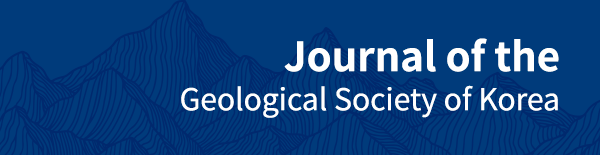
합천 운석충돌구 내 코사이트의 발견과 지질학적 의미: X-선 회절분석을 통한 접근
초록
합천 운석충돌구는 한반도에서 확인된 유일한 운석충돌구로 뚜렷한 테두리와 충돌이후 생성된 호성퇴적층이 관찰된다. 하지만 충돌 당시의 초고압 변성작용을 설명할 수 있는 고압 실리카 광물의 존재는 알려지지 않았다. 본 연구는 합천 운석충돌구에서 수집된 20HCL04 시추공의 65 m 깊이에서 채취된 특징적인 자갈시료들을 대상으로 운석 충돌의 광물학적 증거를 추적하였다. 기반암의 암편으로 식별되는 회색 내지 암회색 자갈시료의 경우 점토질 기질부에 모래 크기의 광물입자가 결합된 양상을 띠며, 석영-사장석-자철석-점토광물(일라이트-운모, 카올린, 스멕타이트, 녹니석 등)의 광물조합이 확인된다. 반면 밝은 회색조를 띠는 매끄러운 표면과 불균질한 공극이 관찰되는 암편은 은미정질 입자들의 응집체로 관찰되었으며 X-선 회절분석을 통하여 높은 비율의 비정질 물질과 코사이트를 함유하고 있음을 확인하였다. 충격에 의한 초고압 조건에서 생성되는 실리카 광물인 코사이트의 발견은 합천 운석충돌구가 운석 충돌로 형성된 구조임을 확증하는 최초의 광물학적 단서를 제공한다.
Abstract
The Hapcheon impact crater, characterized by its distinct rims and impact-induced subsurface lacustrine sedimentary features, is the only confirmed meteorite impact crater on the Korean Peninsula. However, the mineralogical indicators of ultra-high-pressure (UHP) metamorphism resulting from the impact have not been previously identified. In this study, we investigated the mineralogical composition of two distinctive pebbles retrieved from a depth of around 65.65 m in the sedimentary core 20HCL04, drilled within the impact crater. The gray to dark gray pebble specimen, which identified as bedrock fragments, is mainly composed of a clayey matrix with some sand-sized mineral particles. Its mineral assemblage consists of quartz, plagioclase, magnetite, and various clay minerals, including illite-mica, kaolinite, smectite, and chlorite. In contrast, the light grayish pebble specimen features a smooth surface with heterogeneous pores, and is mainly composed of aggregates of cryptocrystalline particles. X-ray diffraction analysis of the light gray pebble revealed a dominant presence of amorphous materials along with a crystalline phase of coesite, an ultra-high-pressure (UHP) polymorph of silica (SiO2). The identification of coesite provides the first direct mineralogical evidence of UHP metamorphism at the Hapcheon impact crater, confirming that the structure undersent extreme pressure conditions associated with a meteorite impact.
Keywords:
Hapcheon Impact Crater, shock metamorphism, ultra-high pressure metamorphism, coesite, X-ray diffraction analysis키워드:
합천 운석충돌구, 충돌변성, 초고압 변성작용, 코사이트, X-선 회절분석1. 서 론
합천 운석충돌구는 적중-초계 분지로도 알려져 있으며, 한반도에서 최초로 확인된 운석충돌구이다(Lim et al., 2021). 이 충돌구의 그릇 모양 형태와 최대 깊이 100 m에 이르는 깊은 퇴적 분지는 지구물리학적 연구를 통해 확인된 바 있다(Choi et al., 2021). 충돌의 증거로는 충격원뿔암(shatter cone), 석영 내 평면 변형 구조(Planar Deformation Features, PDF), 그리고 충돌 각력 내의 높은 농도의 백금족 원소가 있었다(Lim et al., 2021; Choi et al., 2022). 운석충돌구 충전 퇴적물은 충돌 각력으로 구성되어 있으며, 그 위에 호수와 하천 퇴적물이 덮여 있어 고기후 변화와 지역 지질학적 사건에 대한 중요한 기록을 제공할 수 있다(Lee et al., 2023). 다른 많은 운석충돌구 사례와 같이 비교적 잘 보존된 이 충돌구는 충돌 과정과 그것이 지구 지질에 미친 영향을 이해하는 데 중요한 통찰을 제공할 수 있다(Osinski et al., 2022).
특히 운석충돌구 내부에서 발견된 석영 내 평면 변형 구조, 충격원뿔암 및 충돌물질 등의 다양한 충돌 증거들은 이 지역의 지질학적 기원을 명확히 하는 데 중요한 역할을 한다(Lim et al., 2021; Lee et al., 2023). 이러한 증거들은 합천 충돌구가 운석 충돌에 의해 형성된 것임을 강력히 뒷받침하는 지질학적 흔적들이며, 당시의 초고압 환경 조건을 반영한다(Chao et al., 1960). 그러나, 현재까지 합천 운석충돌구에서는 코사이트(coesite)와 같은 고압 실리카 광물이 보고된 사례는 없다.
코사이트는 일반적으로 운석 충돌과 같은 극한의 고압 환경에서 석영이 변성되어 형성되는 실리카 다형체로, 그 존재는 충돌 당시의 고압 조건을 입증하는 데 중요한 역할을 한다(Folco et al., 2018). 특히 합천 운석충돌구의 기반암인 퇴적암과 같이 다공성 암석에서의 충격파는 공극을 통과하면서 국소적인 압력 증폭을 일으킬 수 있는데, 이 과정이 “Shock Pressure Effects in Porous Rocks”로 불리며, 코사이트 형성에 결정적인 역할을 한다(Kieffer, 1971). 다공성 사암에서 코사이트는 공극 붕괴에 따른 국소적 압력-온도 기울기에서 형성될 수 있으며, 이는 용융 또는 유리화 없이 고체 상태에서의 변형(subsolidus transformation)을 통해 발생할 수 있다고 알려져 있다(Folco et al., 2018). 결과적으로 이러한 운석 충돌과 관련한 코사이트의 형성 기작은 운석 충돌에 의해 발생하는 초고압 변성작용을 보다 명확히 이해하는 데 있어 매우 중요한 정보를 제공할 수 있다.
본 연구의 목적은 합천 운석충돌구의 퇴적물에서 코사이트의 존재를 확인하고, 이를 통해 이 지역의 충돌구 형성 과정과 충돌 압력 조건에 대한 정보를 제공하는데 있다. 이를 위하여 Lee et al. (2023)이 합천 운석충돌구의 퇴적기록 보고에서 사용한 시추코어 20HCL04에서 회수된 미고결 퇴적물 시료에서 충돌과 관련된 잔자갈 시료를 채취하여 암석-광물학적 접근을 통해 코사이트와 관련된 지질학적 증거를 제안하고자 한다.
2. 연구 지역
합천군 적중면과 초계면에 위치한 합천 운석충돌구는 남쪽이 높고 북쪽으로 갈수록 분지의 출구가 열려 있는 형태를 보인다(그림 1). 이 지역은 산으로 둘러싸인 자연적 방벽을 형성하고 있어, 충돌 후 형성된 퇴적물이 비교적 잘 보존되어 있다. 합천 운석충돌구의 기반암은 주로 백악기 및 중생대의 비해양 기원 경상계 퇴적암으로 구성되어 있으며, 동명층, 칠곡층, 신라층 등 다양한 퇴적층이 존재한다(Chang, 1968; Kim and Lee, 1969).
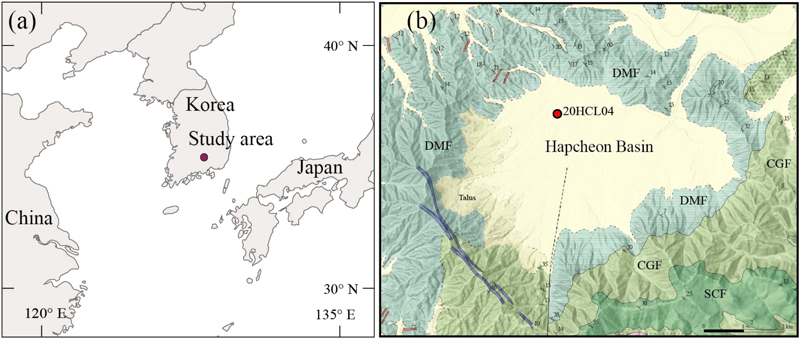
Location and geological map of the Hapcheon meteorite impact crater study area. a) Location of the study area. b) Geology of the Hapcheon Basin and the location of drilling core 20HCL04. The Dongmyeong Formation (DMF), Chilgog Formation (CGF), and Silla Conglomerate Formation (SCF) belong to the Cretaceous and Mesozoic non-marine formations (Chang, 1968; Kim and Lee, 1969; Lim et al., 2024).
동명층(DMF)은 주로 회색에서 녹색 회색의 사암과 셰일, 어두운 회색에서 검은색의 셰일, 모래 셰일, 석회암, 회색 점토암 및 응회암으로 구성되어 분지의 대부분을 둘러싸고 있으며, 상부의 칠곡층(CGF)은 최대 두께 800 m에 이르며, 다양한 색조의 셰일과 사암, 점토암, 석회암, 응회암 등을 포함한다. 또한 칠곡층을 덮고 있는 신라역암층(SCF)은 두께가 100~350 m에 이르며, 연구 지역의 동남부에 분포하고, 보라색과 회갈색의 응회암과 응회 사암, 다양한 점토암과 셰일, 실트암으로 구성된다(Chang, 1968; Kim and Lee, 1969; Lim et al., 2024).
연구지역에서 수행된 퇴적코어 조사를 기반으로 확인된 합천 운석충돌구는 충돌이후 형성된 호성 퇴적층의 특징이 보고되었다(Lim et al., 2021, 2024; Lee et al., 2023). 선행연구에서 공통적으로 확인되는 합천 운석충돌구 퇴적층의 암석학적 특징은 세 가지 단위로 구분된다[(1)상부로부터 주변 경사지 및 하천 기원의 조립질 자갈이 풍부한 토양 퇴적물, (2)실트-점토질 층이 관찰되는 세립질 호성 퇴적물, (3)충돌 각력암을 포함한 자갈이 우세한 사질 퇴적물]. 이와 같이 기반암과 명확하게 구분되는 퇴적물에 기록된 다양한 표면 풍화, 침식 및 충격 변성 작용 등의 다양한 지질학적 인자들은 충돌 후 생성된 호수에 퇴적층의 형성 및 진화과정을 보여준다.
3. 연구 방법
본 연구에서는 합천 운석충돌구의 충돌구 충전물 중에서 시추된 퇴적층을 대상으로 코사이트의 존재를 조사하였다. 연구 대상 시료는 그림 1과 같이 합천 운석충돌구의 북쪽 중앙부에서 2020년 시추된 20HCL04 시추공의 65 m 깊이에서 채취된 8 mm 이상 크기의 자갈 형태의 퇴적물이다. Lee et al. (2023)의 조사에 의하면 해당 층위는 각진 형태의 쇄설성 자갈을 포함하는 사질층이며, 쇄설물은 주로 기반 퇴적암이지만 충돌 분화구와 관련된 퇴적물(충돌 각력암 및 그 파편의 조각) 등 여러 유형의 자갈크기의 암편들을 포함한다(그림 2). 본 연구에서는 퇴적물코어에서 수집된 암편들 중 충돌과 무관한 기반암의 암편 성격의 자갈(N14 시료)과 코사이트와 같은 고압 실리카 광물의 존재 가능성을 평가하기 위한 자갈(N21 시료)을 각각 선정하여 특징을 비교하였다.
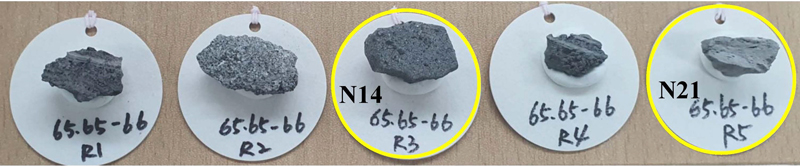
Photographs of pebbles observed at a depth of around 65.65 m in the Hapcheon drilling sediments. The yellow circles indicate the samples used for analysis, and the diameter of each circle is 3 cm.
각 시료는 육안 및 현미경관찰을 통한 암석기재학적 연구를 수행하였으며, 현미경에서 감정된 광물종의 교차검증 및 식별이 불가한 광물의 정확한 조성을 파악하기 위해 X-선 회절분석(XRD)을 수행하였다. XRD 분석은 한국지질자원연구원에서 운영 중인 PANalytical 사의 X'pert3 powder X-선 회절분석기를 이용하여 분말화 된 시료를 측정하였다. XRD 분석조건은 40 kV/30 mA의 가속전압으로 3-65° 2-theta의 구간을 주사간격 0.02° 2-theta, 주사시간 0.5 sec/step으로 설정하여 연속스캔(continuous scan) 방식으로 회절 값을 기록하였다. 또한 시료의 미세구조를 보다 정밀하게 분석하기 위해 주사전자현미경(Scanning electron microscope, SEM)을 사용하였다. SEM 촬영은 국립낙동강생물자원관에서 수행되었으며, 시료의 후방 산란 전자(Back scat-tered Electron, BSE) 이미지를 통해 미세구조를 관찰하였다. 이 과정에서 시료의 조직과 공극 구조, 그리고 광물 입자의 배열 상태 등을 확인하였다.
4. 연구 결과
4.1. 산출 양상 및 현미경 관찰 결과
연구지역 기반암의 자갈 파편인 N14는 점토입자로 구성된 기질부에 일부 모래 크기의 입자가 치밀하게 결합된 양상으로 산출되며 육안상 회색 내지 암회색을 띤다(그림 2). SEM 및 편광현미경 관찰결과(그림 3, 4), 수 백 μm 크기의 석영과 장석 입자가 반정으로 드물게 관찰된다. 시편의 대부분을 차지하는 세립질 기질부에서는 세립질 장석 및 석영 입자가 관찰되며 이들의 사이를 견운모 내지 일라이트화 된 점토광물들이 충전하고 있다. 또한 산화철을 함유하여 적갈색을 띠는 점토 응집체와 자철석으로 여겨지는 유색광물 입자의 존재가 확인되며, 이들의 경계부를 따라 일부 방해석의 소광특성을 보이는 세립질 입자가 관찰된다.
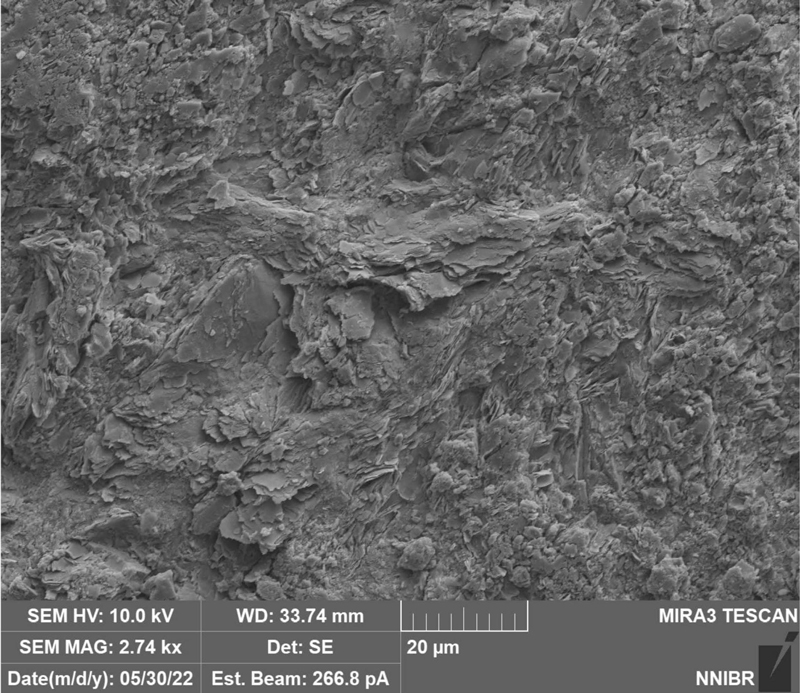
Scanning Electron Microscope (SEM) image showing the microstructure of sediment pebble sample N14 taken from the Hapcheon drilling core. The image shows irregularly arranged layered particles of various sizes. The magnification is 2.74 kx, and the scale bar represents 20 μm.
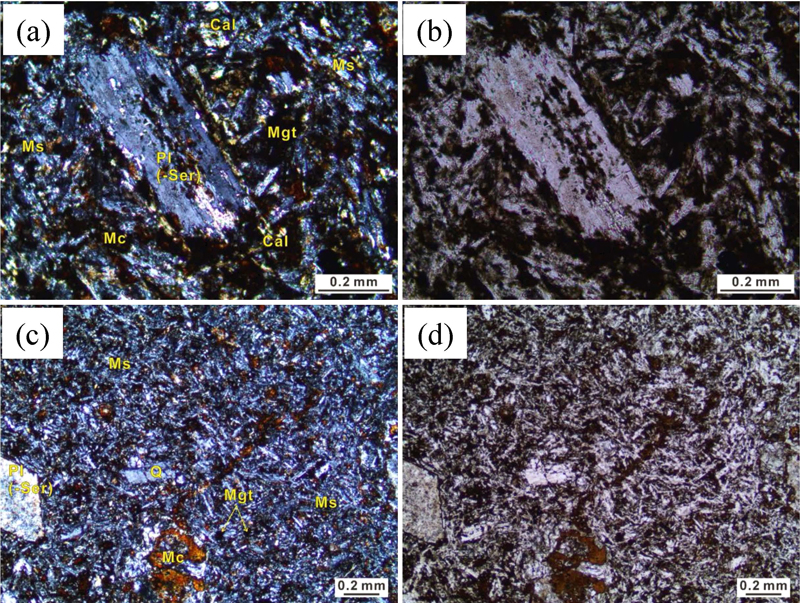
Photomicrographs of rock thin sections under a polarizing microscope. (a) and (c) are images of sample N14 taken under crossed nicols. (b) and (d) are also images of sample N14 taken under open nicols. The scale bar represents 200 micrometers. Cal: calcite, Mc: clayey matrix, Mgt: magnetite, Ms: siliceous matrix, Pl: plagioclase, Ser: sericite.
운석 충돌에 의하여 변질된 암편(N21)의 경우 밝은 회색조를 띠는 매끄러운 표면 질감을 가지며, SEM 관찰시에는 불균질한 공극이 다수 관찰된다(그림 2, 5). 편광현미경 하에서 N21 시편은 소광이 일어나지 않는 은미정질 입자들의 응집체로 구성된 기질부가 대부분을 이루고 있는 것으로 관찰된다(그림 6). 한편 공극을 충전하거나 기질부를 교대하며 존재하는 갈색 점토 응집체와 자형 내지 반자형의 수 μm 내외 입자들도 소량 관찰된다(그림 6). 하지만 너무 작은 입자크기로 인하여 이들의 광물종을 식별할 수 있는 광학 및 구조적 특성의 수집에는 어려움이 있다.
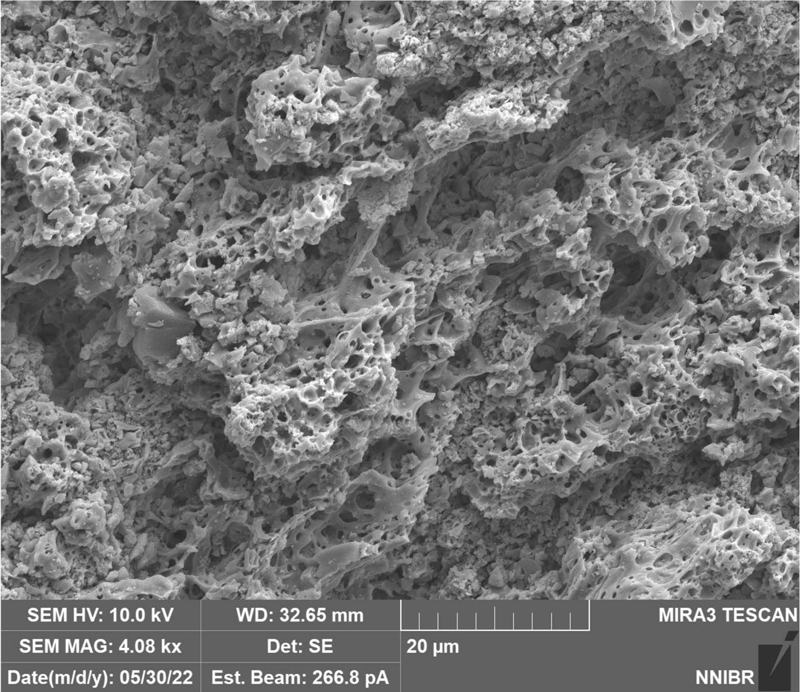
Scanning Electron Microscope (SEM) image showing the microstructure of a sediment pebble sample N21 from the Hapcheon drilling core. The image shows a porous texture with a complex network of interconnected voids and their walls. The magnification is 4.08 kx, and the scale bar represents 20 μm.
4.2. X-선 회절분석 기반 광물조성
편광현미경 관찰 결과와의 비교분석 및 세부적인 광물조성을 파악하기 위하여 수행한 XRD 분석결과를 그림 7과 그림 8에 제시하였다.
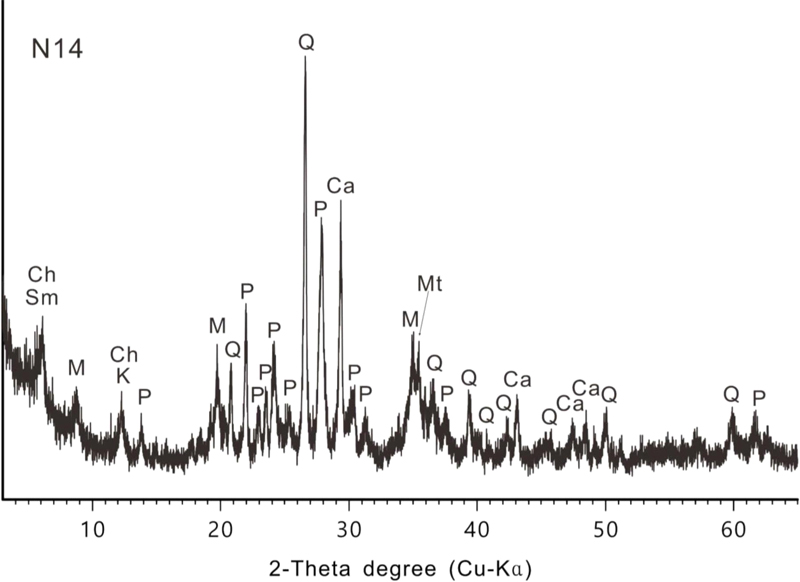
X-ray diffraction (XRD) pattern of a sediment sample N14 showing the mineral composition and relative abundance. The peaks in the diffraction pattern correspond to different minerals identified in the sample, including illite-mica (M), plagioclase (P), calcite (Ca), quartz (Q), chlorite (Ch), kaolin (K), magnetite (Mt), and smectite (Sm).
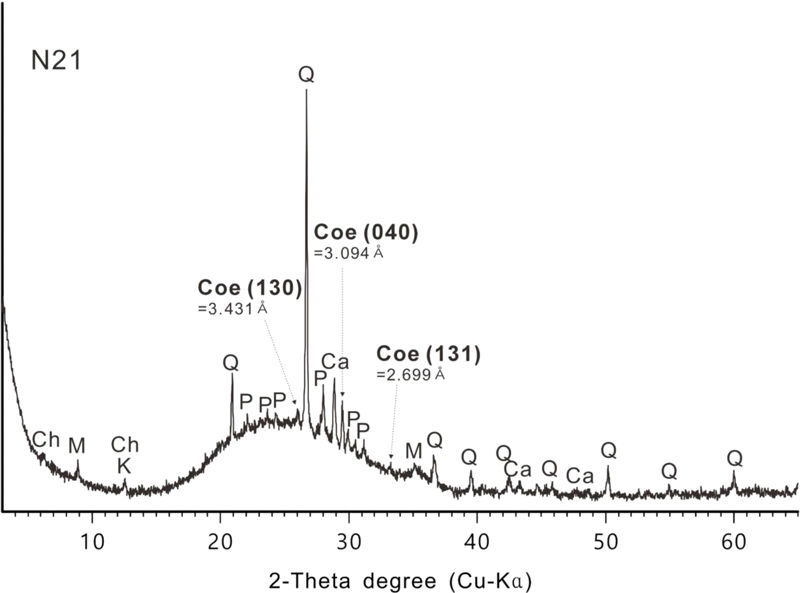
X-ray diffraction (XRD) pattern of sample N21, showing the mineralogical composition and their relative abundances. The predominant phase is an unidentified amorphous material. The identified crystalline mineral components were considered as derived from the sediments, such as illite-mica (M), plagioclase (P), calcite (Ca), quartz (Q), chlorite (Ch), and kaolin (K). In addition, the remarkable XRD peaks were scanned that assigned to the crystalline phase of coesite (Coe; an ultra-high pressure metamorphic polymorph of SiO2).
N14의 경우 사장석, 석영, 방해석, 자철석 및 점토광물(일라이트-운모군 광물, 카올린군 광물, 스멕타이트군 광물, 녹니석군 광물) 등의 회절패턴이 검출되어 연구지역 기반암의 박편에서 관찰된 광물조성과 잘 일치하였다(그림 7).
시편 N21의 XRD 패턴은 15-35° 2-theta 영역에서 강한 비정질의 상승패턴을 기록하는 것이 특징적이다. 또한 3.431 Å, 3.094 Å 및 2.699 Å의 저면간격을 나타내는 회절각도에서 시편 N14에서 발견되지 않은 결정질상의 회절패턴이 기록되었는데, 이들은 각각 코사이트의 (130), (040) 및 (131) 저면반사에 할당되어 시편 N21 내에서 코사이트가 존재함을 증명한다(그림 8). 추가적으로, 기반암의 흔적으로 판단되는 석영, 사장석, 방해석 및 점토광물들 또한 수반광물로 확인된다.
5. 토 의
5.1. 충돌 압력의 해석
코사이트가 발견된 자갈 시료 N21은 원형 내지 타원형의 기공이 발달한 다공성 조직을 보이며(그림 5), 은미정질 입자가 응집된 것처럼 분포하는 기질부가 관찰된다(그림 6). 이와 같은 비정질 기반의 복잡한 조직은 부석과 같이 화산활동에 의하여 생성되는 암편에서도 흔하게 관찰되지만(Kieffer, 1971; Osinski et al., 2022), 시료 N21과 같이 코사이트의 존재가 확인되는 암편의 비정질 성분은 고압의 압축과 감압 과정에서 생성된 기반 퇴적암의 부분용융물질이 급냉된 흔적임을 지시한다(Kieffer, 1971; Stöffler, 1971). 이는 운석 충돌의 충격파가 다공성 암석을 통과하면서 국소적으로 압력을 증폭시켰음을 시사한다. 이러한 압력 증폭 효과는 “Shock Pressure Effects in Porous Rocks”로 알려져 있으며, 코사이트 형성에 중요한 역할을 한다(Kieffer, 1971).
광물성분 분석에 의해 확인되는 합천 운석충돌구에서의 코사이트 발견은 충격 변성의 고압 조건을 시사하며, 이는 다른 운석충돌구에서의 연구 결과와 일치한다. 운석충돌구에서 코사이트가 처음으로 발견된 사례는 미국 애리조나의 Meteor Crater에서였다(Chao et al., 1960). 이 발견은 충격 변성 연구의 기초를 다졌으며, 이후 독일의 Ries Basin (Stöffler, 1971), 캐나다의 Wanapitei crater (Dence et al., 1974), 남아프리카 공화국의 Vredefort Dome 등 다양한 충돌구에서 추가적인 코사이트 발견이 이루어졌다(Spray and Boonsue, 2017). 이러한 연구들은 각각의 충돌구에서 발생한 충격 압력과 형성 메커니즘을 이해하는 데 중요한 기초 자료를 제공한다. 예를 들어, Ries Basin에서의 연구는 약 30 GPa의 충격 압력을 제안하였고(Stöffler, 1971), Vredefort Dome에서는 최대 35 GPa에 달하는 압력 조건이 코사이트 형성에 관여했음을 보여주었다(Kieffer, 1971; Spray and Boonsue, 2017). 이처럼 코사이트의 존재는 운석충돌구의 형성 과정과 관련된 중요한 지표로 사용될 수 있다. 결과적으로 합천 충돌구에서의 유사한 압력 조건은 이 지역이 과거 극한의 충격을 경험했음을 나타내며, 이를 통해 충돌 당시의 압력 조건을 30 GPa 이상으로 평가할 수 있다.
5.2. 지질학적 의미
본 연구에서 입증한 합천 운석충돌구 퇴적물 내 코사이트의 발견은 해당 지역이 운석 충돌에 의해 형성된 충돌분지임을 강하게 시사하며, 이 지역의 충격 변성 메커니즘을 규명하는 데 중요한 지질학적 의미를 갖는다.
운석충돌구의 충격암을 포함하여 그와 관련된 각력암 및 암편 내에서 보고된 코사이트의 경우 일반적으로 비정질 유리기질부 내에 미세결정 함유물(micro-crystalline inclusion) 형태로 나타난다(Kieffer, 1971; Stöffler, 1971; Stöffler and Langenhorst, 1994; Jackson et al., 2016; Osinski et al., 2022). 본 연구의 SEM 이미지는 시료 N21 내에서 확인된 공극들의 미세구조를 보여주며(그림 5), X-선 회절분석 결과에서는 고배율의 현미경 관찰에서도 발견되지 않았던 뚜렷한 코사이트의 구조가 기록되었다(그림 6, 8). 이를 통하여 합천 운석충돌구에서 생성된 코사이트 또한 비정질 기질부에 미세결정 형태로 존재하는 것으로 판단되며, 추후 미세영역에 대한 라만분광 및 현미분석 등의 추가연구를 통하여 더욱 구체적이고 명확한 지질학적 검증이 가능할 것으로 사료된다.
합천 운석충돌구에서의 코사이트 발견은 그 사실만으로도 이 지역의 충격 변성 메커니즘을 규명하는 데 있어 중요한 지질학적 의미를 가진다. 코사이트의 존재는 이 충돌구가 운석 충돌로 형성된 구조임을 확증하는 추가적인 증거가 되며, 충돌 당시의 고압 조건을 명확히 입증한다고 할 수 있다(Kieffer, 1971). 합천 충돌구와 같이 기반암이 퇴적암인 지역에서 형성된 코사이트는 다공성 암석 내에서의 국소적 압력 증폭 효과를 통해 형성되었을 가능성이 높다. 이러한 발견은 충돌 메커니즘과 관련하여 새로운 시각을 제공하며, 운석 충돌이 지구 지질에 미친 영향을 보다 구체적으로 이해하는 데 기여할 수 있다. 뿐만 아니라 이 지역에서 발견된 코사이트의 존재는 기존에 보고된 합천 운석충돌구의 지질학적 증거인 PDF와 충격원뿔암의 발견을 보완하는 광물학적 증거이며, 충격의 강도와 메커니즘을 보다 정밀하게 이해할 수 있는 단서를 제공하므로 이 지역의 지질학적 사건을 이해하는 데 중요한 정보를 제공한다.
6. 결 론
본 연구는 합천 운석충돌구에서 코사이트를 최초로 보고하고, 이를 통해 이 지역의 충돌구 형성 과정과 관련된 중요한 지질학적 증거를 제시하였다. 고압 실리카 광물의 존재는 초고압 변성작용을 일으킬 수 있는 충돌 당시의 극한의 환경을 입증하며, 이를 바탕으로 충격 변성 메커니즘을 보다 깊이 이해할 수 있는 기초 자료를 제공한다는 점에서 향후 추가적인 시료 분석과 현장 조사를 통해 합천 충돌구의 형성 메커니즘을 더욱 구체적인 연구가 요구된다. 이를 통해 합천에서 발생한 운석 충돌이 한반도를 포함한 지구 지질에 미친 영향에 대해 보다 나은 해석을 제공할 수 있을 것으로 기대된다.
Acknowledgments
이 연구는 2025년도 한국지질자원연구원의 주요사업인 ‘국가지질도·지질주제도 디지털전환(DX)과 기반암 내 유망·유해원소 연구(3)(GP2025-032)’의 결과물이다. 심사과정에서 건설적이고 유익한 조언과 비평을 해주신 익명의 두 심사위원과 편집위원에게 감사드린다. 좋은 SEM 사진을 촬영해주신 국립낙동강생물자원관 이상득 박사님께 감사드린다.
References
- Chang, K.H., 1968, Explanatory text of the geological map of Habcheon sheet (1:50,000). Geological Survey of Korea, p. 21 (in Korean with English summary).
-
Chao, E.C.T., Shoemaker, E.M. and Madsen, B.M., 1960, First natural occurrence of coesite. Science, 132, 220-222.
[https://doi.org/10.1126/science.132.3421.220]
-
Choi, S.H., Lee, J.H., Kim, S.S., Yu, J. and Puchtel, I.S., 2022, Meteoritic component in impact breccias of the Jeokjung-Chogye structure, South Korea: Evidence from the HSE abundances and Re-Os isotopic systematics. Chemical Geology, 613, 121145.
[https://doi.org/10.1016/j.chemgeo.2022.121145]
-
Choi, S., Kim, S.W., Choi, E.K. and Lee, Y.C., 2021, Estimating the impact process of the Jeokjung-Chogye Basin in Korea from gravity field interpretation. Geophysical Journal International, 228, 1457-1463.
[https://doi.org/10.1093/gji/ggab410]
-
Dence, M.R., Robertson, P.B. and Wirthlin, R., 1974, Coesite from the Lake Wanapitei crater, Ontario. Earth and Planetary Science Letters, 22, 118-122.
[https://doi.org/10.1016/0012-821X(74)90071-5]
-
Folco, L., Mugnaioli, E., Gemelli, M., Masotta, M. and Campanale, F., 2018, Direct quartz-coesite transformation in shocked porous sandstone from Kamil Crater (Egypt). Geology, 46, 739-742.
[https://doi.org/10.1130/G45116.1]
-
Jackson, J.C., Horton Jr, J.W., Chou, I.-M. and Belkin, H.E., 2016, Coesite in suevites from the Chesapeake Bay impact structure. Meteoritics & Planetary Science, 51, 946-965.
[https://doi.org/10.1111/maps.12638]
-
Kieffer, S.W., 1971, Shock metamorphism of the Coconino Sandstone at Meteor Crater, Arizona. Journal of Geophysical Research, 76, 5449-5473.
[https://doi.org/10.1029/JB076i023p05449]
- Kim, K.W. and Lee, Y.J., 1969, Explanatory text of the geological map of Changryeong sheet (1:50,000). Geological Survey of Korea, p. 18 (in Korean with English summary).
-
Lee, J., Shin, S., Yoon, H.H., Kim, J.C., Choi, Y., Nahm, W. and Kim, H., 2023, The sedimentary records of the Hapcheon impact crater basin in Korea over the past 1.3 Ma. Frontiers in Earth Science, 11.
[https://doi.org/10.3389/feart.2023.1102785]
-
Lim, J., Hong, S.S., Han, M., Yi, S. and Kim, S.W., 2021, First finding of impact cratering in the Korean Peninsula. Gondwana Research, 91, 121-128.
[https://doi.org/10.1016/j.gr.2020.12.004]
-
Lim, J., Park, S., Jung, A. and Kim, S.W., 2024, Post-impact lake environment changes in the Hapcheon impact crater, Korea. Episodes, 47, 477-496.
[https://doi.org/10.18814/epiiugs/2024/02403s16]
-
Osinski, G.R., Grieve, R.A.F., Ferrière, L., Łosiak, A., Pickersgill, A.E., Cavosie, A.J., Hibbard, S., Hill, P.J.A., Bermúdez, J., Marion, C.L., Newman, J.D. and Simpson, S., 2022, Impact Earth: A review of the terrestrial impact record. Earth-Science Reviews, 232, 104112.
[https://doi.org/10.1016/j.earscirev.2022.104112]
-
Spray, J.G. and Boonsue, S., 2017, Quartz-coesite-stishovite relations in shocked metaquartzites from the Vredefort impact structure, South Africa. Meteoritics and Planetary Science, 53, 93-109.
[https://doi.org/10.1111/maps.12997]
-
Stöffler, D., 1971, Coesite and stishovite in shocked crystalline rocks. Journal of Geophysical Research, 76, 5474-5488.
[https://doi.org/10.1029/JB076i023p05474]
-
Stöffler, D. and Langenhorst, F., 1994, Shock metamorphism of quartz in nature and experiment: I. Basic observation and theory. Meteoritics, 29, 155-181.
[https://doi.org/10.1111/j.1945-5100.1994.tb00670.x]