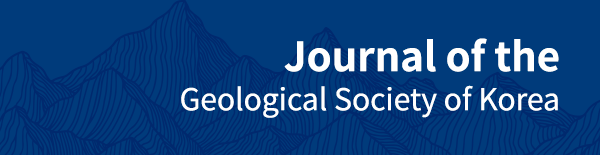
소량의 시료의 X-선 회절분석을 통한 단층 주미끌림대의 물질분석
1경상대학교 지질과학과 및 기초과학연구소2경상대학교 공동실험실습관
2Center for Research Facilities, Gyeongsang National University, Jinju 52828, Republic of Korea
Copyright © 2017 The Geological Society of Korea
This is an Open Access article distributed under the terms of the Creative Commons Attribution Non-Commercial License (http://creativecommons.org/licenses/by-nc/3.0/ which permits unrestricted non-commercial use, distribution, and reproduction in any medium, provided the original work is properly cited.
초록
전단변위가 집중되는 단층핵 내부의 주미끌림대(principal slip zone, PSZ)를 구성하는 물질, 즉 단층물질(fault material)은 단층의 마찰 특성을 결정하는 가장 중요한 인자 중 하나이다. 따라서, 단층물질의 광물조성을 정확히 확인하는 것은 단층의 역학적 거동 이해를 위해 반드시 필요하다. 그러나 자연의 단층대에서 관찰되는 PSZ는 대부분 수 cm에서 수 mm 이하의 매우 좁은 폭을 가지기 때문에 PSZ를 이루는 단층물질만의 정확한 광물조성을 알기 위해서는 시료채취와 분석과정에서 특별한 주의가 필요하다. 본 연구에서는 좁은 PSZ에서 채취된 미량의 시료만으로도 단층물질의 광물조성이 쉽고 정확하게 결정될 수 있는지를 확인하기 위해 고분해능 XRD 기기를 이용한 X-선 회절분석을 시도하였다. 본 연구의 대상물질은 포항 보경사 지역의 양산단층대에서 발달하는 2 cm 폭의 PSZ와 그 주변 단층핵에서 채취되었으며, 이 물질로부터 일차적으로 슬랩을 만들고 X-선 회절분석 시료는 마이크로 드릴을 이용하여 그 슬랩으로부터 소량의 파우더를 긁어내는 방식으로 채취하였다. 시료량에 따른 고분해능 XRD기기의 정확도를 검증한 결과, 정성분석이 가능한 최소시료량은 대략 2 mg으로 측정되었다. 이것을 기준으로 PSZ 내부 단층물질들(PSZ-B, PSZ-R)과 단층핵 내부 갈색 단층물질(BZ)을 미량(2 mg) 채취하여 분석한 결과, PSZ가 인접한 물질들과는 상이한 광물조성을 가질 수 있음을 확인하였다. 본 연구를 통해 개발된 PSZ 단층물질 분석법은 기존 덩어리시료 분석법에 비해 좁은 영역에서 발달하는 단층물질 연구에 효과적이며, 전단실험으로부터 회수한 실험단층물질이나 시추코어에서 발견되는 단층물질 등 이용 가능한 시료량이 극히 제한된 상황에서의 물질연구에도 활용될 수 있을 것이다.
Abstract
Fault materials in principal slip zones (PSZs), where the shear displacement is concentrated, are one of the most important factors that control the frictional properties of a fault. Thus, the identification of mineral composition of fault materials is essential to understanding the mechanical behaviors of faults. However, since the PSZ of natural faults is commonly very thin (less than a few cm or mm), special attention should be paid to the sampling and analysis of the materials in the PSZ. In this study, we designed X-ray diffraction (XRD) analyses on the fault materials taken from a narrow PSZ using a high-resolution X-ray diffractometer to examine if mineral composition can be reliably determined from a small amount of fault materials. The materials analyzed in the study were taken from about 2-cm-thick PSZ and adjacent fault core of the Yangsan fault zone, Bogyeongsa area, Pohang. Some slabs were prepared from the materials, and the powder specimens used in the XRD analyses were scraped from the slabs with a micro-drill. Given the results of the XRD analyses performed by changing the amount of the material, it follows that the minimum amount of sample needed for the reliable determination of mineral composition is estimated to be about 2 mg. The mineral compositions determined by using the 2-mg-specimens of the PSZ materials (PSZ-B and PSZ-R) and the brownish fault core material (BZ) confirm that the PSZ may be composed of different minerals from the adjacent areas. The method developed in the study may be effectively used for the materials not only in thin layers of natural fault materials but also in recovered experimental faults or in thin faults found in drilling cores from which only a small amount of material can be collected.
Keywords:
X-ray diffraction, faults, fault materials, principal slip zones, Yangsan fault zone키워드:
X-선회절, 단층, 단층물질, 주미끌림대, 양산단층대1. 서 론
단층의 주미끌림대(principal slip zone, PSZ)에는 마찰미끌림 시의 다양한 물리 화학적 과정에 의해 만들어진 물질과 더불어 그것이 시간에 따라 변화되어 만들어진 산물 등이 함께 존재하는데, 이를 통틀어 단층물질(fault materials)이라 정의할 수 있다. 이 물질은 눈으로 볼 수 있는 입자에서부터 나노입자까지 크기가 다양하며(e.g., Han et al., 2007a, 2007b; Viti, 2011; Siman-Tov et al., 2013) 때때로 비정질 물질을 포함하기도 한다(e.g., Yund et al., 1990; Lin, 1994; Kirkpatrick et al., 2013).
다양한 단층물질에 대한 지금까지의 전단시험 결과로부터 확인된 중요한 사실은 단층의 주미끌림대의 구성물질이 단층의 역학적 거동에 결정적인 영향을 준다는 점이다(e.g., Carpenter et al., 2009, 2011; Ikari et al., 2011; Fujimoto and Sato, 2017). 가령, 천부에서 흔히 관찰되는 점토질 단층비지(clay-rich fault gouge)를 이루는 광물들 중 스멕타이트(smectite)는 낮은 마찰계수값(실온, 실내습도, σn = 50MPa 조건에서 μ < 0.3)을 보이며, 카올리나이트(kaolinite)는 상대적으로 큰 마찰계수값(실온, 실내습도, σn = 50MPa 조건에서 μ < 0.65)을 나타내는 경향을 보인다(e.g., Ikari et al., 2011). 또한, 단층면에서 강한 분쇄작용이나 열분해작용 등으로 생성되는 결정질 또는 비정질 나노입자가 미끌림대에 존재하는 경우 강한 동적단층약화(dynamic fault weakening)현상이 발생하여 더 큰 변위의 단층미끌림을 유발할 가능성이 높다는 최근의 연구결과들(e.g., Di Toro et al., 2004; Janssen et al., 2010; Han et al., 2011; Hayward et al., 2016; Hirono et al., 2016; Aretusini et al., 2017) 역시 보고되었다. 이런 이유로 단층핵(fault core), 그 중에서도 전단변위가 집중되는 PSZ를 이루는 단층물질의 구성성분을 정확히 알아내는 것이 단층의 역학적 거동연구 측면에서 매우 중요하다고 볼 수 있다.
단층핵 내에 발달하는 PSZ는 주변 단층물질과는 다른 색상, 광물 및 화학조성, 미구조 등을 가질 수 있는 것으로 알려져 있다(e.g., Chester and Cherster, 1998; Ishikawa et al., 2008; Oohashi et al., 2011; Kim et al., 2016). 이러한 PSZ의 폭은 좁게는 수 m, 넓게는 수 백 m 폭으로 발달하는 단층대(fault zone)의 폭에 비해 극도로 얇아서, 대부분 수 cm에서 1 mm 이하의 좁은 영역으로 발달한다(e.g., Chester and Cherster, 1998; Faulkner et al., 2003; Sibson, 2003; De Paola et al., 2008; Smith et al., 2011). 따라서, PSZ의 구성물질을 식별하기 위해서는 그 영역에 존재하는 물질만을 채취하여 분석해야 하나, 기존에는 시료 채취의 어려움과 분석에 필요한 물질의 양적인 제한 등으로 인해 이런 식의 분석이 시도되지 못해왔다.
최근 분석기기들의 기술적 발달로 고분해능 기기들이 보편화 되면서 이러한 기기들을 활용한 지질학적 연구들이 매우 활발하게 진행되고 있다(e.g., Wirth, 2009). 특히, 자연단층과 전단실험으로부터 생산된 단층물질에 대한 나노스케일에서의 광물학 및 지화학적 연구에서도 이러한 기기들이 큰 기여를 하고 있다(Bestmann et al., 2011; De Paola et al., 2011; Janssen et al., 2013; Kirkpatrick et al., 2013; Collettini et al., 2014; Han et al., 2014; Kuo et al., 2016; Viola et al., 2016; Lee et al., 2017; Scuderi et al., 2017). 그러나 기기의 분해능이 높아질수록 까다로운 시료준비 과정과 그 과정에서 발생될 수 있는 시료의 오염, 운용자의 분석숙련도, 높은 분석비용 등의 단점들이 존재한다. 이러한 측면에서 X-선 회절분석(X-ray diffraction, XRD)은 간편한 시료준비과정 및 운용, 상대적으로 짧은 분석시간, 그리고 저렴한 분석비용으로 물질의 광물학적 특징을 파악할 수 있다는 장점들을 가진 장비임에 틀림없지만 상대적으로 많은 량(> 2 g)의 시료가 필요하며, 덩어리(bulk) 샘플링 및 분석으로 인해 미소구간에 대한 분해능이 상대적으로 떨어지는 단점 또한 존재한다. 그렇기 때문에 지금까지의 단층물질에 대한 XRD 분석에서는 PSZ를 포함한 보다 넓은 영역에서 물질이 채취 및 분석되어 분해능 있는 물질분석이 이루어지지 못했던 측면이 있다(e.g., Choo and Chang, 2000). 그러나 최근 XRD 기기 역시 다수의 채널을 가지는 고분해능 검출기를 이용하여 극소량의(수 mg) 시료만으로도 신뢰도 높은 분석이 가능한 수준으로 발전하였기 때문에 XRD의 분석 한계를 넘어서 단점을 극복하는 수준에 이르렀다.
본 연구의 목표는 XRD 분석 시 통용되고 있는 덩어리 샘플링 및 이를 이용한 분석법의 단점을 극복하기 위해 고분해능 XRD를 활용하여 분석결과의 신뢰성이 확인되는 최저 시료량을 확인하고, 나아가 좁은 폭의 PSZ로부터 소량의 물질을 직접 채취하여 분석한 결과를 덩어리 시료로부터 최저 시료량만을 취하여 분석한 결과와 비교함으로써 시료량이 극도로 제한된 단층물질에 대해 적용될 수 있는 XRD 분석법을 제시하고자 한다.
2. 포항 보경사 지역의 양산단층대에서 관찰되는 단층핵
포항 보경사 인근의 북북서-남남동 방향으로 발달하는 하천에는 한반도 동남부의 주요 주향이동단층들 중의 하나인 양산단층대의 전체 내부구조가 연속적으로 잘 드러나 있다(e.g., Chae and Chang, 1994; 그림 1a). 연구지역의 단층대는 백악기 경상분지 하양층군에 속하는 퇴적암층과 유천층군에 속하는 화산암(데사이트질 라필리 응회암)의 경계부에서 약 220 m의 폭으로 발달하고 있으며, 내부에는 약 10 m 폭의 단층 핵대(fault core zone)가 발달한다(Kim et al., 2016; 그림 1b). 단층핵대를 구성하는 단층비지대(fault gouge zones)들 중의 하나인 BZ (brown gouge zone)와 서편의 단층손상대(데사이트질 라필리 응회암 측)와의 경계부(그림 1c)를 자세히 관찰한 결과, N10°E/80°NW 자세의 직선에 가까운 경계부를 따라 기존 연구에서 보고되지 않은 치밀한 조직의 암갈색 물질이 최대 2 cm의 폭으로 관찰되었다(그림 1d).

(a) Satellite image of the study area (http://map.naver.com) showing the trace of the Yangsan fault and the study area (star). (b) Internal structure of the Yangsan fault, Bogyeongsa area (modified from Kim et al., 2016). BPZ, blue and purple gouges. BZ, brown gouge. PSZ, principal slip zone. (c) Outcrop photograph of the boundary between the dacitic lapilli tuff and brown gouge zone (BZ). (d) Dark brown layer (white arrows) developed along the boundary between the damage zone and fault core.
2.1 단층암 시료의 슬랩 및 박편제작
단층핵(BZ)과 단층손상대의 경계에서 관찰된 암갈색 물질의 미구조와 광물조성을 확인하기 위해 BZ와 데사이트질 라필리 응회암의 경계부를 중심으로 약 20 cm × 30 cm 크기의 정향된 덩어리(bulk)시료를 채취하였으며, 미고결 상태의 시료의 파손을 방지하기 위해 저점도 에폭시(Struers 사의 Epofix-set)를 이용하여 외부를 보강한 후 슬랩형태의 관찰면을 연마하였다. 연마된 관찰면의 고해상도 이미지를 얻기 위해 고해상도 스캔작업을 실시하였으며(그림 2a), 이미지 상에서 확인된 미끌림면을 포함하는 부분을 칩(chip) 형태로 잘라 박편을 제작하였다. 모든 슬랩 및 박편제작과정에는 수분에 취약한 시료의 특성 상 연마제와 금속가공용 방전가공유를 섞은 현탁액을 이용하여 관찰면을 연마하였다. 또한, 모든 관찰면은 단층의 미끌림선(slickenline)에 평행하고 단층면에 수직한 면을 기준으로 제작되었다.

Slab and microstructural images of the fault materials in the principal slip zone (PSZ) and brown gouge zone (BZ). (a) Polished rock slab image showing the PSZ and BZ. The zones are composed of the fault materials of different colors and structures. FC, fault core materials; PSZ-R, reddish materials in PSZ; PSZ-B, bluish materials in PSZ. White boxes are the sampling area for the XRD analyses. Optical photomicrographs (cross polarized light; gypsum plate inserted) and BSE (backscattered electron) images showing the microstructures of PSZ-R (b), PSZ-B (c), and BZ (d).
2.2 단층암의 미구조적 특성
BZ와 단층손상대의 경계부에서 채취한 슬랩의 내부에는 야외에서 관찰한 바와 같이 최대 2 cm 폭의 암갈색 물질이 대상으로 경계부를 따라 거의 직선형태로 관찰된다. 암갈색 물질의 내부에는 눈에 보이는 크기의 암편들이 결핍된 매우 치밀한 조직을 가지며, 광학관찰결과 내부 입자들의 강한 배열이 관찰되었기 때문에 본 영역을 미끌림이 집중되어 극도의 파쇄현상을 경험한 PSZ로 판단하였다(그림 2a). PSZ 내부에는 암갈색 물질뿐만 아니라 청색(그림 2a의 PSZ-B 영역) 및 암적색(그림 2a의 PSZ-R 영역)의 물질들이 약 1 mm 내외의 폭을 가지는 얇은 띠 형태로 교호한다. 암적색의 PSZ-R 물질은 광학현미경 하에서 수 십 μm 크기의 암편들이 전체적으로 분포하고 있으며, 기질부는 암편보다 더 작은 크기의 점토 및 불투명광물(opaque minerals)들로 이루어진다. 전자현미경 관찰결과, 기질부는 초미립의 판상의 점토 광물뿐만 아니라 최대 2 μm에서 마이크론(micron) 이하 크기의 입상(granular)조직을 가지는 물질들이 다량 관찰된다(그림 2b). 청색의 PSZ-B 물질은 광학 현미경 하에서 PSZ-R에 비해 암편을 적게 포함하고 있으며, 기질부는 강한 배열을 가지는 점토광물들로 구성된다. 그러나 전자현미경 관찰결과, PSZ-R 물질과 유사한 마이크론 이하 크기의 입상조직의 물질들과 초미립의 점토광물들이 관찰되었다(그림 2c). 슬랩에서 관찰되는 BZ 물질은 최대 1 cm 크기의 방해석, 퇴적암, 화산암 등으로 이루어진 암편들과 주전단방향에 사교하는 방향의 엽리들을 이루는 갈색의 점토광물로 이루어져 있으며, 전자현미경 관찰결과, 주로 미립의 판상의 점토광물들이 크고 작은 암편들 사이를 채우고 있다(그림 2d).
3. 실험방법
3.1 고분해능 XRD
소량 채취된 점토질 단층물질의 상(phase)분석을 위해 경상대학교 공동실험실습관의 고해상도 X-선 회절분석기기(Bruker사의 D8 Advance A25)를 이용하였다(그림 3a). 본 기기의 측정대원(measuring circle)의 반지름은 560 mm이며, 1.5418 Å의 입사각을 가지는 Cu Kα1 특성 X-선을 사용 한다. X-선 회절분석기는 기본적으로 X-선 발생장치(generator), 고니오미터(goniometer), 검출기(detector)로 구성되어 있다. X-선 발생장치는 최대 3 kW의 출력으로 가속전압은 20 ~ 60 kV, 가속전류는 2 ~ 60 mA로 설정이 가능하며 Cu 타겟의 X-선 튜브(최대 2.2 kW)가 장착되어 있다. 고니오미터는 수직형의 theta/theta 구동방식이며, 측정대원의 반지름은 560 mm, 최대 사용각도는 -60 ~ +158°, 정확도는 0.005°로 이전 장비에 비해 회절피크의 분해능이 현저히 향상되었다. 검출기는 다중채널 복합 실리콘검출기(multi-channel compound silicon strip detector; Bruker사의 LYNXEYE XE)를 사용하였으며 극소량의 시료로부터 양질의 회절 X-선을 얻는 주요 부품이다. 본 검출기는 냉각장치없이 실온에서 작동이 가능한 에너지 분산형 다중채널 방식이다(표 1). 680 eV의 탁월한 에너지 분해능으로 니켈 필터 없이도 고해상도(high resolution)모드에서 백색 및 Kβ-선을 제거할 수 있고, 192개의 채널을 사용하여 짧은 시간에 양질의 회절선을 얻을 수 있어 극소량의 시료를 측정하는데 매우 용이하다. 소량시료 측정 시, 소량의 시료를 흩뿌려 로딩할 수 있는 실리콘홀더(low-background silicon sample holder)를 사용하였다. 이 홀더는 실리콘 단결정의 911면으로 제작되어 비정질 피크나 배경 피크가 검출되지 않아 소량시료를 측정하기 적합하다.

(a) The high-resolution XRD diffractometer used in the study. (b) For the XRD analysis, a small amount of the powder sample is collected from the slab surface using a micro-drill. (c) Photograph showing the suspension (powder and distilled water) from which clay minerals in the fault materials are collected for the XRD analysis. (d) The powder sample (not less than 2 mg) is placed on the low-background Si holder which has not a cavity.
3.2 시료준비
PSZ 내부에서 얇게 발달하는 PSZ-R와 PSZ-B 영역, BZ 물질에서 눈에 보이는 크기의 암편을 제외한 영역, 그리고 기존의 덩어리시료 채취방법을 고려한 FC 영역(PSZ와 BZ 물질을 모두를 포함하는 단층핵 물질; fault core materials) 등 각각의 영역(그림 2a의 흰색 사각형)에 대한 분말샘플링을 실시하였다. 비교적 많은 량의 시료를 얻을 수 있는 BZ와 FC 영역은 야외에서 직접 채취 후 실내에서 약 24시간 동안 40℃의 건조오븐에서 건조시켰으며, 각각 5 g 이상의 시료를 10분 동안 마이크로나이징 밀(micronizing mill; McCrone microscopes & accessories)을 사용하여 <5 μm 크기의 입자로 분쇄시켰다. BZ 및 FC 영역과는 달리 수 mm 폭으로 산출되는 PSZ 물질들은 완성된 슬랩에서 정확한 위치를 파악 후 마이크로 드릴(micro-drill; Proxxon사의 FBS 240/E)로 슬랩의 표면을 긁어 분말시료를 채취하였다(그림 3b). 이후 소형 아게이트에 무수에탄올과 채취된 분말을 섞어 10분간 <5 μm 크기의 입자로 분쇄시켰다.
3.3 분석방법
기기가 분석 가능한 최소시료량을 알기 위해 동일한 물질(FC)을 대상으로 시료량을 각각 1,500 mg, 150 mg, 2 mg, 1 mg, 0.5 mg으로 나누어 실험을 실시하였다. 그 이후 검증된 최소시료량을 기준으로 FC 물질에 대한 점토광물 정방형시료분석과 슬랩으로부터 채취된 소량의 PSZ 물질 및 단층핵 물질에 대한 XRD 정성분석을 실시하였다. 점토광물의 정방형시료를 위해 10 g의 건조시료와 100 ml의 증류수를 혼합하여 10분간 초음파 세척하고, 30분 동안 마그네틱으로 교반하여 약 1시간 침전시킨 후 상부 2 cm의 혼탁액을 채취하여 7,000 rpm에서 15분간 원심분리하였다. 분리된 점토입자들은 슬라이드글라스 위에 도포하여 상온에서 건조하였다. 소량의 점토광물 정방형시료는 마이크로 튜브(micro-tube)에 약 2 mg의 시료와 1.5 ml의 증류수를 혼합하여(그림 3c) 10분간 초음파 세척 후 10분간 교반기(vortex)를 이용하여 시료 내의 점토광물을 분리 하였고, 이후 30분간 침전과정을 거쳐 혼탁액의 상부 0.5 ml를 슬라이드글라스위에 도포한 후 상온에서 건조하였다. 정방형시료를 제외한 모든 분석은 40 kv/40 mA, 4~70° 2-theta 구간에서 주사간격 0.02°, 주사시간은 시료량에 따라 각각 0.2초, 0.5초, 1초로 설정하였으며(표 2), 모든 시료는 0.4 mm 발산슬릿(divergent slit)을 이용하였다. 점토광물 정방형시료의 분석조건은 4~15° 2-theta 구간에서 주사간격 0.01°, 주사시간 1초로 설정하였으며 나머지 조건은 정성분석을 위한 조건과 같다. 시료의 홀더는 시료의 량에 따라 1,500 mg은 저각홀더(low-angle holder), 150 mg은 홈이 있는 실리콘 홀더(low-background silicon holder with cavity), 2 mg 이하는 모두 평평한 실리콘홀더(low-background silicon holder without cavity)를 사용하였다(표 2; 그림 3d).
4. 결 과
4.1 고분해능 XRD의 최소 시료량 검증
시료량에 따른 회절 X-선의 변화를 알아보기 위해 PSZ 물질과 BZ 물질을 모두 포함하는 FC 시료를 대상으로 1,500 mg (FC-N), 150 mg (FC-150), 2 mg (FC-2), 1 mg (FC-1), 0.5 mg (FC-0.5)의 각기 다른 양을 투입하여 XRD 정성분석을 실시하였다(그림 4a). 그 결과, FC는 스멕타이트 그룹, 녹니석, 일라이트, 일라이트/스멕타이트 혼합층광물 등의 다양한 점토 광물들과 석영, 장석, 방해석 등의 광물로 이루어져 있으며, 상대적으로 소량의 적철석 또한 포함되어 있다. 시료량에 따른 회절패턴의 변화를 비교해 보면, FC-N과 FC-150은 거의 유사한 회절패턴을 보였으며, FC-2의 경우 전체적으로 피크(peak)의 크기가 다소 감소하였지만 FC-N 및 FC-150과 매우 유사한 회절패턴으로 정성분석이 가능한 정도의 해상도를 보여준다. 반면, FC-1은 FC-2에 비해 상대적으로 저각에서의 배경값(background)과 전체 진폭이 다소 상승하는 결과를 보이며, FC-0.5의 회절패턴은 몇몇의 피크가 사라지며 정성분석이 불가능 할 정도의 해상도를 보여준다. 그렇기 때문에 본 기기의 신뢰도가 보장된 최소 시료량을 약 2 mg으로 설정하였다. 또한 10 g (FC-N)과 2 mg (FC-2)의 FC 물질에서 각각 분리된 점토광물의 정방향시료 XRD 분석결과(그림 4b), FC-N에 비해 FC-2의 저각에서의 배경값이 다소 높고, 점토광물이 아닌 다른 광물들의 피크가 미미하게 감지되었지만 스멕타이트 그룹 및 녹니석으로 구성된 점토광물들의 정성적인 판별은 가능한 수준의 결과를 보였다.
4.2 PSZ 물질과 단층핵의 XRD 결과 비교
앞서 검증된 XRD 분석이 가능한 최소시료량인 2 mg을 기준으로 PSZ 내부의 PSZ-B 및 PSZ-R 물질, 그리고 BZ 물질을 대상으로 XRD 정성분석을 실시하였고, 그 결과를 PSZ 및 BZ 물질을 모두 포함하는 FC-2의 회절패턴과 비교해 보았다(그림 5). BZ 물질은 스멕타이트 그룹과 일라이트/스멕타이트 혼합층 광물, 녹니석, 그리고 소량의 일라이트로 구성된 점토 광물들이 주를 이루며, 석영과 방해석 등의 광물들도 포함되어 있는데, 장석의 피크가 매우 낮게 관찰되는 것이 특징적이다. 이 결과는 BZ 물질을 덩어리 채취해서 1,500 mg의 시료량으로 분석을 실시한 Kim et al. (2016)의 XRD 결과와 매우 유사하다. 반면, PSZ-R과 PSZ-B 물질은 BZ에 비해 저각에서의 배경값이 높고 점토광물들의 피크가 낮으며 석영 및 장석의 피크가 높은 것을 확인 할 수 있다. 그리고 FC-2 결과에서는 BZ와 PSZ-R 및 PSZ-B를 구성하고 있는 대부분의 광물들이 모두 인지되었다.
5. 토의 및 결론
실험결과에 따르면, 고분해능 XRD를 이용하여 약 2 mg의 미량의 시료만을 사용하더라도 신뢰도가 높은 XRD 정성분석결과를 도출 해 낼 수 있었으며(그림 4), 이를 통해 양산단층의 단층핵 내부에 존재하는 여러 가지 단층물질들의 광물상(mineral phase)을 비교적 손쉽게 알아낼 수 있었다. 특히 단층의 미끌림이 집중된 PSZ 영역을 이루고 있는 물질의 XRD 결과는 점토광물의 함량이 BZ에 비해 적고 입상의 물질들이 다수 포함되어 있는 미구조적 특징을 잘 반영하는 결과이며, 미립의 입상의 물질들은 대부분 석영 내지 장석으로 이루어진 암편일 가능성이 높다(그림 5). 이 결과는 단층의 미끌림에 주도적으로 관여한 물질과 단층핵 전체를 이루는 물질의 조성이 다를 수 있다는 사실을 직접적으로 보여주는 좋은 예로서, 본 분석법이 단층핵 내부에서 수 cm 이하의 좁은 영역에서 발달하는 주 미끌림대 구성물질을 식별하는데 효율적임을 지시한다. 그러므로 단층의 미끌림과 직접 관계된 좁은 PSZ 물질만을 채취하여 XRD 분석을 시도할 경우 본 분석법을 고려해야 할 필요가 있을 것으로 판단된다. 그러나 몇 가지 개선되어야 할 사항들이 확인되었다. 미량 XRD 분석시에 사용된 홈이 없는 실리콘홀더의 특성상 도포된 시료의 두께로 인해 전체 피크가 다소 이동(displacement)한 것을 관찰할 수 있었는데(그림 5), 이러한 경우 잘 알려진 광물들의 단독 피크를 기준으로 보정이 필요하다. 또한, 시료의 절대량이 적기 때문에 XRD 정량분석이 가능한 만큼의 신호가 충분하지 못한다는 문제점도 존재한다. 게다가 미량의 점토광물의 정방향시료 분석결과에서 점토광물이 아닌 다른 광물이 일부 인지되는데(그림 4b), 이러한 문제점들은 차후 정확한 분석이 가능한 시료량과 X-선의 주사시간, 점토광물의 침전시간 등의 새로운 분석조건을 찾아내야 할 것이다. 그럼에도 불구하고 본 연구로부터 개발된 분석법은 정성분석 면에서 상당히 간편하고 정확한 결과를 낼 수 있기 때문에 야외에서 얇게 관찰되는 단층암 이외에도 퇴적암내의 수 mm 두께의 얇은 엽층리, 실내전단실험 후 회수된 소량의 단층물질, 그리고 대심도 시추코어 등 시료량이 매우 제한되어 있는 상황에서의 광물상 분석연구 측면에서도 폭넓게 기여할 수 있을 것으로 기대된다.
Acknowledgments
이 연구는 경상대학교 학술진흥지원사업 신임교원 연구기반조성연구비의 지원으로 수행되었다. X-선 회절분석 결과의 해석에 도움을 주신 안동대 정기영 교수님과 심사과정에서 유익한 조언을 해주신 추창오 박사님 및 익명의 심사위원께 감사드린다.
References
-
Aretusini, S., Mittempergher, S., Plümper, O., Spagnuolo, E., Gualtieri, A.F., and Di Toro, G., (2017), Production of nanoparticles during experimental deformation of smectite and implications for seismic slip, Earth and Planetary Science Letters, 463, p221-231.
[https://doi.org/10.1016/j.epsl.2017.01.048]
-
Bestmann, M., Pennacchioni, G., Frank, G., Göken, M., and Wall, H., (2011), Pseudotachylyte in muscovite-bearing quartzite: Coseismic friction-induced melting and plastic deformation of quartz, Journal of Structural Geology, 33, p169-186.
[https://doi.org/10.1016/j.jsg.2010.10.009]
-
Carpenter, B., Marone, C., and Saffer, D., (2009), Frictional behavior of materials in the 3D SAFOD volume, Geophysical Research Letters, 36, pL05302.
[https://doi.org/10.1029/2008GL036660]
-
Carpenter, B., Marone, C., and Saffer, D., (2011), Weakness of the San Andreas Fault revealed by samples from the active fault zone, Nature Geoscience, 4, p251-254.
[https://doi.org/10.1038/ngeo1089]
- Chae, B.-G., and Chang, T.W., (1994), Movement history of Yangsan fault and its related fractures at Chongha- Yongdok area, Korea, Journal of the Geological society of Korea, 30, p379-394, (in Korean with English abstract).
-
Chester, F.M., and Chester, J.S., (1998), Ultracataclasite structure and friction processes of the Punchbowl fault, San Andreas system, California, Tectonophysics, 295, p199-221.
[https://doi.org/10.1016/S0040-1951(98)00121-8]
-
Choo, C.O., and Chang, T.W., (2000), Characteristics of clay minerals in gouges of the Dongrae fault, Southeastern Korea, and implications for fault activity, Clays and clay minerals, 48, p204-212.
[https://doi.org/10.1346/CCMN.2000.0480206]
-
Collettini, C., Carpenter, B.M., Viti, C., Cruciani, F., Mollo, S., Tesei, T., Trippetta, F., Valoroso, L., and Chiaraluce, L., (2014), Fault structure and slip localization in carbonate-bearing normal faults: An example from the Northern Apennines of Italy, Journal of Structural Geology, 67, p154-166.
[https://doi.org/10.1016/j.jsg.2014.07.017]
-
De Paola, N., Collettini, C., Faulkner, D.R., and Trippetta, F., (2008), Fault zone architecture and deformation processes within evaporitic rocks in the upper crust, Tectonics, 27, pTC4017.
[https://doi.org/10.1029/2007TC002230]
-
De Paola, N., Hirose, T., Mitchell, T., Di Toro, G., Viti, C., and Shimamoto, T., (2011), Fault lubrication and earthquake propagation in thermally unstable rocks, Geology, 39, p35-38.
[https://doi.org/10.1130/G31398.1]
-
Di Toro, G., Goldsby, D.L., and Tullis, T.E., (2004), Friction falls towards zero in quartz rock as slip velocity approaches seismic rates, Nature, 427, p436-439.
[https://doi.org/10.1038/nature02249]
-
Faulkner, D.R., Lewis, A.C., and Rutter, E.H., (2003), On the internal structure and mechanics of large strike-slip fault zones: field observations of the Carboneras fault in southeastern Spain, Tectonophysics, 367, p235-251.
[https://doi.org/10.1016/S0040-1951(03)00134-3]
-
Fujimoto, K., and Sato, K., (2017), Studies of montmorillonite mechanochemically decomposed at different water contents, Geomaterials, 7, p41-50.
[https://doi.org/10.4236/gm.2017.72004]
-
Han, R., Hirose, T., Jeong, G.Y., Ando, J., and Mukoyoshi, H., (2014), Frictional melting of clayey gouge during seismic fault slip: Experimental observation and implications, Geophysical Research Letters, 41, p5457-5466.
[https://doi.org/10.1002/2014GL061246]
-
Han, R., Hirose, T., Shimamoto, T., Lee, Y., and Ando, J., (2011), Granular nanoparticles lubricate faults during seismic slip, Geology, 39, p599-602.
[https://doi.org/10.1130/G31842.1]
-
Han, R., Shimamoto, T., Ando, J., and Ree, J.-H., (2007a), Seismic slip record in carbonatebearing fault zones: an insight from high-velocity friction experiments on siderite gouge, Geology, 35, p1131-1134.
[https://doi.org/10.1130/G24106A.1]
-
Han, R., Shimamoto, T., Hirose, T., Ree, J.-H., and Ando, J., (2007b), Ultralow friction of carbonate faults caused by thermal decomposition, Science, 316, p878-881.
[https://doi.org/10.1126/science.1139763]
-
Hayward, K.S., Cox, S.F., Fitz Gerald, J.D., Slagmolen, B.J.J., Shaddock, D.A., Forsyth, P.W.F., Salmon, M.L., and Hawkins, R.P., (2016), Mechanical amorphization, flash heating, and frictional melting: Dramatic changes to fault surfaces during the first millisecond of earthquake slip, Geology, 44, p1043-1046.
[https://doi.org/10.1130/G38242.1]
-
Hirono, T., Asayama, S., Kaneki, S., and Ito, A., (2016), Preservation of amorphous ultrafine material: A proposed proxy for slip during recent earthquakes on active faults, Scientific Reports, 6, p36536.
[https://doi.org/10.1038/srep36536]
-
Ikari, M.J., Marone, C., and Saffer, D.M., (2011), On the relation between fault strength and frictional stability, Geology, 39, p83-86.
[https://doi.org/10.1130/G31416.1]
-
Ishikawa, T., Tanimizu, M., Nagaishi, K., Matsuoka, J., Tadai, O., Sakaguchi, M., Hirono, T., Mishima, T., Tanikawa, W., Lin, W., Kikuta, H., Soh, W., and Song, S.-R., (2008), Coseismic fluid–rock interactions at high temperatures in the Chelungpu fault, Nature Geoscience, 1, p679.
[https://doi.org/10.1038/ngeo308]
-
Janssen, C., Wirth, R., Lin, A., and Dresen, G., (2013), TEM microstructural analysis in a fault gouge sample of the Nojima Fault Zone, Japan, Tectonophysics, 583, p101-104.
[https://doi.org/10.1016/j.tecto.2012.10.020]
-
Janssen, C., Wirth, R., Rybacki, E., Naumann, R., Kemnitz, H., Wenk, H.R., and Dresen, G., (2010), Amorphous material in SAFOD core samples (San Andreas Fault): Evidence for crush-origin pseudotachylytes?, Geophysical Research Letters, 37, pL01303.
[https://doi.org/10.1029/2009GL040993]
-
Kim, C.-M., Han, R., Jeong, G.Y., Jeong, J.O., and Son, M., (2016), Internal structure and materials of the Yangsan fault, Bogyeongsa area, Pohang, South Korea, Geosciences Journal, 20, p1-15.
[https://doi.org/10.1007/s12303-016-0019-8]
-
Kirkpatrick, J., Rowe, C., White, J., and Brodsky, E., (2013), Silica gel formation during fault slip: Evidence from the rock record, Geology, 41, p1015-1018.
[https://doi.org/10.1130/G34483.1]
-
Kuo, L.-W., Song, S.-R., Suppe, J., and Yeh, E.-C., (2016), Fault mirrors in seismically active fault zones: A fossil of small earthquakes at shallow depths, Geophysical Research Letters, 43, p1950-1959.
[https://doi.org/10.1002/2015GL066882]
-
Lee, S.K., Han, R., Kim, E.J., Jeong, G.Y., Khim, H., and Hirose, T., (2017), Quasi-equilibrium melting of quartzite upon extreme friction, Nature Geoscience, 10, p436.
[https://doi.org/10.1038/ngeo2951]
-
Lin, A., (1994), Glassy pseudotachylyte veins from the Fuyun fault zone, northwest China, Journal of Structural Geology, 16, p71-83.
[https://doi.org/10.1016/0191-8141(94)90019-1]
-
Oohashi, K., Hirose, T., and Shimamoto, T., (2011), Shear-induced graphitization of carbonaceous materials during seismic fault motion: Experiments and possible implications for fault mechanics, Journal of Structural Geology, 33, p1122-1134.
[https://doi.org/10.1016/j.jsg.2011.01.007]
-
Scuderi, M.M., Collettini, C., Viti, C., Tinti, E., and Marone, C., (2017), Evolution of shear fabric in granular fault gouge from stable sliding to stick slip and implications for fault slip mode, Geology, 45, p731-734.
[https://doi.org/10.1130/G39033.1]
-
Sibson, R.H., (2003), Thickness of the Seismic Slip Zone, Bulletin of the Seismological Society of America, 93, p1169-1178.
[https://doi.org/10.1785/0120020061]
-
Siman-Tov, S., Aharonov, E., Sagy, A., and Emmanuel, S., (2013), Nanograins form carbonate fault mirrors, Geology, 41, p703-706.
[https://doi.org/10.1130/G34087.1]
-
Smith, S.A.F., Billi, A., Toro, G.D., and Spiess, R., (2011), Principal Slip Zones in Limestone: Microstructural Characterization and Implications for the Seismic Cycle (Tre Monti Fault, Central Apennines, Italy), Pure and Applied Geophysics, 168, p2365-2393.
[https://doi.org/10.1007/s00024-011-0267-5]
-
Viola, G., Scheiber, T., Fredin, O., Zwingmann, H., Margreth, A., and Knies, J., (2016), Deconvoluting complex structural histories archived in brittle fault zones, Nature Communications, 7, p13448.
[https://doi.org/10.1038/ncomms13448]
-
Viti, C., (2011), Exploring fault rocks at the nanoscale, Journal of Structural Geology, 33, p1715-1727.
[https://doi.org/10.1016/j.jsg.2011.10.005]
-
Wirth, R., (2009), Focused Ion Beam (FIB) combined with SEM and TEM: Advanced analytical tools for studies of chemical composition, microstructure and crystal structure in geomaterials on a nanometre scale, Chemical Geology, 261, p217-229.
[https://doi.org/10.1016/j.chemgeo.2008.05.019]
-
Yund, R.A., Blanpied, M.L., Tullis, T.E., and Weeks, J.D., (1990), Amorphous material in high strain experimental fault gouges, Journal of Geophysical Research: Solid Earth, 95, p15589-15602.
[https://doi.org/10.1029/JB095iB10p15589]