수질 자료를 확보하여 분석하였다. 그 결과 먹는물 수질기준을 초과하지는 않지만 가장 많이 나오는 오염물질로 비소, 질산성질소 및 불소로 나왔으며, 지속가능한 먹는샘물 생산을 위해서는 이들에 대한 지속적인 관심과 모니터링이 필요함을 시사하였다.
Keywords:
bottled water, environmental impact survey, groundwater quality, arsenic, fluoride, nitrate키워드:
병입수, 환경영향조사, 지하수질, 비소, 불소, 질산성질소1. Introduction
In 1991, there was an incident involving a phenol spill (approximately 30 tons) from an industrial complex near the Nakdong River, situated in south Korea, which was a tap water source for eight millions residents (Kim et al., 1993). The level of phenol in tap water reached up to 0.11 mg/L (Korean standard=0.005 mg/L), and the residents suffered odorous tap water for a prolonged period. After that incident, a lack of trust in the quality of tap water has prevailed among Koreans. Consequently, this has led to an active search for safer sources of drinking water.
Amid this turmoil, the Korean government established the Drinking Water Management Act (DWMA) in 1995 to officially control drinking water quality and address people’s safety concerns. With this Act, commercial sales of natural mineral waters (bottled water sourced from groundwater) were supported under the condition of a strict Environmental Impact Survey (EIS). Since the first official permit for commercial sale of bottled water in 1995, the domestic sales have steadily increased with an average rate of 4 million (M) USD per year (Lee, 2016), from 70M USD in 1995 to 826M USD in 2018. It is estimated that this domestic sale will be over 1 billion USD in 2020.
In 1995, the Ground Water Act (GWA) was formulated to control and manage groundwater resources used for all purposes (agriculture, industry, home, military) except for drinking (Lee et al., 2007). With the enactment of the GWA, the Korean government made it mandatory for groundwater developers (landowners) to conduct a Groundwater Impact Assessment (GIA) to evaluate the impact of groundwater development and devise mitigation measures. National groundwater monitoring was also carried out with the aid of this legislation (Lee and Kwon, 2016; Lee et al., 2017, 2018).
This study aims to examine the quality of the groundwater source used to manufacture bottled water in Korea and to compare it with quality of nearby groundwater wells and streams.
2. Study Area and Data Collection
2.1 Study Area and Hydrogeological Characteristics
The 18 bottled water production sites (plants) studied are located in northern (Gyeonggi and Gangwon Provinces) and middle (Chungbuk Province) parts of Korea (Fig. 1). Each site has 1 to 6 production wells (a total of 63 wells) with some distant groundwater wells (total n=53) and nearby streams (total n=62, only a few meters away from the production wells). The depth of the production wells ranges from 67.4 to 291 m with a mean value of 183.1 m (Table 1). The wells mostly tap into fractured bedrock aquifers with a few meters of upper casing and bentonite grouting. The permitted production rate per well varies from 30 to 660 m3/day with a median value of 170 m3/day.
The geology of the production sites consists of plutonic rocks (mostly granite, biotite granite, granodiorite) (38.9%), metamorphic rocks (mostly banded gneiss, biotite gneiss, green schist) (50%), and limestones (11.1%). The overburden above the bedrock is approximately 10 m, composed of a few meters of upper soils and weathered rocks. The degree of fracture development in the bedrock aquifers varies at each site.
Table 2 shows the statistics of major parameters of the production wells (n=63). The natural groundwater levels (before pumping) ranged from 2.03 to 47.69 m below the ground surface with a mean value of 15.84 m. The maximum and minimum drawdown due to groundwater pumping were 139.47 and 1.32 m (mean=57.98 m), respectively. The hydraulic conductivity for the production wells varied (four orders of magnitude), and it ranged from 4.2×10-6 to 3.0×10-2 cm/sec with a median of 3.6×10-5 cm/sec. The median value is within a typical range of fractured rocks (Freeze and Cherry, 1979) while the arithmetic mean (2.0×10-3 cm/sec) is relatively higher than that for typical fractured rock aquifers.
The storage coefficients of the aquifers, where the production wells are tapped, are between 4.4×10-6 and 1.96×10-1, with a median value of 6.0×10-3. This coefficient is higher for typical bedrock aquifers, indicating that these wells are generally highly fractured. The transmissivity of the production wells ranges from 0.69 to 984.96 m2/day (median= 4.64 m2/day), with specific capacity of 0.52-321.84 m3/day/m (median=4.45 m3/day/m). Considering the median values of specific capacity and drawdown, a volume of 289 m3 is pumped daily at each production well, which is relatively similar to the mean value of permitted production rate per well (227 m3/day; see Table 1).
2.2 Data Collection
This study focused on the water quality of production wells, groundwater wells and nearby streams. Therefore, in addition to the relevant geological and hydrogeological properties described above, we also collected water quality data including field measured parameters (water temperature, oxidation-reduction potential (ORP), dissolved oxygen (DO), electrical conductivity (EC), pH, chemical compositions, microbes, hazardous inorganic and organic materials, and aesthetic parameters), from the EIS reports for 18 bottled water production sites. We secured 630 water quality data sets for production wells (450 sets), surrounding groundwater (78 sets), and nearby streams (102 sets). It is noted that not all data sets have values for all of the above parameters.
2.3 Principal Component and Cluster Analyses
In addition to the basic statistical analysis on the collected data and plots using chemical components levels, including Piper and Gibbs diagrams, we also conducted a principal component analysis (PCA) and cluster analysis (CA). For this, we excluded the data sets with not detected (n.d) values and finally included 12 quality components: Ca2+, Mg2+, Na+, K+, HCO3-, Cl-, SO42-, NO3-, KMnO4 consumption, color, pH, and turbidity. Among the 630 data sets, only 115 data sets were selected for the analyses. We first tested the normal distribution of the data using the Shapiro-Wilk test at a 95% confidence level (Lee et al., 2009; Noshadi and Ghafourian, 2016). Furthermore, to avoid any prevailing dominance by parameters with large magnitudes and standardize the weight of each component, we conducted z-transformation on the analyzed parameters (mean=0, standard deviation=1).
PCA is a process to reduce the number of variables in data sets by computing the principal components and increasing interpretability with minimum information loss, using only the first few principal components and neglecting the rest (Jolliffe and Cadima, 2016). CA is a technique to group similar observations into a small number of clusters based on the observed values of several variables for each individual, and the grouping is represented by a dendrogram (Lee et al., 2009; Fabbrocino et al., 2019). In this study, the k-means clustering was applied to group the data sets. These multivariate analyses were conducted using the free statistical program Past 3.06 (Hammer et al., 2001).
3. Results and Discussion
3.1 Field Measured Parameters
Table 3 shows the statistical summary of field measured parameters and chemical compositions of water from production wells, surrounding groundwater wells and streams. The production well water temperatures ranged between 9.2 and 21.7℃ (mean=14.1℃); these values were similar to those of surrounding groundwater wells (range=8.2-19.5℃, mean=14.1℃; Fig. 2). Considering the groundwater temperatures (range=7.4-20.4℃, mean=14.2℃) of bedrock aquifers of Korea (Lee and Hahn, 2006), the water temperatures of production wells and surrounding groundwaters are considered to be normal and stable. The stream water temperatures, which were largely affected by atmospheric temperatures, were much more variable (range=0.7-24℃) than those of groundwater.

Box plots of field measured parameters for production well, groundwater well, and stream water: (a) water temperature (℃), (b) oxidation-reduction potential (mV), (c) dissolved oxygen (mg/L), (d) electrical conductivity (μS/cm) and (e) pH (SU).
The ORP values of production wells, indicative of redox conditions, ranged from 89.5 to 496.9 mV (mean=230.8 mV); these were very similar to those of surrounding groundwaters (range=46.2-476.8 mV, mean=232 mV) (Fig. 2). However, the ORP of stream water was relatively higher (mean=267.3 mV). The ORP of the three water bodies was all indicative of their oxic conditions (see Fig. 2). These ORP values are consistent with those of DO. The DO ranges of production wells, groundwater wells and stream water were 4.6-9.6 mg/L (mean=7.8 mg/L), 4.3-10.5 mg/L (mean=8.1 mg/L), and 6.5-11.7 mg/L (mean=10.3 mg/), respectively. The high DO values of stream waters are expected because they are in direct contact with the atmospheric air. The high DO values of groundwater wells may be explained by rapid rainfall infiltration due to very shallow well depths. However, the very high DO values of production wells tapping the bedrock aquifers are quite strange. The very high values for both ORP and DO indicate a probable intrusion of nearby stream water into the production wells. This interpretation is also supported by very low and high water temperatures of production wells, which indicate that the groundwater of production wells is affected by the stream water with much varying water temperatures.
Mean electrical conductivities of the production wells, surrounding groundwaters, and stream waters were 176, 165, and 122 μS/cm, respectively. The high EC values of production well water and surrounding groundwaters indicate higher levels of dissolved solids, greater than that of the stream water. The pH values of the production well (mean=7.5) and stream water (mean=7.4) are similar but quite different from that of the surrounding groundwater (mean=7.1). This also indicates the production well water may be influenced by the stream water (see Fig. 2).
3.2 Chemical Characteristics and Undergoing Processes
As shown in Table 3, concerning mean values for production wells, calcium (Ca2+) is the most prevailing cation (range=3.8-90.3 mg/L, mean=22.4 mg/L), followed by Na+, Mg2+, and K+ concentrations. The surrounding groundwater wells and stream water also show the same patterns. The anions showed the following order for production wells, HCO3-> SO42->Cl->NO3-; for surrounding groundwater wells, HCO3->NO3->Cl->SO42-; and for streams, HCO3-> Cl->NO3->SO42-. High levels of NO3- and Cl- in the groundwater wells and stream waters indicate possible contamination by agricultural activities and domestic wastewater (Vengosh and Pankratov, 1998; Lee et al., 2017; Maimoona and Lee, 2019; Cellone et al., 2020).
The Pearson correlation coefficients among the major chemical constituents are presented in Table 4. In the case of the production well waters, Ca2+ showed high positive correlations with Mg2+ (0.68), K+ (0.63), HCO3- (0.65), and SO42- (0.77). Because only two sites are located in the carbonated rock area, the overall water chemistry is considered to be less affected by calcite dissolution, while dolomite and gypsum dissolution may probably be based on the high correlations with Mg2+ and SO42- (Nazzal et al., 2014). However, for the surrounding groundwater, Ca2+ shows high correlations with Mg2+ (0.77) and HCO3- (0.62), which indicates that the groundwater may be affected by some other factors as well as the water-rock interactions. The stream water showed an interesting correlation pattern. Ca2+ is highly correlated with Mg2+ (0.91), HCO3- (0.75), SO42- (0.66) and Na+ is highly correlated with Cl- (0.75). The latter correlation between Na+ and Cl- was not found in the other two water bodies. Thus, we inferred that the stream water was largely influenced by domestic effluents.

Pearson correlation coefficients among chemical parameters for production wells, groundwater wells, and stream waters. (n=number of data). High values greater than 0.6 are in bold.
Fig. 3 shows Piper diagrams of major components of the studied water bodies. Most of these (85.8%) are of the Ca2+(+Mg2+)-HCO3- type, a typical type of fresh shallow groundwater (Lee et al., 2013; Fenta et al., 2020). Small portions of the Na+-HCO3- (8.9%), Ca2+-Cl- (2.9%), and Na+-Cl- (2.4%, only stream water) types were also found. Considering that the study sites are quite far from the seas, the impact of seawater intrusion is not considered; however, the probable influence of domestic wastewater, ion exchange between Ca2+ and Na+, and/or dissolution from silicate minerals (albite and microcline) may be plausible (Maimoona and Lee, 2019; Patel et al., 2020; Sunkari et al., 2020).

Piper diagram of major ion chemistry for production well, groundwater well, and stream water in the study area.
Fig. 4 shows the Gibbs diagrams of the production well water, surrounding groundwater, and stream water. For Na+ and Ca2+ ions, it is indicated that most of these (90.5%) were affected by rock weathering (water-rock interaction) while a small proportion (9.5%) was affected by precipitation (rainfall infiltration). In addition, regarding Cl- and HCO3- ions, the majority of the waters (91.1%) were influenced by the water-rock interaction, while 8.9% were influenced by the dilution due to rainfall. Considering the anthropogenic source of Na+ and Cl- from domestic effluents, it is reasonably inferred that the Gibbs diagram cannot appropriately explain the artificial origins of some constituents (Sajil Kumar, 2014; Zhang et al., 2018; Ryu et al., 2019).
3.3 Principal Components and Clustering
The exploratory PCA has yielded four principal components whose eigenvalue is greater than 1 (Fig. 5). These four principal components explain 65.88% of the total variance of the data sets (25.09, 15.12, 14.78, and 10.89%, respectively). Therefore, we assumed that these four components were appropriate for data interpretation without significant information loss. Table 5 shows the loadings of each component on water quality parameters. PC1 has moderate to high positive loadings on Ca2+ (0.62), Mg2+ (0.65), HCO3- (0.63), and SO42- (0.71). Therefore, this component is highly associated with water-rock interaction or weathering of silicate, carbonate, and/or sulfate minerals.

Eigenvalues of principal components and cumulative % variance explained by the components. Four principal components were retained with the criteria of eigenvalue greater than 1. The symbol cross indicates the eigenvalue and bar means the cumulative % variance.

Loadings of four principal components (PCs) retained with criteria of eigenvalue over 1. Values over 5.0 are in bold.
PC2 shows moderate loadings on Na+ (0.49), Cl- (0.66), and NO3- (0.69). Thus, it appears to be highly associated with anthropogenic contamination, such as the arrival of domestic wastewater and effects of agricultural activities in upstream areas (Vengosh and Pankratov, 1998; Lee et al., 2017; Maimoona and Lee, 2019; Cellone et al., 2020). Surely these components may be provided by rainfall but the EIA reports confirm the existence of houses and dry fields upstream of some bottled water facilities. PC3 shows a negative loading on Na+ (-0.56) with moderate positive loadings for KMnO4 consumption (0.68) and color (0.55). This component is related to organic contamination rather than the dissolution of silicate minerals (albite and microcline). PC4 has moderate positive loadings on color (0.44) and turbidity (0.67). Therefore, this component is closely associated with organic matter and fine particles suspended in water. High turbidity prevailed in the waters of carbonate rock areas.
From PCA, it is concluded that the water quality of the study areas is affected by weathering of silicate, carbonate, and sulfate minerals, and some anthropogenic pollution from domestic wastewater and agricultural activities.
Table 6 shows the mean water quality values of each cluster grouped by CA using k-means clustering. We predetermined three clusters (groups) based on the water chemistry and have examined the differences among them as well as how well they are classified by CA in terms of water chemistry. Contrary to our expectations, the clusters were not grouped according to the different water bodies. All water bodies are present in each cluster, indicating there are no significant differences in their water chemistry.

Mean values of parameters of each cluster grouped by the CA. The largest value for each parameter is in bold.
Of the 115 data sets, 8.7% are in Cluster 1, and most of these represent production well water in carbonate rock areas. Considering the high concentrations of Ca2+, Mg2+, HCO3-, and SO42-, this cluster indicates the geogenic enrichment from weathering of relevant minerals. According to high values of Na+, Cl-, NO3-, and turbidity, Cluster 2 (only 6.9%) is indicative of anthropogenic contamination with organic matter and agricultural fertilizers. Most of the water samples (84.3%) examined are classified into Cluster 3, which comprises many production well, surrounding groundwater, and stream water. This indicates that these water sources are not substantially different from each other in terms of their water chemistry. Furthermore, the water chemistry is the mid point between two end members, Clusters 1 and 2, representing geogenic and anthropogenic origins. Therefore, we inferred that the production wells are affected by anthropogenic contamination even though their water quality meets the Korean drinking water standards.
Fig. 6 shows some implications of anthropogenic effects on the water chemistry of production wells, surrounding groundwater wells, and stream water. Chloride (Cl-) and nitrate (NO3-) are representative constituents indicating the human impact on water chemistry. The cumulative probability plots and their inflection points help determine the water affected by anthropogenic pollution (Park et al., 2005). The inflection points of Cl- and NO3- are 15.84 and 16.21 mg/L, respectively. Using these values, we estimated 12.3% of waters examined were affected by the anthropogenic contamination with regard to Cl- and NO3-.
3.4 Contaminants of Concern
In addition to the water chemistry, we also examined contaminants of concern for production wells, as designated by the Korean Ministry of Environment (Fig. 7). The most frequently occurring contaminants are fluoride (F-), arsenic (As), nitrate (NO3-), and some heavy metals. These are mostly of natural origins, except for high levels of nitrate. In some areas, anthropogenic organic contamination, such as dichloromethane and toluene, was found even though their values did not exceed the Korean drinking water standards. However, this indicates a lack of maintenance of production wells and fuel storage tanks at bottled water manufacturing sites.
4. Conclusions
This study examined water chemistry and quality of production wells, surrounding groundwater wells, and stream water. Surprisingly, we found that they have similar characteristics in terms of water chemistry and quality. This indicates that the stream water migrates to the production wells, which is not a good sign for bottled water quality control. This migration between the stream water and production well water may be attributed to the installation of the production wells in the immediate vicinity of the streams (a few meters, within 20 meters). Therefore, stricter maintenance of nearby streams is required to secure high water quality standards of the production wells.
Arsenic is one of the most frequently occurring contaminants in the production wells. While they are all of natural origin, we require more intensive and sustained monitoring, as they are extremely toxic to human health (Goswami et al., 2019). Microplastics have also become an emerging contaminant in water environment, which could have potentially adverse effects on human health. As they have been found in bottled water in other countries (Kankanige and Babel, 2020; Makhdoumi et al., 2020) and bottled water consumption is increasing in Korea, the survey and monitoring of microplastics in groundwater has also become essential (Kim and Lee, 2020).
Acknowledgments
The authors would like to thank Mr. Sungbin Lee for his contribution in collecting the groundwater data from several Environmental Impact Survey reports. This research was supported by the Basic Science Research Program through the National Research Foundation of Korea (NRF) funded by the Ministry of Education (No.2019R1A6A1A03033167) and this work was supported by Korea Environment Industry & Technology Institute (KEITI) through Measurement and Risk assessment Program for Management of Microplastics Program (or Project), funded by Korea Ministry of Environment (MOE)(2020003110010).
References
-
Cellone, F., Carol, E., Pugliese, I., Cordoba, J., Butler, L. and Lamarche, L., 2020, Nitrate pollution in dairy farms and its impact on groundwater quality in a sector of the Pampas plain, Argentina. Environmental Earth Sciences, 79, 258.
[https://doi.org/10.1007/s12665-020-09005-3]
-
Fabbrocino, S., Rainieri, C., Paduano, P. and Ricciardi, A., 2019, Cluster analysis for groundwater classification in multi-aquifer systems based on a novel correlation index. Journal of Geochemical Exploration, 204, 90-111.
[https://doi.org/10.1016/j.gexplo.2019.05.006]
-
Fenta, M.C., Anteneh, Z.L., Szanyi, J. and Walker, D., 2020, Hydrogeological framework of the volcanic aquifers and groundwater quality in Dangila Town and the surrounding area, Northwest Ethiopia. Groundwater for Sustainable Development, 11, 100408.
[https://doi.org/10.1016/j.gsd.2020.100408]
- Freeze, R.A. and Cherry, J.A., 1979, Groundwater. Prentice-Hall, Inc., New Jersey, 604 p.
-
Goswami, R., Kumar, M., Biyani, N. and Shea, P.J., 2019, Arsenic exposure and perception of health risk due to groundwater contamination in Majuli (river island), Assam, India. Environmental Geochemistry and Health, 42, 443-460.
[https://doi.org/10.1007/s10653-019-00373-9]
- Hammer, ∅., Harper, D.A.T. and Ryan, P.D., 2001, PAST: Paleontological statistics package for education and data analysis. Paleontological Electronica, 4, 1-9.
-
Jolliffe, I.T. and Cadima, J., 2016, Principal component analysis: a review and recent development. Philosophical Transactions of the Royal Society A, 374, 20150202.
[https://doi.org/10.1098/rsta.2015.0202]
-
Kankanige, D. and Babel, S., 2020, Smaller-sized micro-plastics (MPs) contamination in single-use PET-bottled water in Thailand. Science of The Total Environment, 717, 137232.
[https://doi.org/10.1016/j.scitotenv.2020.137232]
- Kim, D.H., Jang, B.K., Hong, S.C., Moon, H.J., Lee, D.H. and Oh, H.J., 1993, Assessment of the Naktong River pollution after phenol spilling from the Kumi Industrial Estates II, Korea. Korean Journal of Preventive Medicine, 26, 268-281 (in Korean with English abstract).
-
Kim, H. and Lee, J.Y., 2020, Emerging concerns about microplastic pollution on groundwater in South Korea. Sustainability, 12, 5275.
[https://doi.org/10.3390/su12135275]
- Lee, J.Y., 2016, Step-drawdown test is not a tool to determine the so called Problems in determining optimal discharge using step-drawdown tests. Journal of the Geological Society of Korea, 46, 485-495 (in Korean with English abstract).
-
Lee, J.Y. and Hahn, J.S., 2006, Characterization of groundwater temperature obtained from the Korean national groundwater monitoring stations: Implications for heat pumps. Journal of Hydrology, 329, 514-526.
[https://doi.org/10.1016/j.jhydrol.2006.03.007]
-
Lee, J.Y., Kim, H.S., Yun, S.T. and Kwon, J.S., 2009, Factor and cluster analyses of water chemistry in and around a large rockfill dam: Implications for water leakage. Journal of Geotechnical and Geoenvironmental Engineering, 135, 1254-1263.
[https://doi.org/10.1061/(ASCE)GT.1943-5606.0000039]
-
Lee, J.Y. and Kwon, K.D., 2016, Current status of groundwater monitoring networks in Korea. Water, 8, 168.
[https://doi.org/10.3390/w8040168]
-
Lee, J.Y., Kwon, K.D., Park, Y.C. and Jeon, W.H., 2017, Unexpected nationwide nitrate declines in groundwater of Korea. Hydrological Processes, 31, 4693-4704.
[https://doi.org/10.1002/hyp.11389]
-
Lee, J.Y., Lee, K.S., Park, Y., Choi, H.M. and Jo, Y.J., 2013, Chemical and isotopic compositions of groundwater and stream water in a heavy agricultural basin of Korea. Journal of Geological Society of India, 82, 169-180.
[https://doi.org/10.1007/s12594-013-0135-2]
-
Lee, J.Y., Raza, M. and Park, Y.C., 2018, Current status and management for the sustainable groundwater resources in Korea. Episodes, 41, 179-191.
[https://doi.org/10.18814/epiiugs/2018/018015]
-
Lee, J.Y., Yi, M.J., Yoo, Y.K., Ahn, K.H., Kim, G.B. and Won, J.H., 2007, A review on the National Groundwater Monitoring Network in Korea. Hydrological Processes, 21, 907-919.
[https://doi.org/10.1002/hyp.6282]
-
Maimoona, R. and Lee, J.Y., 2019, Factors affecting spatial patter of groundwater hydrochemical variables and nitrate in agricultural region of Korea. Episodes, 42, 135-148.
[https://doi.org/10.18814/epiiugs/2019/019011]
-
Makhdoumi, P., Amin, A.A., Karimi, H., Pirsaheb, M., Kim, H. and Hossini, H., 2020, Occurrence of microplastic particles in the most popular Iranian bottled mineral water brands and an assessment of human exposure. Journal of Water Process Engineering, 101708.
[https://doi.org/10.1016/j.jwpe.2020.101708]
-
Nazzal, Y., Ahmed, I., Al-Arifi, N.S.N., Ghrefat, H., Zaidi, F.K., El-Waheidi, M.M., Batayneh, A. and Zumlot, T., 2014, A pragmatic approach to study the groundwater quality suitability for domestic and agricultural usage, Saq aquifer, northwest of Saudi Arabia. Environmental Monitoring and Assessment, 186, 4655-4667.
[https://doi.org/10.1007/s10661-014-3728-3]
-
Noshadi, M. and Ghafourian, A., 2016, Groundwater quality analysis using multivariate statistical techniques (case study: Fars province, Iran). Environmental Monitoring and Assessment, 188, 419.
[https://doi.org/10.1007/s10661-016-5412-2]
-
Park, S.C., Yun, S.T., Chae, G.T., Yoo, I.S., Shin, K.S., Heo, C.H. and Lee, S.K., 2005, Regional hydrochemical study on salinization of coastal aquifers, western coastal area of South Korea. Journal of Hydrology, 313, 182-194.
[https://doi.org/10.1016/j.jhydrol.2005.03.001]
-
Patel, M.P., Gami, B., Patel, A., Patel, P. and Patel, B., 2020, Climatic and anthropogenic impact on groundwater quality of agriculture dominated areas of southern and central Gujarat, India. Groundwater for Sustainable Development, 10, 100306.
[https://doi.org/10.1016/j.gsd.2019.100306]
-
Ryu, H.S., Lee, J.Y., Lim, C.W. and Kim, K., 2019, Hydrochemical characteristics of groundwater and stream water in a karst area of Samcheok, Korea. Journal of the Geological Society of Korea, 55, 117-129 (in Korean with English abstract).
[https://doi.org/10.14770/jgsk.2019.55.1.117]
-
Sajil Kumar, P.J., 2014, Evolution of groundwater chemistry in and around Vaniyambadi Industrial Area: Differentiating the natural and anthropogenic sources of contamination. Chemie der Erde, 74, 641-651.
[https://doi.org/10.1016/j.chemer.2014.02.002]
-
Sunkari, E.D., Abu, M., Zango, M.S. and Wani, A.M.L., 2020, Hydrogeochemical characterization and assessment of groundwater quality in the Kwahu-Bombouaka Group of the Voltaian Supergroup, Ghana. Journal of African Earth Sciences, 169, 103899.
[https://doi.org/10.1016/j.jafrearsci.2020.103899]
-
Vengosh, A. and Pankratov, I., 1998, Chloride/bromide and chloride/fluoride rations of domestic sewage effluents and associated contaminated ground water. Ground Water, 36, 815-824.
[https://doi.org/10.1111/j.1745-6584.1998.tb02200.x]
-
Zhang, Y., Xu, M., Li, X., Qi, J., Zhang, Q., Guo, J., Yu, L. and Zhao, R., 2018, Hydrochemical characteristics and mutivariate statistical analysis of natural water system: A case study in Kangding County, Southwestern China. Water, 10, 80.
[https://doi.org/10.3390/w10010080]