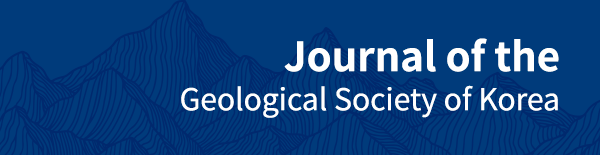
백령도 네오기 진촌 현무암에 포획된 첨정석 페리도타이트의 암석학적 특성
Copyright © 2018 The Geological Society of Korea
This is an Open Access article distributed under the terms of the Creative Commons Attribution Non-Commercial License (http://creativecommons.org/licenses/by-nc/3.0/) which permits unrestricted non-commercial use, distribution, and reproduction in any medium, provided the original work is properly cited.
초록
한반도 서북단 백령도에 분포하는 네오기 판내부 알칼리 현무암에는 첨정석 레졸라이트성분의 페리도타이트가 포획되어 있다. 이 첨정석 레졸라이트 포획암는 조립의 원생입상조직에서 비등립질, 조립질 결정들이 쌓여있는 큐물레이트 조직으로 산출되며, 이 조직들은 점이적으로 변하고 있다. 또한 어닐링을 나타내는 직선의 입자경계와 120°의 삼중점이 발달하고 있어 포획암들이 정적(±동력)재결정화작용을 겪었음을 나타낸다. 사방휘석 내에는 남겨진 감람석 결정들이 발달하고 있으며, 사방휘석이 감람석을 치환하는 조직도 관찰된다. 첨정석 레졸라이트 포획암의 구성광물인 감람석, 사방휘석, 단사휘석의 Mg#[=100×Mg/(Mg + Fetotal)]는 89-93, 첨정석의 Cr#[=100×Cr/(Cr+Al)]는 9-15의 범위를 보인다. 이들 포획암 구성광물들의 지화학적 성분은 입자의 크기나 조직적 차이와는 상관없이 거의 일정하며, 지화학적 평형이 이루어졌음을 반영한다. 계산된 평형온도와 산소분압은 각각 920-1,070℃ 와 △fO2(QFM)= -1.5~-0.5으로 나타난다. 첨정석 레졸라이트 포획암의 구성광물인 단사휘석은 대부분의 불호정성 원소에 대해 대체로 부화되어 있으며, 세 종류의 REE 패턴을 가진다: ① 경희토류(LREE)결핍[(La/Yb)N=0.3-0.4], (La/Ce)N=0.9-1.0], ② 경희토류 부화[(La/Yb)N=1.8-2.7, (La/Ce)N=1.6-1.7], ③ 경희토류 중에 La가 Ce 보다 더 높은 부화된 것(La/Yb)N=0.2-0.5, (La/Ce)N=1.0-1.1]이다. 중희토류 원소는 분별하지 않은 비교적 평평한 분포(10 × C1 chondrite)를 보인다. 이들 단사휘석은 고장력원소(Nb-Ta, Zr-Hf, Ti)와 저장력원소(Rb, Ba)에 결핍되어 있다. 이러한 조직적/지화학적 특성은 백령도 첨정석 레졸라이트 포획암은 부분용융에 의해 마그마를 배출하고 난 후 맨틀에 남아있었던 암석으로, 이후 실리카와 경희토류가 부화된 유체에 의해 모달/은폐 교대작용을 겪었음을 나타내고 있다.
Abstract
Peridotite xenoliths occurring in late Neocene intraplate alkaline basalt from Baegryeong Island, west-northern part of the Korean peninsula, are mainly anhydrous spinel lherzolites. The xenoliths display coarse grained protogranular through inequigranular to cumulate textures, grading into each other. They often show well-developed annealed textures and the triple junctions, suggesting that they went through static(±ynamic) recrystallization. They also contain left-over olivine grains within orthopyroxene, indicating replacement of olivine by orthopyroxene. The constituent minerals are compositionally homogeneous and appear to be equilibrated. The xenoliths are characterized by the high Mg#[=100×Mg/(Mg+Fetotal) atomic ratio] of olivine, orthopyroxene and clinopyroxene (89-93) and the Cr#[=100×Cr/(Cr+Al)] atomic ratio] of spinel (9-15). The calculated equilibrium temperatures and oxygen fugacities resulted in 920-1,070℃ and ΔfO2(QFM) = -1.5 ~ -0.5, respectively. Clinopyroxenes of the xenoliths are mostly enriched in incompatible trace elements, exhibiting three types of REE patterns: ① LREE-depleted [(La/Yb)N=0.3-0.4, (La/Ce)N=0.9-1.0], ② LREE-enriched [(La/Yb)N=1.8-2.7, (La/Ce)N=1.6-1.7], and ③ a enrichment in La over Ce [(La/Yb)N=0.2-0.5, (La/Ce)N=1.0-1.1]. They also show depletion in high field strength elements(e.g., Nb-Ta, Zr-Hf, Ti), and low field strength elements (e.g., Rb and Ba). We thus propose the Baegryeong peridotite xenoliths represent residues left after early melt extraction, which was subsequently subjected to different degrees of modal/cryptic metasomatism by silica- and LREE-enriched fluids (or melts).
Keywords:
Baegryeong Island spinel lherzolites xenoliths, Miocene alkaline basalt, recrystallization, metasomatism, silica- and LREE-enriched fluids (or melts)키워드:
백령도 첨정석 레졸라이트 포획암, 네오기 알칼리 현무암, 재결정화작용, 교대작용, 실리카와 경희토류 부화유체1. 서 론
현무암질 마그마에 포획되어 지표면에 노출된 페리도타이트 포획암은 그 크기는 비록 작지만 상부맨틀 암석권을 구성하고 있던 물질로서 상부맨틀 암석권에서 일어나는 부분용융, 교대작용과 같은 물질 순환에 대한 직접적이고 중요한 정보를 제공한다(Frey and Prinz, 1978; Kelemen et al., 1998; Xu et al., 2000; Downes et al., 2004; Perinelli et al., 2008; Grégoire et al., 2010; Woo et al., 2014; Ichiyama et al., 2016 and references therein). 맨틀암석을 포획하여 지표면을 향해 상승하는 마그마의 속도는 2-3 cm/sec로 포획된 맨틀암석이 상부맨틀에 있을 당시의 조직적/광물학적 특성이 거의 변형되지 않고 지표면까지 운반되어질 수 있는 상당히 빠른 속도로 해석되고 있다(Spera, 1980). 이러한 맨틀포획암의 특성은 상부맨틀 암석권에 대한 직접적인 지질학적 연구를 가능하게 해준다.
한반도가 위치하는 서태평양지역은 지구 내부의 물질이 지구 밖으로 배출되는 판내부 발산환경(즉, 백령도, 백두산, 제주도 등)과 지표면 물질이 지구 내부 속으로 들어가는 지판충돌 섭입대(동일본지진대)가 서로 가깝게 위치하고 있다(Sager et al., 1988; Chough et al., 2000; 그림 1a). 이러한 지구조적 과정과 관련되어 형성된 마이오세 배호분지(동해)가 함께 분포하고 있다(Otofuji et al., 1985; Kaneoka et al., 1992; Jolivet et al., 1994). 이 지역은 암석권의 생성과 소멸이 동시에 일어나는 곳으로 지구 물질 순환이 매우 활발한 지역임을 나타낸다. 그 결과 이곳의 암석권에는 다양한 사건을 경험한 암석이 분포하고 있을 것이며, 이를 반영하는 복잡한 암석조직과 성분이 포함되어 있을 것이다. 서태평양지역에 위치하는 한반도는 상부맨틀 암석권 연구에 최적의 실험실인 것이다.

(a) Google photo-map showing the geodynamic setting along the eastern margin of the Eurasian Plate. (b) Generalized geological map of Baegryeong Island showing the stratigraphic relationships of rocks and the sample locations of this study (Lim et al., 1999).
본 연구는 백령도 지역에 분포하는 후기 네오기 판내부 알칼리현무암에 포획된 페리도타이트 포획암을 대상으로 한다(그림 1b). 이 백령도 페리도타이트 포획암편들은 지구조적으로 확장축(동해)과 섭입대(일본 아크)가 인접해 있는 곳의 맨틀 구성물질로 네오기의 백령도 지역 대륙지각 하부의 상부맨틀 암석권의 모습을 나타내고 있다. 이 맨틀암석에 대한 암석학적 기재와 구성광물의 주성분, 미량원소 분석을 수행하여 조직적/지화학적 특성과 교대작용의 특성에 대해 보고한다. 이러한 특성은 섭입환경과 확장환경이 인접한 곳의 대륙지각 하부 상부맨틀 암석권에서 일어나는 물질순환에 대한 정보를 제공해 줄 것이다.
2. 일반지질
백령도는 유라시안판 내부의 동쪽 연변에 위치하며, 일본 아크에서 대륙쪽으로 약 1,200 km 가량 떨어져 있다(그림 1a). 유라시아판과 일본 아크 사이에 발달하고 있는 동해는 광범위한 배호분지의 확장에 의해 일본 아크가 유라시아판에서 분리되면서 초기-중기 마이오세에 형성되었다(Otofuji et al., 1985; Kaneoka et al., 1992; Jolivet et al., 1994). 일본 아크는 이자나기판이 유라시아판 아래로 섭입을 시작하였던 트라이아스기 이전부터 형성된 것으로 알려져 있다(Sager et al., 1988; Steinberger and Gaina, 2007). 동해분지에는 17-20 Ma (Ar-Ar 연대)에 분출한 현무암이 넓게 분포하고 있으며, 기반암은 배호분지 확장 동안 형성된 해양지각과 확장에 의해 지구조적으로 열려진(rift) 대륙지각으로 구성되어 있다(Kaneoka et al., 1992). 현재는 태평양판(해양판)이 쿠릴-캄챠카, 일본, 이주-보닌, 마리아나 해구를 따라 북서방향으로 유라시아판(대륙판)과 필리핀판(해양판) 아래로 섭입하고 있다. 유라시아판 아래로 섭입하고 있는 태평양판은 백두산 아래 약 660 km 깊이에서 유라시아판을 만나고 있다(Tang et al., 2014).
한반도 서북단에 위치하는 백령도는 중-신원생대 상원계의 백령층군과 이를 관입한 맥암류, 네오기의 진촌현무암과 충적층으로 이루어져있다(그림 1b). 백령층군은 변성퇴적암층으로 주로 점판암, 천매암, 규암 등으로 구성되어 있다. 첨정석 페리도타이트를 포획한 진촌현무암은 분포면적이 약 3 km2정도이며, 판내부 환경에서 4.2-7.1 Ma에 백령층군을 관입/분출하였다(Park and Park, 1996; Kim et al., 2004; Choi et al., 2006). 진촌현무암은 미세립의 감람석, 단사휘석과 자철석을 포함하고있는 알칼리 현무암이다(Park and Park, 1996).
3. 연구방법
본 연구는 백령도 북동쪽 진촌동 동측 해안가를 따라 분포하는 현무암에 포획된 첨정석 페리도타이트를 대상으로 한다(그림 2). 페리도타이트 포획암의 구성광물에 대한 암석학적, 조직적 특성과 성분조성을 파악하기 위해 박편과 연마편이 제작되었다. 제작된 박편과 연마편은 편광현미경(Nikon, Labophot2-pol)하에서 관찰하였으며 모드분석, 조직분류, 광물들 사이의 반응모습 등에 대한 기재를 수행하였다. 시료의 모달(modal) 조성은 전통적인 포인트 카운트 방법을 이용하여 편광현미경을 통해 분석하였으며, 각 시료마다 대략 1,500포인트를 카운트하였다. 본 연구에서는 Mecier and Nicolas (1975)의 정의를 따라 백령도 첨정석 페리도타이트 조직에 대한 분류와 조직적 특징을 기재하였다.

The outcrop overview of representative peridotite xenoliths showing a (a) protogranular and (b) equigranular texture appearance, respectively and (c) feldspar mega xenocrysts enclosed in the Jinchon basalt from Baegryeong Island.
광물(감람석, 사방휘석, 단사휘석, 첨정석)에 대한 주성분원소 분석은 부산대학교 공동실험실습관에 있는 CAMECA SX100 전자현미분석기를 통해 이루어졌다. 가속전압 20 kv, 샘플 전류 20 nA, 빔 직경 5 µm으로 분석 되었으며 각 원소의 분석시간은 10 초로 하였다. 분석을 위해 천연과 인조의 표준 샘플이 사용되었다. 정밀도는 ±1%, 정확도는 ±2%로 유지되었다. 하나의 결정 내에서 BSE 사진을 촬영과 광물의 중앙부와 가장자리에 대한 정량분석을 실시하였으나 화학적 누대는 관찰되지 않았으며 대체로 균질한 조성을 보였다. 조립질과 세립질 광물 사이에도 주성분 원소 차이가 없었다.
단사휘석에 대한 미량원소 분석은 한국기초과학지원연구원(충북 오창)에 있는 LA-MC-ICP-MS (Nu Plasma II/NWR193UC)로 수행되었다. LA-ICP-MS는 193 nm 파장의 레이저를 사용하며 레이저 분석조건은 빔 직경 85 µm, 분석에너지 5 J/cm2, ablation 시간은 60 s, 분석시간은 120 s, 반복률 10 Hz로 분석하였다. 외부표준물질은 NIST SRM 612가 사용되었으며 운반 가스로는 Ar-He 혼합물이 사용되었다. 단사휘석의 각 분석은 내부표준으로써 EPMA 분석에 의해 결정된 Si와 Mg함량에 표준화 되었으며 GLITTER! ver. 4.4.4 소프트웨어를 사용하여 광물의 미량원소 조성을 계산하였다.
4. 맨틀포획암
4.1 암석기재
백령도 진촌 현무암에는 직경 2-15 cm 크기의 첨정석 페리도타이트가 각상에서 아각상, 불규칙한 형 등 다양한 형태로 포획되어 있으며, 직경 3-20 cm의 거정질의 알칼리 장석도 포획되어 있다(그림 2). 이 첨정석 페리도타이트의 모달함량은 감람석 54-65%, 사방휘석 19-32%, 단사휘석 11-13%으로 레졸라이트(lherzolite)로 분류된다(표 1). 구성광물의 입자 크기와 엽리 등의 특징에 따라, 조립의 원생입상(protogranular) (그림 3a, 3b), 입자크기가 세립과 조립으로 이루어진 비등립질(inequigranular)(그림 3c, 3d), 그리고 특정한 방향성으로 길쭉길쭉한 광물들이 쌓여 배열된 큐뮬레이트(cumulate) 조직(그림 3e, 3f)으로 분류된다.

Modal composition, lithology, calculated pressure and equilibrium temperature conditions for the studied peridotite xenoliths from Baegryeong Island.

Photomicrographs of the spinel peridotite xenoliths from Baegryeong Island. All photos were taken under cross-polarized light. (a) A protogranular texture in the xenolith showing kink band in olivine and a olivine grain enclosed in orthopyroxene(16BR09). (b) A left-over olivine inclusion in a large orthopyxene (16BR09). (c) A transition from protogranular (the right upper section) to equigranular (the left lower section) (16BR03). (d) A left-over olivine inclusion in orthopyroxene (16BR03). (e) A cumulate texture showing elongated minerals with a preferred orientation (16BR08). (f) A left-over olivine inclusion in orthopyroxene (16BR08). ol=olivine; opx=orthopyroxene; cpx=clinopyroxene; sp=spinel.
원생입상조직 포획암은 결정입자의 크기는 직경 약 1 mm에서 10 mm까지 연속적으로 산출되지만 대체로 조립질 결정(>3 mm)이 우세하다(그림 3a). 구성광물들은 타형이며 입자 경계는 완만한 커브를 이루거나 불규칙한 곡선 혹은 직선으로 다양하다. 조립의 감람석에는 킹크밴드(kink band)가 발달하지만 세립의 네오블라스트(neoblast)는 이러한 변형흔적이 없다. 첨정석은 대체로 사방휘석, 감람석과 접촉하여 산출된다.
비등립질 조직의 포획암은 원생입상 조직에서 입자크기가 쌍봉을 이루는 반상쇄설(porphyroclastic)로 전이되는 중간단계의 조직으로 관찰된다(그림 3c). 조립질 입자는 직경 3 mm 보다 크고, 세립질 입자는 2 mm 이하로 산출되는 경향을 보인다. 입자들 사이의 경계는 더욱 직선화 되어지고 120°를 이루는 직선의 삼중점이 원생입상 조직보다 더욱 흔하게 발달하고 있어 어닐링(annealing) 특징을 보인다.
큐뮬레이트 조직은 결정들이 길쭉하게 신장되어 있으며 누적되어 쌓여있는 특성을 보인다(그림 3e). 특히 감람석의 경우 가로세로 비율(e.g., aspect ratio)이 1:8 정도로 신장되어 있으며, 첨정석도 길게 신장되어 있다. 직선의 입자경계와 120°의 삼중점이 원생입상과 비등립질 조직보다 더욱 흔하게 발달하고 있다. 첨정석은 불규칙한 모습으로 거의 대부분 다른 입자들 사이를 채우는 간극형으로 산출된다.
원생입상에서 비등립질, 큐물레이트 조직으로 가면서 입자경계가 더 직선화되고, 직선의 삼중점이 더 빈번하게 나타나고 있다. 레졸라이트 포획암의 연속적인 조직변화는 Mercier and Nicolas (1975)와 Harte et al. (1975)에서 설명되는 조립질에서 반상쇄설을 거쳐 등립질로 가는 연속적 모습의 중간단계를 잘 보여주고 있다. 이러한 조직을 통해 백령도 하부 상부맨틀 암석권에 다양한 조직의 페리도타이트가 점이적으로 변하면서 존재하고 있음을 반영한다.
백령도 페리도타이트 포획암에는 사방휘석 결정 내에 둥근 감람석 결정이 포유물로 산출되는 것이 특징적이다(그림 3a, 3b, 3d, 3f). 이는 사방휘석이 성장하여 입자경계이동(grain boundary migration, Passchier and Trouw, 1996)하여 생성된 것으로 감람석은 남겨진 결정(left-over)으로 해석된다. 남겨진 감람석 결정과 사방휘석에 의해 분리된 조립질의 감람석들은 서로 같은 간섭색을 보이거나 소광이 동시에 일어나는 광학적 연속성을 보이기도 한다(ol1 and ol2 in 그림 3b). 또한 사방휘석이 감람석의 가장자리 혹은 입자경계면을 따라 치환하는 조직도 관찰되며, 이런 경우 감람석 입자경계는 뚜렷하지 않고 지저분하게 보인다.
4.2 광물화학
백령도 첨정석 페리도타이트 포획암을 구성하는 감람석, 사방휘석, 단사휘석, 첨정석의 주성분 원소조성은 다음과 같다.
감람석은 Mg#[=100×Mg/(Mg+Fetotal)]=89-91, NiO =0.26-0.37 wt%, CaO=0.03-0.06 wt%의 조성을 보이며 NiO와 CaO는 비교적 낮은 값을 보인다(표 2). 조직에 따른 주성분 원소조성이 큰 차이를 보이지 않으며 비교적 균질한 값을 보여준다.
사방휘석은 enstatite Mg#=90-91, Al2O3=3.7-4.2 wt%, CaO=0.5-0.7 wt%, Cr2O3=0.3-0.4 wt%으로 좁은 조성적 범위를 보인다(표 3). 조직에 따른 주성분 원소조성이 큰 차이를 보이지 않으며 비교적 균질한 값을 보여준다. 사방휘석의 Al2O3와 CaO, Cr2O3, 그리고 감람석의 Mg#사이에서 상관관계를 형성하지 않는다(그림 4).

Olivine and orthopyroxene composition of the peridotite xenoliths from Baegryeong Island. (a, b) Relationships between Al2O3 versus CaO, and Cr2O3 for orthopyroxene. (c) Relationship between Mg# for olivine vs Al2O3 for orthopyroxene.
단사휘석은 투휘석(diopside)으로 Mg#=91-93, Al2O3 =5.5-6.4 wt%, TiO2=0.2-0.6 wt%, Na2O=1.5-1.8 wt%, Cr2O3=0.6-0.8 wt%, CaO=19.3-21.0 wt%의 조성을 가진다(표 4). Al2O3는 TiO2와는 양의 상관관계, Cr2O3와는 상관관계를 형성하지 않는다(그림 5a, 5b). Mg#와 Al2O3, Na2O와는 미약한 음의 상관관계를 보인다(그림 5c, 5d). 반면 공존하는 사방휘석의 Mg#와 단사휘석의 Mg#는 양의 상관관계를 보인다(그림 5e).

Clinopyroxene composition of the peridotite xenoliths from Baegryeong Island. (a, b) Al2O3 vs TiO2 and Cr2O3. (c, d) Relationship between Mg# vs Al2O3 and Na2O. (e) Relationship between clinopynoxene Mg# vs orthopyroxene Mg#.
첨정석은 Cr#[=100×Cr/(Cr+Al)]=9-15, Mg#=76-79, TiO2=0.04-0.12 wt%의 범위를 보인다(표 5). 이는 Carswell (1980)의 분류법에 의하면 알루미늄 첨정석에 해당한다. 첨정석의 Cr#와 감람석의 Mg# 사이에는 양의 상관관계를, 첨정석의 Mg#와 Cr#는 음의 상관관계를 보인다(그림 6a, 6b). 첨정석의 주성분 원소 조성은 심해 페리도타이트(abyssal peridotite) 성분 영역과 유사하다(그림 6).

(a) Relationships between Cr#[=100Cr/(Cr+Al) atomic ratio] for spinel and Fo[=100Mg/(Mg+Fe) atomic ratio] for olivine. (b) Relationships between the Mg# and Cr# of spinel. Olivine-spinel mantle array and melting trend (annotated by partial melting (%) are from Arai (1994). FFM, fertile MORB mantle; SSZ, supra-subduction zone.
함수광물이 없는 상부맨틀 암석권에서 단사휘석은 미량원소의 주요 운반자로 알려져 있다(Rampone et al., 1991). 백령도 하부 상부맨틀 암석권 미량원소의 지화학적 특성을 알기 위해 단사휘석에 대한 희토류원소(REE: Rare Earth Element) 분포도와 거미도(spider diagram)를 도시하였다(그림 7a, 7b). 미량원소의 표준화에는 McDonough and Sun (1995)의 C1 chondrite와 Primitive Mantle 조성이 사용되었다.

Clinopyroxene composition of the peridotite xenoliths from Baegryeong Island. (a) C1 chondrite-normalized (McDonough and Sun, 1995) REE patterns. (b) Primitive mantle-normalized (McDonough and Sun, 1995) multi-element spider diagram.
단사휘석의 희토류원소 함량은 C1 콘드라이트에 비해 부화되어 있다. 경희토류 원소의 패턴은 세 그룹으로 나눠진다(표 6; 그림 7a). ① 경희토류 원소 결핍 패턴: (La/Yb)N=0.3-0.4, (La/Ce)N=0.9-1.0을 나타낸다. ② 경희토류 원소에 부화 패턴: (La/Yb)N=1.8-2.7, (La/Ce)N=1.6-1.7을 나타낸다. ③ La 부화 패턴: (La/Yb)N =0.2-0.5, (La/Ce)N=1.0-1.1을 나타낸다. 경희토류 원소는 다양하게 부화되어 있지만 중희토류 원소는 분별하지 않은 비교적 평평한 분포(10 × C1 chondrite)를 보인다.
거미도표에서 대부분의 샘플은 저장력(LFS: low field strength)원소인 Ba, Pb와 고장력(HFS: high field strength)원소인 Ti, Ta-Nb, Hf-Zr는 음의 이상(anomaly)이 나타난다. 예외적으로 시료 “16BR08”의 경우 Hf-Zr이 양의 이상을 보인다(그림 7b). Th-U은 모든 시료에서 양의 이상을, Ti는 음의 이상을 보인다. 이러한 선택적인 원소의 부화와 결핍은 교대 대행자에 대한 성분적 정보 또는 확산능력에 대한 정보를 제공할 수 있다.
5. 토 의
백령도 북동쪽 진촌동 동측 해안가를 따라 분포하는 알칼리 현무암에 포획된 첨정석 페리도타이트의 조직과 구성광물인 감람석, 사방휘석, 단사휘석의 Mg#(89-93), 첨정석의 Cr#(9-15)은 세계 다른 곳의 상부맨틀 암석권의 조직적/지화학적 특성과 유사하다(Frey and Prinz, 1978; Downes et al., 2004; Kil and Wendlandt, 2004; Arai and Ishimaru, 2008; Woo et al., 2014). 본 연구에서는 백령도 맨틀포획암의 조직적/지화학적 그리고 교대작용의 특성에 대해 논의한다.
5.1 조직적/지화학적 특성(textural/geochemical characteristics)
백령도 진촌 현무암에 포획된 첨정석 페리도타이트의 조직은 연속적이고 점이적으로 변화하지만 원생입상에서 비등립질, 큐물레이트 조직으로 가면서 입자경계가 더 직선화되고, 직선의 삼중점이 더 빈번하게 나타나고 있다. 이는 포획암이 열에 의해 형성되는 어닐링의 결과이며, 정적재결정작용(static recrystallization)이 다양하게 이루어졌음을 나타낸다(Passchier and Trouw, 1996). 정적재결정화작용이란 암석의 변형이 멈춘 이후에 내부에너지의 분배에 의해 결정의 배치와 결정경계지역의 재결정화를 만드는 작용이다. 또한 페리도타이트 포획암에서 킹크밴드와 파동소광을 가지는 조립의 반상쇄정과 변형의 흔적이 없는 세립의 네오블라스트가 관찰된다. 이는 편압 하에서 비교적 균질한, 변형의 흔적이 없는 세립의 결정이 새로 형성되어지는 동력재결정화작용(dynamic recrystallization)의 결과임을 지시한다(Passchier and Trouw, 1996). 동력재결정화작용이란 재결정화작용의 종류중 하나이며 입자경계이동(grain boundary migration), 아결정입자 회전(subgrain rotation)과 같이 변형이 일어나는 동안 결정의 재결정화가 일어나는 것을 의미한다. 그러나 백령도 페리도타이트 포획암에서 관찰되는 재결정작용은 이전의 조직적 특성을 파괴할 만큼 충분히 강하지 않았으며, 연속적인 스펙트럼을 보이는 조직적 특성은 이 모든 암상이 하나의 구조영역에서 유래했음을 지시한다.
백령도 하부 상부맨틀 암석권에서 유래된 페리도타이트에서 관찰되는 이러한 국부적이고 연속적인 변형작용은 여러 가지 요인이 작용할 수 있지만 그중에서 현무암질 마그마의 상승이 추정된다. 어닐링은 맨틀포획암을 운반한 모마그마의 이동에 따른 열의 공급, 교대작용 대행자인 멜트/유체의 이동에 의해, 혹은 맨틀플룸으로부터 뜨거운 연약권 물질의 유입에 의해서 야기될 수도 있다(Mercier and Nicolas, 1975; Tracy, 1980; Vauchez and Garrido, 2001). 거의 대부분의 맨틀포획암은 열에 의해 야기되는 정적재결정작용과 어닐링을 경험하며, 이때 용융체나 유체가 관여하게 되면 정적재결정작용을 촉진하게 된다는 것은 널리 알려진 사실이다(Passchier and Trouw, 1996). 백령도 하부 상부맨틀 암석권은 모현무암 혹은 그 이전의 현무암이 분출하기 이전에 일어난 현무암질 마그마의 상승과 관련된 단층작용과 지진 사건(paleoseismic event)이 있었을 것이며 이에 의해 야기된 편압에서 정적/동력재결정작용이 다양하게 형성되었을 가능성을 제시한다.
백령도 첨정석 페리도타이트 포획암을 구성하고 있는 광물들의 주성분원소 조성은 대체로 겹치거나 분산된 경향을 보이지만 Mg 함량이 높고 현무암질 성분인 Al, Ti 함량은 낮아 분별용융을 경험한 맨틀잔류암석임을 나타내고 있다(그림 4-6). 주성분조성이 겹치거나 분산된 것은 페리도타이트가 다양한 교대작용을 경험했음을 반영한다. 왜냐하면 이 포획암이 부분용융만 겪었다면 이들의 주성분분원소는 어떤 경향성을 보이는 규칙적인 모습을 보여야한다. 백령도 첨정석 페리도타이트 포획암이 다소 분산된 모습을 보이고 있는 것은 교대작용의 결과로 해석된다. 페리도타이트 포획암 구성광물의 중앙부와 가장자리, 조립질과 세립질 광물의 주성분/미량원소 성분의 차이는 없다(표 2-6). 이는 상부맨틀 암석권에서 페리도타이트가 지화학적으로 평형상태에 도달했음을 나타내며, 온도가 높은 상부맨틀에서는 흔하게 관찰되는 모습이다(e.g., Falus et al., 2008; Woo et al., 2014).
첨정석의 Cr#와 감람석의 Fo 사이의 상관관계를 나타내는 OSMA (Olivine-Spinel Mantle Array) 다이아그램에서 백령도 페리도타이트 포획암은 10% 미만의 부분용융을 경험했다(그림 6a). 한편 단사휘석의 희토류원소 패턴을 이용한 부분용융의 정도는 가장 교대작용을 적게 받은 시료 “16BR03”이 약 10%를 보이고 있다(그림 8a). 단사휘석의 YbN과 YN에 대한 비율을 이용한 부분용융의 정도는 약 4%까지의 값을 보여주고 있다(그림 8b). 맨틀에서의 부분용 매케니즘에는 가장 end-member로서 배치용융(batch melting)과 분별용융(fractional melting)이 있다. 자연에서는 대체로 배치부분용융이 분별부분용융보다 더 그럼직한 것으로 여겨지는 것이 일반적이다(Wilson, 1989). 이러한 미량원소의 함량을 이용한 부분용융의 정도를 계산할 때에는 교대작용의 영향력을 고려해야한다. 단사휘석의 경희토류 패턴을 보았을 때, 교대작용의 영향이 있었음을 확인할 수 있고 중희토류 원소는 경희토류 원소에 비해 영향을 덜 받지만 교대작용의 영향을 같이 받음으로서 전체 희토류 원소 함량의 증가를 일으킬 수 있다. 왜냐하면 경희토류 원소에서 중희토류 원소로 갈수록 그 정도는 변하지만, 희토류원소는 모두 불호정성이기 때문이다. 따라서 희토류 원소의 패턴을 이용해 계산된 부분용융의 정도는 교대작용으로 인해 다소 작아진 값일 수 있음을 감안하여야 한다. 백령도 페리도타이트는 1-10%의 부분용융을 경험한 맨틀잔류암으로 비교적 퍼타일(fertile)한 특성을 보인다(그림 6a, 8). 백령도 페리도타이트 포획암의 주성분원소 조성은 심해 페리도타이트의 주성분 원소 조성과 유사한 특성을 보이는 반면에 섭입대 페리도타이트와는 다르게 나타난다(그림 6). 심해 페리도타이트는 해령에서 부분용융이 일어나고 난 후에 맨틀에 남아있는 잔류암석(residue)으로 알려져 있으며, 이와 대비되는 섭입대 페리도타이트는 심해 페리도타이트보다 좀더 refractory한 특성을 나타낸다.

Model calculations for clinopyroxene REE from the studied xenoliths (Ntaflos et al., 2008). (a) Chondrite-normalized REE abundance patterns (after McDonough and Sun, 1995) of clinopyroxenes and of predicted residual clinopyroxenes from bach melting model calculation(shaded area). Closed square and open square represent core and rim of the sample “16BR03”, respectively. (b) Predicted batch melting model trend with primitive mantle normalized Y and Yb (after McDonough and Sun 1995) of clinopyroxenes from the Baegryeong Island.
Brey and Köhler (1990)의 지온계인 Ca-in-Opx를 사용하여 계산된 평형온도는 910-920℃ 범위에 있다(표 1). Brey and Köhler (1990)의 지온계인 사방휘석-단사휘석의 주성분으로 계산된 평형온도는 920-1,070℃으로 시료 하나를 제외하면 920-980℃를 보인다. 이 온도범위는 제주도(910-1,000℃, 875-1,050℃; Choi et al., 2001; Woo et al., 2014)와 백두산(920-1,070℃, 750-1,010℃; Kim et al., 2017; Park et al., 2017)의 맨틀포획암에서 획득한 평형온도와 유사하다. 또한 캐나다, 미국서부의 Cascades, Patagonia와 같은 대륙아크의 페리도타이트 포획암의 평형온도(e.g., Dewey and Lamb, 1992; Ramos, 1999)와 유사하며, 대략 1,000℃ 정도를 보이는 심해 페리도타이트(Dygert and Liang, 2015)와 유사하다. 이 온도범위는 북동일본아크의 낮은 온도 그룹의 맨틀포획암(930-1,030℃)과 유사하며, 남서일본아크의 평형온도(1,100-1,250℃)보다는 낮은 온도이다(Arai and Ishimaru, 2008). 이때 압력은 1.5 GPa로 선택되었으며, 첨정석 페리도타이트 포획암에서 주로 사용되는 압력이다(Webb and Wood, 1986; McInnes et al., 2001). 백령도 맨틀포획암에 석류석 페리도타이트가 관찰되지 않고, 항상 첨정석 페리도타이트만이 관찰된다는 것은 이 포획암이 상대적으로 얕은 부분에 위치하고 있던 암석임을 반영한다(Wilson, 1989).
백령도 하부 암석권의 산화상태는 감람석-사방휘석-첨정석-산소 분압계(Ballhaus et al., 1991)를 사용하여 계산되었다. FMQ buffer에 비교하여 나타내는 ΔlogfO2(FMQ)(oxygen fugacity relative to the FMQ value) 값은 -1.5에서 -0.5 Δlog units의 범위를 보인다(표 1). 이 값은 FMQ buffer보다 환원적인 환경을 보이기는 하지만 거의 FMQ buffer에 접근하고 있음을 의미한다. 백두산 맨틀포획암의 ΔlogfO2(FMQ) 값(-0.8 to 0.2)과 제주도의 맨틀포획암에서 계산된 ΔlogfO2(FMQ)값(-1.1 to 0.4) 값보다는 낮은 편이다(Choi, 2000; Woo et al., 2014; Kim et al., 2017). logfO2(FMQ)값의 표준오차는 ±0.23 (log units)이다.
5.2 교대작용
백령도 첨정석 레졸라이트 포획암의 사방휘석 내부에는 감람석 결정이 남겨진 입자(left-over)로 산출되거나 감람석의 가장자리 혹은 입자경계면을 따라 사방휘석이 감람석과 반응한 조직도 관찰된다 (그림 3). 사방휘석내에 남겨진 감람석 결정은 사방휘석에 의해 분리된 큰 감람석결정과 간섭색이 같고 소광을 같이하는 광학적 연속성을 보인다. 이는 원래 하나의 감람석이었던 입자가 SiO2 성분이 포화된 유체나 멜트가 상부맨틀 암석권의 페리도타이트에 침투하며 감람석을 사방휘석으로 치환하면서 두 개의 감람석입자로 분리되어 진 것으로 해석된다(그림 3b, 3d, 3f). 이러한 모습은 백령도 아래 암석권이 다양한 모달 교대작용을 받아 사방휘석의 함량이 많아졌을 가능성도 제시한다(e.g., Kelemen et al., 1998).
주성분원소는 분별결정작용 혹은 분별부분용융에 대해 민감하게 반응하는 반면에 불호정성 미량원소는 교대작용에 대한 정보를 제공하는 것이 일반적인 이론이다(Wilson, 1989). 부분용융을 겪은 맨틀 암석은 경희토류 원소가 결핍된 암석이 되어야한다. 왜냐하면 암석의 중희토류 원소가 경희토류 원소에 비해 상대적으로 덜 불호정성이기 때문에 부분용융에 의해 마그마를 배출한 후의 잔류암석에는 경희토류 원소가 결핍되어진다. 그러나 백령도 첨정석 레졸라이트 포획암은 경희토류 원소가 다양하게 부화되어 있다(그림 7a). 세 종류의 경희토류 원소 패턴이 제시되어진다. ① 경희토류 원소 결핍 패턴(시료 17BR06)으로 이 시료는 교대작용을 받기 전 부분용융에 의해 만들어진 희토류 원소 패턴을 간직하고 있는 암석이다. ② 경희토류 원소에 부화된 패턴(시료 16BR08)으로 이는 경희토류 원소에 부화된 유체가 침입하였음을 증거하는 패턴이다. ③ 경희토류 원소 중에 La가 Ce에 비해 더 부화된 패턴(시료 16BR03)으로 경희토류 원소 중에 특히 La에 더욱 부화된 유체가 교대작용을 일으켰음을 나타낸다. 즉, 어떤 암석은 교대작용을 거의 받지 않았거나, 어떤 암석은 “경희토류 원소”를 선택적으로 더 많이 교대/부화시킨 모습으로 나타난다(그림 7a). 그 결과 경희토류 원소는 다양한 모습으로, 중희토류 원소는 평평한 모습을 지니게 되었다. 이러한 미량원소의 다양한 패턴은 백령도 아래 암석권이 다양한 은폐(cryptic) 교대작용을 받았음을 나타내고 있다. 이들 암석들 사이에 일어난 교대작용의 정도가 다양하고 서로 다르게 나타나는 것은 페리도타이트와 반응한 교대대행자인 유체나 멜트의 양이 달랐거나, 암석과 교대대행자와의 거리가 서로 달랐기 때문으로 해석되는 것이 일반적이다. 이러한 해석은 파푸아 뉴기니아와 Spitsbergen의 맨틀포획암에서 이미 제시되어져 있다(Grégoire et al., 2001, 2010).
판내부 환경에서 만들어진 백령도 현무암내에 실리카와 경희토류 원소에 부화된 맨틀포획암이 있다는 것은 일반적인 것은 아니다. 왜냐하면, 페리도타이트에 실리카와 경희토류 원소가 유입되는 환경은 일반적으로 섭입대 위에 놓인 맨틀웻지 환경이며, 이런 실리카와 경희토류 원소에 부화된 유체나 멜트는 섭입하는 슬랩(slab)에서 유래한다는 것이 일반적인 모델이기 때문이다(Kelemen et al., 1998; Smith et al., 1999; Manning, 2004; Arai and Ishimaru, 2008 and references therein). 또한 앞선 토의에서 백령도 첨정석 레졸라이트 포획암은 섭입대 페리도타이트보다는 심해 페리도타이트에 유사한 특성을 보여주었고 백령도의 조구조 환경은 현재 맨틀웻지 환경과는 물리적인 거리가 있기 때문이다. 그러나 연구된 맨틀포획암은 Ba, Ti, 고장력원소인 Nb-Ta와 Zr-Hf이 결핍되어 있고, 경희토류 원소와 U에 부화되어 있으며, 중희토류 원소는 분별하지 않는 평평한 모습을 보인다(그림 7). 이러한 특성은 슬랩 기원의 유체/멜트에 의해 교대작용 받은 맨틀암석에 공통적으로 나타난다(McCulloch and Gamble, 1991; Ionov and Hofmann, 1995; Keppler, 1996).
슬랩 기원의 함수멜트/유체는 지표면을 향해 상승하면서 함수광물인 각섬석과 금운모를 정출하고 Ti, Nb, Ta, Rb, Ba원소에 결핍되어진다. 이 잔류 규산염 멜트/유체가 백령도 맨틀암석을 교대작용 시켰으며, 그 결과 현재의 단사휘석에 나타나는 미량원소의 모습이라는 것이 본 연구의 해석이다. 이 경우 금운모의 Rb와 Ba의 상대적인 분배계수에 의해 교대작용 대행자인 잔류 멜트나 유체는 Rb이 Ba보다 더 결핍되어진다. 금운모의 Rb에 대한 분배계수는 Ba 보다 크기 때문에 금운모를 정출하고 난 후의 잔류 규산염 멜트는 Rb가 Ba보다 훨씬 더 결핍되어진다. 이러한 특성을 지닌 교대작용 대행자가 Ba이 더 결핍된 모습의 단사휘석(그림 7b)을 형성할 수 있는지에 대해 의문을 제기하게 된다. Ba에 비해 더욱 더 Rb에 결핍된 규산염 멜트일지라도 Ba에 비해 Rb에 부화된 현재의 단사휘석의 미량원소 특성을 만들 수 있다. 왜냐하면 단사휘석과 규산염멜트와 사이의 Rb과 Ba 분배계수는 각각 0.07과 0.0007이다(Neumann et al., 2002). 즉, 단사휘석에 Rb이 Ba에 비해 100배 이상 부화되어질 수 있기 때문이다. Choi et al. (2005)은 카보나타이트 멜트와 단사휘석의 미량원소 패턴이 유사한 것에서 백령도 페리도타이트의 변성교대작용의 대행자는 카보나타이트 멜트로 제시하고 있다. 이 경우 카보나타이트 멜트와 단사휘석 사이의 Rb과 Ba 분배계수는 각각 0.006과 0.07이다(Neumann et al., 2002). 즉, 카보나타이트 멜트도 현재의 단사휘석의 패턴(그림 7b)을 형성시킬 수 있다. 교대작용 대행자가 규산염멜트이냐 카보내타이트냐에 따라 분배계수는 상당하게 달라지기 때문에 지화학적 정보와 함께 암석기재/조직에 대한 정보가 매우 중요하다.
이곳에서 각섬석이나 금운모를 만들고 난후 분별되어진 규산염 잔류유체/멜트에는 이러한 성분(즉, Ti, Nb, Ta, Ba)이 결핍되어진다. 이 잔류유체/멜트는 주변의 맨틀 페리도타이트에 스며들게 되고 그곳의 암석을 다양하게 부화시킨다. 백령도 하부 상부맨틀 암석권은 현재 지구조적으로 판내부환경이지만 이곳을 부화시킨 교대작용은 섭입대 관련 슬랩기원의 잔류유체(혹은 마그마)가 중요한 역할을 담당했을 수도 있다. 현재 섭입환경과 전혀 상관없어 보이는 미국의 Bearpaw산의 화산암(50-54 Ma)에 포획된 맨틀포획암의 교대작용도 백악기 섭입대 관련 교대작용으로 해석되고 있다(Downes et al., 2004). 백령도 하부 상부맨틀 암석권의 다양한 정도의 부분용융과 부화 작용이 국부적인 조직적/지화학적 불균질성을 야기하는 것으로 해석된다.
상부맨틀 암석권에서 부화(enrichment)작용을 야기 시키는 교대작용 대행자의 특성은 크게 3 종류로 (1) 규산염 멜트(e.g., Ionov et al., 2002), (2) 탄산염 혹은 카보내타이트 멜트/유체(e.g., Xu et al., 2003), (3) 함수 멜트/유체(e.g., Downes, 2001)로 구분된다. 점성이 낮은 휘발성 성분(H2O-CO2)이 풍부한 유체는 페리도타이트에 침투하면서 모암과 강하게 반응하면서 지속적으로 페리도타이트를 교대작용시킨다는 것이 최근에 제시되는 모델이기도 하다(e.g., Downes, 2001; Orejana and Villaseca, 2008). 앞으로 함수유체의 가능성도 확인되어야할 문제이다. 또한 이러한 변성교대작용이 하나의 성인을 가진 대행자에 의한 것인지 아니면 성인적으로 관련이 없는 여러 개의 교대작용 대행자가 관여했는지를 밝히는 것도 매우 복잡한 앞으로의 과제이다.
6. 결 론
- 1) 네오기 진촌 알칼리 현무암에 포획된 백령도 첨정석 레졸라이트는 조립질의 원생입상, 비등립질, 큐물레이트 조직이 연속적인 스펙트럼을 형성하며, 사방휘석이 감람석 포유물 입자를 산출하고 있는 것이 흔하게 관찰된다.
- 2) 이 페리도타이트 포획암은 정적/동력 재결정화작용을 다양하게 경험했다.
- 3) 백령도 첨정석 레졸라이트는 지화학적으로 완전히 평형상태에 도달하였으며, 계산된 평형온도는 비교적 높은 920-1,070℃, 산화/환원 상태는 ΔlogfO2(FMQ) = -1.5 ~ -0.5로서 거의 FMQ buffer에 접근하고 있다.
- 4) 백령도 첨정석 레졸라이트는 적어도 두 단계의 지화학적 진화과정을 기록하고 있다. 일차적으로 1-10%의 분별부분용융에 의해 결핍되었고 그 이후 실리카와 미량원소(특히 경희토류 원소)에 부화된 유체에 의해 다양한 모달/은폐 교대작용을 경험하였다.
- 5) 백령도 첨정석 레졸라이트는 지화학적으로 심해 페리도타이트와 유사한 특성을 보이지만 슬랩 기원의 잔류유체(혹은 멜트)가 이 포획암의 모달/은폐 교대작용의 대행자로서 중요한 역할을 담당했다.
Acknowledgments
세심한 심사를 통해 본 논문의 수준을 높여주신 익명의 심사위원님들께 깊은 감사를 드립니다. 이 논문은 2015년도 정부(교육부)의 재원으로 한국 연구재단의 지원을 받아 수행된 연구(NRF-2015R1D1A1A01056955)입니다.
References
-
Arai, S., (1994), Characterization of spinel peridotites by olivine-spinel compositional relationships: Review and interpretation, Chemical Geology, 113, p191-204.
[https://doi.org/10.1016/0009-2541(94)90066-3]
- Arai, S., and Ishimaru, S., (2008), Insights into petrological characteristics of the lithosphere of mantle wedge beneath arcs through peridotite xenoliths: a review, Journal of Petrology, 49, p665-695.
-
Ballhaus, C., Berry, R.F., and Green, D.H., (1991), High pressure experimental calibration of the olivine-orthopyroxene-spinel oxygen geobarometer: implications for the oxidation state of the upper mantle, Contributions to Mineralogy and Petrology, 107, p27-40.
[https://doi.org/10.1007/bf00311183]
- Brey, G.P., and Köhler, T.P., (1990), Calcium exchange between olivine and clinopyroxene calibrated as a geobarometer for natural peridotites from 2 to 60 kb with applications, Geochimica et Cosmochimica Acta, 54, p2375-2388.
-
Carswell, S.A., (1980), Mantle derived lherzolite nodules associated with kimberlites, carbonatite and basalt magmatism: a review, Lithos, 13, p121-138.
[https://doi.org/10.1016/0024-4937(80)90013-4]
-
Choi, S.H., (2000), Oxidation state of mantle xenoliths from Jeju-do, South Korea, Geosciences Journal, 4, p211-220.
[https://doi.org/10.1007/bf02910139]
- Choi, S.H., Jwa, Y.J., and Lee, H.Y., (2001), Geothermal gradient of the upper mantle beneath Jeju Island, Korea: Evidence from mantle xenoliths, Island arc, 10, p175-193.
-
Choi, S.H., Kwon, S.T., Mukasa, S.B., and Sagong, H., (2005), Sr-Nd-Pb isotope and trace element systematics of mantle xenoliths from Late Cenozoic alkaline lavas, South Korea, Chemical Geology, 221, p40-64.
[https://doi.org/10.1016/j.chemgeo.2005.04.008]
-
Choi, S.H., Mukasa, S.B., Kwon, S.T., and Andronikov, A.V., (2006), Sr, Nd, Pb and Hf isotopic compositions of late Cenozoic alkali basalts in South Korea: Evidence for mixing between the two dominant asthenospheric mantle domains beneath East Asia, Chemical Geology, 232, p134-151.
[https://doi.org/10.1016/j.chemgeo.2006.02.014]
-
Chough, S.K., Kwon, S.T., Ree, J.H., and Choi, D.K., (2000), Tectonic and sedimentary evolution of the Korean peninsula: a review and new view, Earth Science Reviews, 52, p175-235.
[https://doi.org/10.1016/s0012-8252(00)00029-5]
-
Dewey, J.F., and Lamb, S.H., (1992), Active tectonics of the Andes, Tectonophysics, 205, p79-95.
[https://doi.org/10.1016/0040-1951(92)90419-7]
-
Downes, H., (2001), Formation and modification of the shallow sub-continental lithospheric mantle: a review of geochemical evidence from ultramafic xenolith suites and tectonically emplaced ultramafic massifs of western and central Europe, Journal of Petrology, 42, p233-250.
[https://doi.org/10.1093/petrology/42.1.233]
-
Downes, H., Macdonald, R., Upton, B.G.J., Cox, K.G., Bodinier, J.-L., Mason, P.R.D., James, D., Hill, P.G., and Hearn, B.C. Jr, (2004), Ultramafic xenoliths from the Bearpaw Mountains, Montana, USA: evidence for multiple metasomatic events in the lithospheric mantle beneath the Wyoming craton, Journal of Petrology, 45, p1631-1662.
[https://doi.org/10.1093/petrology/egh027]
-
Dygert, N., and Liang, Y., (2015), Temperatures and cooling rates recorded in REE in coexisting pyroxenes in ophiolitic and abyssal peridotites, Earth and Planetary Science Letters, 420, p151-161.
[https://doi.org/10.1016/j.epsl.2015.02.042]
-
Falus, G., Tommasi, A., Ingrin, J., and Szabo, C., (2008), Deformation and seismic anisotropy of the lithospheric mantle in the southeastern Carpathians inferred from the study of mantle xenoliths, Earth and Planetary Science Letters, 272, p50-64.
[https://doi.org/10.1016/j.epsl.2008.04.035]
-
Frey, F.A., and Prinz, M., (1978), Ultramafic inclusions from San Carlos, Arizona: petrologic and geochemical data bearing on their petrogenesis, Earth and Planetary Science Letters, 38, p129-176.
[https://doi.org/10.1016/0012-821x(78)90130-9]
- Grégoire, M., McInnes, B.I.A., and O'Reilly, S.Y., (2001), Hydrous metasomatism of oceanic sub-arc mantle, Lihir, Papua New Guinea Part 2. Trace element characteristics of slab-derived fluids, Lithos, 59, p91-108.
- Grégoire, M., Chevet, J., and Maaloe, S., (2010), Composite xenoliths from Spitsbergen: evidence of the circulation of MORB-related melts within the upper mantle, Geological Society, London, Special Publications, 337, p71-86.
-
Harte, B., Cox, K.G., and Gurney, J.J., (1975), Petrography and geological history of upper mantle xenoliths from the Matsoku kimberlite pipe, in Ahrens, L.H., Dawson, J.B., Duncan, A.R., and Erlank, A.J. eds., physics and chemistry of the Earth, 9, Oxford, Pergamon, p477-506.
[https://doi.org/10.1016/0079-1946(75)90035-x]
-
Ichiyama, Y., Morishita, T., Tamura, A., and Arai, S., (2016), Peridotite xenoliths from the Shiribeshi Seamount, Japan Sea: insights into mantle processes in a back-arc basin, Contributions to Mineralogy and Petrology, 171, p86.
[https://doi.org/10.1007/s00410-016-1300-6]
-
Ionov, D.A., and Hofmann, A.W., (1995), Nb, Ta-rich mantle amphiboles and micas: Implications for subduction-related metasomatic trace element fractionations, Earth and Planetary Science Letters, 131, p341-356.
[https://doi.org/10.1016/0012-821x(95)00037-d]
- Ionov, D.A., Bodinier, J.L., Mukasa, S.B., and Zanetti, A., (2002), Mechanisms and sources of mantle metasomatism: major and trace element conditions of peridotite xenoliths from Spitzbergen in the context of numerical modelling, Journal of Petrology, 43, p2261-2278.
-
Jolivet, L., Tamaki, K., and Fournier, M., (1994), Japan Sea, opening history and mechanism: a synthesis, Journal of Geophysics Resourch, 99, p22237-22259.
[https://doi.org/10.1029/93jb03463]
- Kaneoka, I., Takigami, Y., Takaoka, N., Yamashita, S., and Tamaki, K., (1992), 40Ar-39Ar analysis of volcanic rocks recovered from the Japan Sea floor: constraints on the age of formation of the Japan, In: Tamaki, K., Suyehiro, K., Allan, J., McWilliams, S. (eds), Proceedings of the Ocean Drilling Program Scientific Result, 127/128, College Station, Texas, (Ocean Drilling Program) p819-836.
-
Kelemen, P.B., Hart, S.R., and Bernstein, S., (1998), Silica enrichment in the continental upper mantle via melt/rock reaction, Earth and Planetary Science Letters, 164, p387-406.
[https://doi.org/10.1016/s0012-821x(98)00233-7]
-
Keppler, H., (1996), Constraints from partitioning experiments on the composition of subduction-zone fluids, Nature, 380, p237-240.
[https://doi.org/10.1038/380237a0]
-
Kil, Y., and Wendlandt, R.F., (2004), Pressure and temperature evolution of upper mantle under the Rio Grande Rift, Contributions to Mineralogy and Petrology, 148, p265-280.
[https://doi.org/10.1007/s00410-004-0608-9]
- Kim, E., Park, G., Kim, S., Kil, Y., and Yang, K., (2017), Petrology and Geochemistry of peridotite xenoliths from Miocene alkaline basalt near the Mt. Baekdu area, Journal of Petrological Society of Korea, (in press).
- Kim, K.H., Nagao, K., Tanaka, T., Sumino, H., Nakamura, T., Okuno, M., Ishitsuka, Y., Jin, B.L., and Song, J.H., (2004), He-Ar and Nd-Sr isotopic compositions of ultramafic xenoliths and host alkali basalts from the Korean peninsula, Annual meeting of the Korea Society of Economic and Enviromental Geology (Abstracts), 125.
- Lim, S.B., Choi, H.I., Kim, B.C., and Koh, H.J., (1999), Depositional System and Geological Structures of the Protrozoic Paegryeong Group, Journal of the Geological Society of Korea, 54, p11, (in Korean with English abstract).
- Manning, C.E., (2004), Chemistry of subduction-zone fluids, Earth and Planetary Science Letters, 223, p1-16.
-
McCulloch, M.T., and Gamble, J.A., (1991), Geochemical and geodynamical constraints on subduction zone magmatism, Earth and Planetary Science Letters, 102, p358-374.
[https://doi.org/10.1016/0012-821x(91)90029-h]
- McDonough, W.F., and Sun, S.S., (1995), The composition of the Earth, Chemical Geology, 120, p223-253.
-
McInnes, B.I.A., Grégoire, M., Binns, R.A., Herzig, P.M., and Hannington, M.D., (2001), Hydrous metasomatism of oceanic sub-arc mantle, Lihir, Papua New Guinea: Petrology and geochemistry of fluid-metasomatised mantle wedge xenoliths, Earth and Planetary Science Letters, 188, p169-183.
[https://doi.org/10.1016/s0012-821x(01)00306-5]
-
Mercier, J.C.C., and Nicolas, A., (1975), Textures and fabrics of upper-mantle peridotites as illustrated by xenoliths from basalts, Journal of Petrology, 16, p454-487.
[https://doi.org/10.1093/petrology/16.1.454]
-
Neumann, E.R., Wulff pedersen, E., Pearson, N.J., and Spencer, E.A., (2002), Mantle xenoliths from Tenerife (Canary Islands): Evidence for Reactions between Mantle Peridotites and Silicic Carbonatite Melts inducing Ca Metasomatism, Journal of Petrology, 43, p825-857.
[https://doi.org/10.1093/petrology/43.5.825]
-
Ntaflos, T., Tschegg, C., Coltorti, M., Akinin, V.V., and Kosler, J., (2008), Asthenospheric signature in fertile spinel lherzolites from the Viliga Volcanic Field in NE Russia, Geological Society, London, Special Publications, 293, p57-81.
[https://doi.org/10.1144/sp293.4]
-
Orejana, D., and Villaseca, C., (2008), Heterogeneous metasomatism in cumulate xenoliths from the Spanish Central System: implications for percolative fractional crystallization of lamprophyric melts, Geological Society, London, Special publications, 293, p101-120.
[https://doi.org/10.1144/sp293.6]
- Otofuji, Y., Mastuda, T., and Nohda, S., (1985), Paleomagnetic evidence for the Miocene counter-clockwise rotation of Northeast Japanç rifting process of the Japan Sea, Earth and Planetary Science Letters, 72, p265-277.
- Park, J.B., and Park, K.H., (1996), Petrology and petrogenesis of the Cenozoic alkali volcanic rocks in the middle part of Korean Peninsula (I): petrography, mineral chemistry and whole rock major element chemistry, Journal of the Geological Society of Korea, 32, p223-249, (in Korean with English abstract).
- Park, K., Choi, S.H., Cho, M., and Lee, D.C., (2017), Evolution of the lithospheric mantle beneath Mt. Baekdu (changbaishan): Constraints from geochemical and Sr-Nd-Hf isotopic studies on peridotite xenoliths in trachybasalt, Lithos, 286-287, p330-344.
-
Passchier, C.W., and Trouw, R.A.J., (1996), Microtectonics, Springer-Verlag, Berlin, p289.
[https://doi.org/10.1007/978-3-662-08734-3]
-
Perinelli, C., Sapienza, G.T., Armienti, P., and Morten, L., (2008), Metasomatism of the upper mantle beneath the Hyblean Plateau (Sicily): evidence from pyroxenes and glass in peridotite xenoliths, In: Coltorti, M., Gregoire, M. (Eds.), Metasomatism in oceanic and continental lithospheric mantle: Geological Society of London Special Publication, 293, p197-221.
[https://doi.org/10.1144/sp293.10]
- Ramos, V.A., (1999), Plate tectonic setting of the Andean Cordillera, Epiodes, 22, p183-190.
-
Rampone, E., Bottazzi, P., and Ottolini, L., (1991), Complementary Ti and Zr anomalies in orthopyroxene and clinopyroxene from mantle peridotites, Nature, 354, p518-520.
[https://doi.org/10.1038/354518a0]
- Sager, W.W., Handschumacher, D.W., Hilde, T.W.C., and Bracey, D.R., (1988), Tectonic evolution of the northern Pacific plate and Pacific-Farallon-Izanagi triple junction in the late Jurassic and early Cretaceous, Tectonophysics, 155, p345-364.
- Smith, D., Riter, J.C.A., and Mertzman, S.A., (1999), Erratum to ‘‘water-rock interactions, orthopyroxene growth and Si-enrichment in the mantle: evidence in xenoliths from the Colorado Plateau, southwestern United States’’, Earth and Planetary Science Letters, 167, p347-356.
- Spera, F.J., (1980), Aspects of magma transport, In: HARGRAVES, R.B. (ed.), Physics of Magmatic Processes, Princeton University Press, Princeton, NJ, p265-324.
-
Steinberger, B., and Gaina, C., (2007), Plate-tectonic reconstructions predict part of the Hawaiian hotspot track to be preserved in the Bering Sea, Geology, 35, p407-410.
[https://doi.org/10.1130/g23383a.1]
-
Tang, Y., Obayashi, M., Niu, F., Grand, S.P., Chen, Y.J., Kawakatsu, H., Tanaka, S., Ning, J., and Ni, J.F., (2014), Changbaishan volcanism in northeast China linked to subduction-induced mantle upwelling, Nature Geoscience, 7, p470-475.
[https://doi.org/10.1038/ngeo2166]
- Tracy, R.J., (1980), Petrology and genetic significance of an ultramafic xenolith suite from Tahiti, Earth and Planetary Science Letters, 48, 1, p80-96.
-
Vauchez, A., and Garrido, C.J., (2001), Seismic properties of an asthenospherized lithospheric mantle: constraints from lattice preferred orientations in peridotite from the Ronda massif, Earth and Planetary Science Letters, 192, p235-249.
[https://doi.org/10.1016/s0012-821x(01)00448-4]
-
Webb, S.A.C., and Wood, B.J., (1986), Spinel-pyroxene-garnet relationships and their dependence on Cr/Al ratio, Contributions to Mineralogy and Petrology, 92, p471-480.
[https://doi.org/10.1007/bf00374429]
-
Wilson, M., (1989), Igneous petrogenesis a global tectonic approach, Springer Science & Business Media, p466.
[https://doi.org/10.1180/minmag.1989.053.372.15]
- Woo, Y.H., Yang, K.H., Kil, Y., Yun, S., and Arai, S., (2014), Silica- and LREE-enriched spinel peridotite xenoliths from the Quaternary intraplate alkali basalt, Jeju Island, South Korea: Old subarc fragments?, Lithos, 208, p312-323.
-
Xu, X., O, 'Reilly, S.Y., Griffin, W.L., and Zhou, X., (2000), Genesis of young lithospheric mantle in southeastern China: an LAM-ICPMS trace element study, Journal of Petrology, 41, p111-148.
[https://doi.org/10.1093/petrology/41.1.111]
-
Xu, Y.G., Menzies, M.A., Thirlwall, M.F., Huang, X.L., Liu, Y., and Chen, X.M., (2003), "Reactive" harzburgites from Huinan, NE China: products of the lithosphere- asthenosphere interaction during lithospheric thinning?, Geochimica et Cosmochimica Acta, 67, p487-505.
[https://doi.org/10.1016/s0016-7037(02)01089-x]