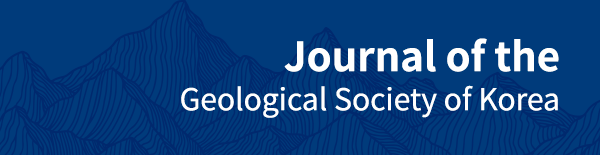
제주도 현무암류의 감람석에 포획된 멜트 포유물의 지화학성분에 기초한 근원 맨틀 물질 연구
초록
한반도 상부맨틀에 존재하는 불균질성의 특성을 파악하고자 제주도 현무암류의 감람석 반정(Fo77-83)에 포획된 멜트 포유물의 주성분과 미량원소 성분을 분석하였다. 가열하여 재균질화한 멜트 포유물의 주성분 원소 중량(SiO2 = 47.2-54.5%, MgO = 5.5-8.7%, Al2O3 = 14.1-16.8%, K2O = 0.6-3.2%)은 전암 현무암(SiO2 = 53.2-55.7%, MgO = 5.0-6.0%, Al2O3 = 14.7-15.4%, K2O = 0.6-0.8%)에 비해 다양성을 보인다. 아알칼리계열에 속하는 전암 현무암류와 달리 일부의 멜트 포유물은 알칼리 조면현무암으로 분류되며, 고-알루미나 알칼리 소그룹에 속하기도 한다. 멜트 포유물의 (La/Yb)N 비는 5.1-16.2로 전암 값(2.4-5.5)에 비해서 넓은 범위를 띤다. 이런 다양성은 근원 맨틀에 이질성이 있으며, 현무암질 멜트는 맨틀에서 분별용융되어 생성되고, 차후 지각 마그마 방에서 혼합된다는 것을 의미한다. 제주 멜트 포유물에서 관찰되는 K, Ba, Pb, Eu에서의 양(+)의 이상과 Al2O3 부화 등은 근원 맨틀 속에 K-hollandite가 포함되어 있음을 시사한다. 멜트 포유물에서 관찰되는 P에서의 양의 이상과 양의 값으로 분별된(Zr/Hf)N 비로부터, 인회석 또는 그 고압상과 (함)탄산염 에클로자이트의 존재 가능성이 유추된다. 이런 부화물질은 고-태평양판의 섭입과정에 동반되어 맨틀 전이대에 정체되어 있다가, 상승하는 플룸 속에 수반되어 제주도 화산활동에 연루되었을 것으로 추정된다.
Abstract
We determined the major and trace element concentrations of olivine (Fo77-83)-hosted melt inclusions from Jeju basaltic rocks in order to understand the nature of upper mantle heterogeneity beneath the Korean Peninsula. Heated and re-homogenized melt inclusions show a wider compositional range in major element concentrations (SiO2 = 47.2-54.5%, MgO = 5.5-8.7%, Al2O3 = 14.1-16.8%, K2O = 0.6-3.2%) than the host basaltic rocks (SiO2 = 53.2-55.7%, MgO = 5.0-6.0%, Al2O3 = 14.7-15.4%, K2O = 0.6-0.8%). Unlike host basaltic rocks, which belong to the sub-alkaline series, some melt inclusions are classified as an alkaline series or the high-alumina alkaline sub-group. The (La/Yb)N ratios of melt inclusions range from 5.1 to 16.2, which is a wide range compared to those of the host basaltic rocks (2.4 to 5.5). This diversity suggests that the upper mantle beneath Jeju Island is heterogeneous, and that basaltic melts are produced by fractional melting in the mantle and subsequently mixed in crustal magma chambers. Positive anomalies in K, Ba, Pb and Eu, and relative enrichments in Al2O3 observed in Jeju melt inclusions suggest that K-hollandites may reside in the mantle source. Positive anomaly in P, and the positively fractionated (Zr/Hf)N ratios of the Jeju melt inclusions indicate the possible presence of apatite or its high-pressure phase, and carbonated eclogite in the mantle source. The enriched materials residing in the stagnant paleo-Pacific slab in the mantle transition zone might have been entrained by the ascending plume, resulting in the Jeju volcanism.
Keywords:
Jeju Island, basalt, melt inclusion, K-hollandite키워드:
제주도, 현무암, 멜트 포유물1. 서 언
멜트 포유물(melt inclusion)은 맨틀이나 지각에서 빠르게 성장하는 결정 속에 갇혀 있는 마그마 성분의 액상 방울이다. 멜트 포유물은 모광물(host mineral)이 불규칙적으로 성장하는 동안 갇혀 있다가, 모암이 냉각하는 동안 유리질로 급냉하거나 부분적으로 또는 완전히 결정화된다. 결정이 성장하는 동안 포획된 멜트 포유물은 포획 순간에 존재하는 마그마를 샘플링한다. 만일 마그마 시스템에서 이런 포획 과정이 심부에서 발생한다면, 멜트 포유물의 성분은 천부에서 발생하는 정출작용, 지각혼염 및 마그마 혼합과 같은 과정에 의해서 바뀌기 이전의 초기 마그마 성분에 대한 정보를 제공할 수 있다(e.g., Sobolev and Shimizu, 1993; Saal et al., 1998; Schiano, 2003). 그러나 멜트 포유물은 포획된 후 잔류 마그마가 냉각하거나 정출작용을 겪는 동안 딸광물을 정출한다거나 또는 포유물의 벽에 모광물이 정출한다거나 혹은 수축 기포(shrinkage bubble)의 형성 등에 의해서 성분이 변화될 수 있다(e.g., Roedder, 1984; Cottrell et al., 2002; Danyushevsky et al., 2002). 그러나 차후의 재균질화(rehomogenization) 실험이나 또는 수치적 재건(numerical reconstruction) 과정을 통하여 초기 성분으로 복원할 수 있다(e.g., Danyushevsky et al., 2002; Schiano, 2003; Kent, 2008).
감람석은 대부분의 경우 고철질 마그마에서 가장 먼저 정출하는 규산염광물이다(e.g., Wallace et al., 2021). 감람석은 대개의 현무암질 원소에 대해서 불호정적이기 때문에, 재가열 실험 동안 또는 포획 후 모광물과의 상호작용 등에 의해서 멜트 포유물의 성분이 바뀔 가능성 등에서 자유롭다(Cottrell et al., 2002; Spandler et al., 2007). 따라서 감람석에 포획된 멜트 포유물은 초기 마그마 성분에 가까워 맨틀에서의 부분용융 과정, 맨틀 이질성, 마그마 혼합 과정 등에 대한 정보를 제공할 수 있다(e.g., Maclennan, 2008a, 2008b; Choi, S.H. et al., 2013; Choi, H.-O. et al., 2017). 심부에서 형성된 마그마가 천부 맨틀에서 반응에 의해서 감람석을 정출할 수도 있으므로(Kelemen et al., 1995), 감람석에는 지각 내 마그마 방에서 혼합되기 이전의 다양한 성분의 멜트가 포획되어 있을 수 있다.
제주도는 판내부 환경에서 생성된 제4기 화산섬이다(e.g., Lee, 1982; Park and Kwon, 1993; Tatsumi et al., 2005; Choi et al., 2006; Brenna et al., 2010; Koh et al., 2013; Kim et al., 2019). 제주도 화산암은 주로 알칼리계열의 현무암-조면안산암-조면암으로 구성되어 있지만, 소량의 아알칼리(subalkaline)의 쏠리아이트(tholeiite)계열 현무암-현무암질 안산암이 알칼리계열의 암석 사이에 협재되어 있다(Choi et al., 2006; Brenna et al., 2012; Baek et al., 2014; Choi, 2022). Sr-Nd-Pb 동위원소 조성에 의하면 제주도 현무암류는 결핍된 중앙해령현무암의 근원맨틀(DMM, Depleted MORB Mantle)과 부화맨틀 타입2(EM2, Enriched Mantle Type 2)의 조합으로 구성된 맨틀에서 기원한 것으로 알려져 있다(e.g., Choi et al., 2006; Kim et al., 2019; Choi and Liu, 2022). 이는 한반도 타지역 제4기 화산(백두산, 울릉도, 독도, 백령도, 전곡, 간성 등)암이 DMM-EM1 (Enriched Mantle Type 1)의 조합으로 구성된 맨틀에서 유래된 것과는 상반된다(Choi et al., 2006, 2020; Kim and Choi, 2021; Choi and Liu, 2022). EM1형 단성분(end-member)의 역할을 하는 물질로는 약 2.0 Ga 전에 섭입되어 맨틀 전이대에 정체되어(stagnant) 있는 (함)K-hollandite 원양 퇴적물이 추정되고 있다(Kuritani et al., 2011, 2013; Wang et al., 2017a; Choi et al., 2020; Kim and Choi, 2021; Choi and Liu, 2022). 반면에 EM2형 단성분은 맨틀로 섭입된 후 상대적으로 빠른 시간 안에 재활성화된 원양 점토 퇴적물일 가능성이 제기된 바 있다(Choi, 2022; Choi and Liu, 2022; Kim et al., 2023). 그러나 EM1과 EM2형 원양 퇴적물의 조성과 생성 연대의 차이가 발생한 근본적인 원인에 대해서는 아직까지 충분히 논의되지 못하였다. K-hollandite는 정장석의 고압상(>~10 GPa)이다(e.g., Schmidt, 1996; Rapp et al., 2008). 한반도 하부에 있는 맨틀 내 부화 물질의 이질성에 대한 보다 정밀한 규명을 위하여, 본 연구에서는 제주도 현무암질 암석 중에서 직경 1mm 이상 크기의 감람석 반정을 가지고 있는 시료를 대상으로 감람석 속에 포획되어 있는 멜트 포유물과 전암(wholerock)의 주성분과 미량원소 성분 분석을 시도하였다. 본 연구를 통하여 우리는 제주도 현무암류를 생성한 근원 맨틀 속에도 K-hollandite가 존재할 가능성에 대해서 처음으로 보고한다. 아울러 인회석 또는 그 고압상과 (함)탄산염 에클로자이트의 존재 가능성에 대해서도 논의한다.
2. 분석방법
본 연구를 위해서 감람석 반정을 포함하고 있는 신선한 현무암질 암석 시료를 제주도 함덕리와 남원읍 지역에서 채취하였다(그림 1). 전암 분석을 위한 시료는 텅스텐 카바이드 절구로 직경 5 mm 미만으로 분쇄한 후 Milli-Q로 세척하고, 마노 볼 밀(agate ball mill)을 사용하여 분말을 만들었다. 주성분 함량은 부경대학교에서 X-선 형광 분광법(XRF)으로 분석했다. 표준 시료인 BIR-1과 MO-5로 생성한 가중 회귀선을 사용하여 데이터를 처리하였으며, 이에 기반한 분석 정밀도는 5% 이내였다(표 1). 전암의 미량원소 함량은 캐나다 온타리오주의 ActLab에서 유도결합 플라즈마 질량 분석기(ICP-MS)를 사용하여 측정하였다. 정밀도는 표준시료(BIR-1, JR-1)의 동시 분석에 기반하여 ±10%로 추정된다(표 1).
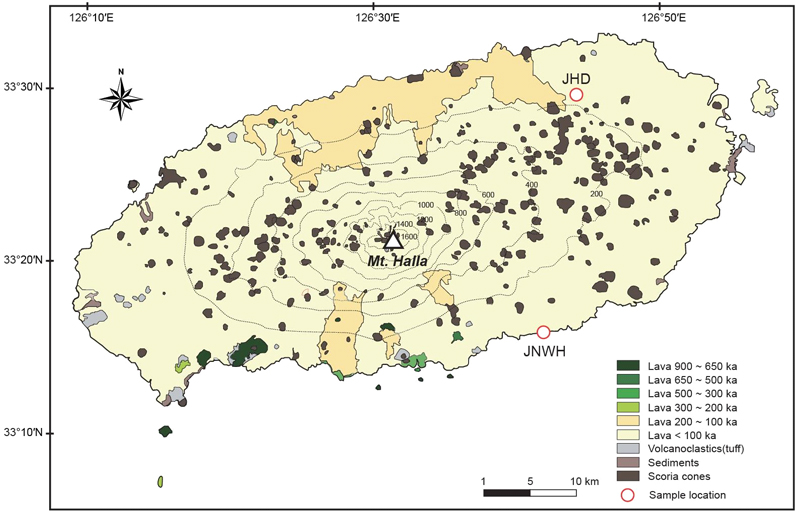
Simplified geological map of Jeju Island (after Koh et al., 2013; Kim et al., 2019) with sample locations.
포획 당시 멜트 포유물의 성분으로 복원하기 위하여 프랑스 Clermont-Ferrand의 Magmas et Volcans 연구소에서 Vernadsky형 가열 현미경 스테이지(Sobolev et al., 1980)를 사용하여 가열 실험을 수행하였다. Iron-Wüstite 버퍼에 해당하는 산화·환원 조건하의 정제된 He 대기에서 길이 6mm의 수직 Pt90Rh10 튜브 속에서 감람석을 가열하여 포유물(유리질, 가스, 딸광물)을 균질화하였다. 온도는 시료 홀더에 용접된 Pt-Pt90Rh10 열전대(thermocouple)를 통해 조정되었다. 온도 측정의 정확도는 약 ±20℃였으며, 시스템은 Au의 용융 온도(1064℃)로 보정하였다. 모든 실험은 포유물의 변형 속도 변화로 인한 효과에 근접하기 위해 20℃~900℃에서는 0.9℃/s, 900℃ 이상에서는 0.4℃/s의 가열 속도로 수행되었다. 멜트 포유물의 균질화 온도는 1218-1293℃로 다양했다(표 2). 급냉시킨 후 시료를 연마하여 멜트 포유물을 표면으로 노출시킨 후 전자현미경 분석을 수행하였다.

Uncorrected major element concentrations (wt.%) of reheated melt inclusions in olivine phenocrysts from Jeju basaltic rocks.
가열하여 균질화된 멜트 포유물과 모광물인 감람석의 주성분 원소 함량은 Magmas et Volcans 연구소에서 CAMECA SX-100 전자현미분석기를 사용하여 측정하였다. 유리질 시료에 대한 분석은 주성분 원소(Na, Al, Si, Ca, Fe, Mg, Ti, Mn, K, P)의 경우 15 kV, 8 nA에서, S의 경우 15 kV, 80 nA에서 디포커싱(defocusing) 빔(5-10 μm)을 사용하여 수행하였다. 감람석 분석은 빔 직경 1 μm, 빔 전류 15 nA에서 분석하였다. 측정 시간은 피크의 경우 10초, 배경의 경우 5초였다. S는 통합모드에서 각각 10초씩 5단계로 분석하였다. S의 검출 한계는 ~50 ppm이었고, 분석 불확도는 10% 이내였다. 보정에는 천연 및 합성 광물과 산화물을 사용하였다. 다만, S는 스미소니언 마이크로빔 표준에 따라 VG2 현무암 유리질로 보정하였다. 유리질 분석의 경우, 국제 유리질 표준시료인 A99(킬라우에아 유리질 현무암, Jarosewich et al., 1979; Thornber et al., 2002)를 사용하여 실험실 간 교차검증을 수행했다. 결과는 표 3, 4에 나타내었다.

Trace element concentrations (ppm) of reheated melt inclusions in olivine phenocrysts from Jeju basaltic rocks.
재가열된 멜트 포유물의 미량원소 성분은 Magmas et Volcans 연구소에서 레이저 삭박 유도결합 플라즈마 질량 분석기(LA-ICP-MS)로 분석하였다. 이 장비는 Excimer ArF 레이저(193 nm)와 Agilent 7500 CS 사중극자 질량분석기로 구성되어 있다. 분석에는 각 멜트 포유물의 노출면적보다 작은 스팟이 사용되었으며, 그 크기는 10~20 µm로 다양했다. 레이저는 2 Hz로 펄싱되었고, 데이터 수집 시간은 배경의 경우 30초, 피크의 경우 60초였다. 멜트 포유물 삭박 전과 다섯 번의 배치마다 기준 물질인 NIST-610 및 NIST-612를 분석하여 시그널 변화를 테스트하고 보정하였다. 분석의 정확성을 확인하기 위해 미국 지질조사국(USGS)의 컬럼비아강 현무암(BCR) 유리질 표준시료를 함께 분석하였다. 데이터 처리에 사용된 NIST-610 및 NIST-612의 보정 값은 Gagnon et al. (2008)의 것을 사용하였다. 미량원소 성분은 내부 표준으로 Ca의 미량 동위원소(43Ca 및 44Ca)를 사용하여 시료와 표준물질 간의 삭박 효율 변화에 대해 보정하였다. 멜트 포유물의 CaO 함량은 사전에 전자현미분석기를 사용하여 측정하였다. BCR-2를 여러 번 분석한 결과, 보고된 대부분의 원소에 대해 분석 재현성과 정확도가 10%보다 좋았다(표 4). 적절한 분석 동위원소를 선택하였고 또 +ThO/+Th <0.5%로 분자 종의 형성을 최소화했기 때문에, 간섭 분자 또는 동중원소에 대한 보정이 필요하지 않았다. 결과는 표 4에 나타내었다.
3. 결 과
3.1. 전암
전암의 주성분과 미량원소 함량은 표 1에 나타내었다. SiO2 함량은 53.2-55.7 wt.%, MgO 함량은 5.0-6.0 wt.%이며, Mg#[=100Mg/(Mg+Fe2+)]는 54.0-61.7이다. 이때 전암의 Mg#는 Fe2O3/FeO가 0.15라고 가정하고 계산되었다. 총 알칼리 대 실리카 도(일명 TAS도; 그림 2)에서, 연구 시료는 아알칼리계열의 현무암질 안산암으로 분류된다. Ni, Co, Cr 함량은 각각 60-100 ppm, 24-39 ppm, 160-320 ppm이다. 콘드라이트 값에 표준화한 희토류원소 분포도(그림 3)에서, 시료는 경희토류 원소에 부화되어 있으며, (La/Yb)N 값은 2.4-5.5 범위이다(표 1). 남원 시료의 경우 Eu에서 양(+)의 이상이 관찰된다. 시원적 맨틀 값에 표준화한 미량원소 분포도(일명 거미도; 그림 4)에서, 전형적인 해양도현무암(OIB, oceanic island basalt)처럼 불호정성이 매우 높은 원소(highly incompatible element)에 부화되어 있으며, 인접한 경희토류 원소에 비해 Nb-Ta 같은 고장력원소(HFSE, high-field-strength element)에도 부화되어 있다. 그러나 전형적인 OIB에 비해 Th과 Pb에서의 부화가 관찰되며, 남원 시료의 경우 Sr과 P에서의 부화가 특징적이다.
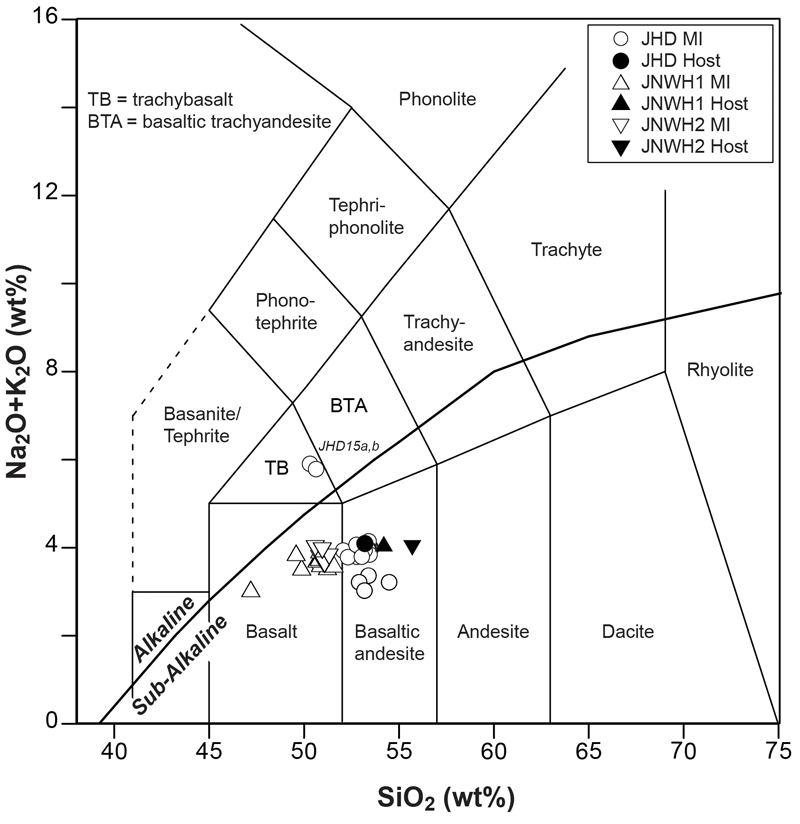
Total alkali versus silica plots for corrected melt inclusions in olivines and host basaltic rocks. The boundary lines dividing alkaline and sub-alkaline series is from Irivine and Baragar (1971). MI = melt inclusion.
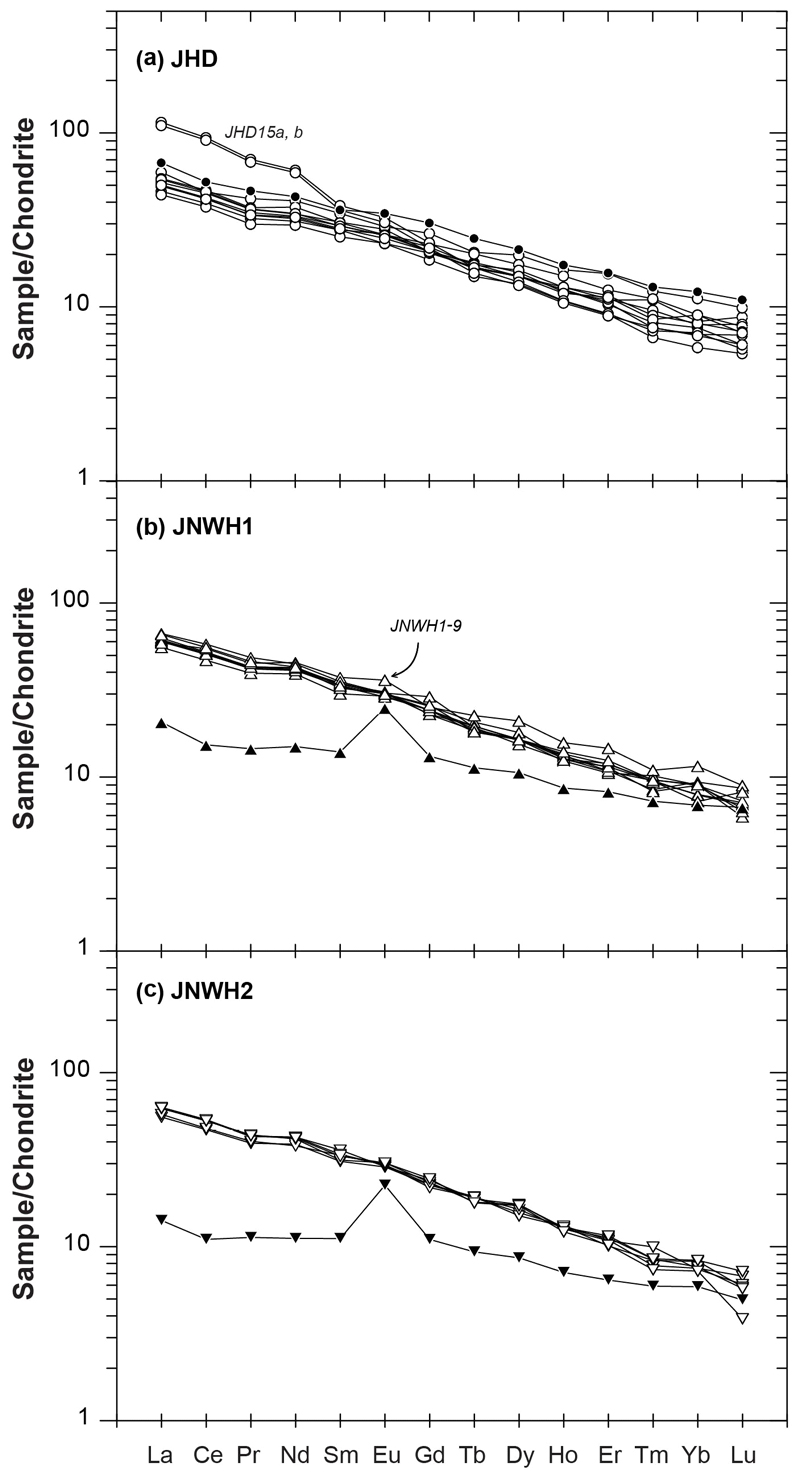
Rare earth element concentrations normalized to chondrite value (Sun and McDonough, 1989) for melt inclusions in olivines and host basaltic rocks. Symbols as in Fig. 2.
3.2. 감람석 반정
멜트 포유물을 가지고 있는 감람석 반정의 주성분 원소 함량을 표 3에 나타내었다. 감람석의 Fo 값은 76.8-83.1이다. CaO 함량은 0.20-0.27 wt.%로, 맨틀 페리도타이트를 구성하는 감람석의 값(CaO < 0.1 wt.%; Thompson and Gibson, 2000)보다 매우 높다(그림 5). 따라서 연구 대상인 감람석은 맨틀 포획광물(xenocryst)이 아니라 현무암 반정이다. 감람석의 Fo 값과 전암의 Mg#간의 상관관계를 그림 6에 나타내었다. 감람석과 현무암질 액의 Fe-Mg 분배계수는 실험에 의해서 잘 구해져 있으며, 1기압에서 0.30 ± 0.03이고 5-15 kbar에서 0.31-0.34인 것으로 알려져 있다(Roeder and Emslie, 1970). 연구 대상인 감람석 반정은 전암인 현무암질 암석과 평형상태를 이루고 있다(그림 6).
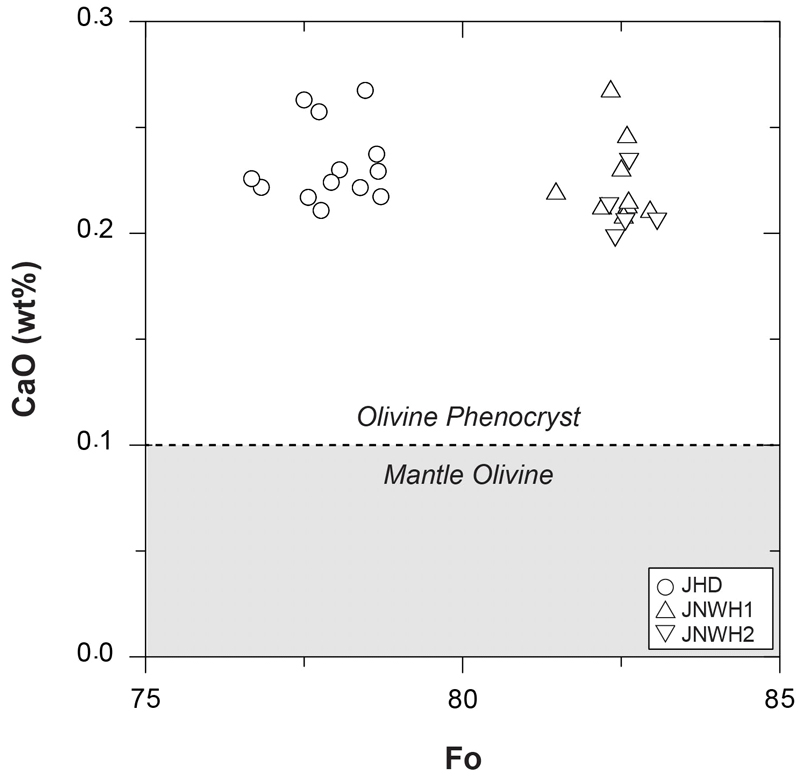
CaO contents vs. Fo values of melt inclusion-bearing olivines from Jeju basaltic rocks. The dashed line dividing magmatic phenocrysts from mantle olivines on the basis of CaO is from Thompson and Gibson (2000).
3.3. 멜트 포유물
멜트 포유물의 주성분 원소 함량은 표 2에 나타내었다. 분석된 멜트 포유물의 직경은 23-90 μm이다(표 2). 재가열 실험 동안 멜트 포유물이 과열로 터지게 되면 휘발성분은 쉽게 확산되어 빠져나가게 된다. 이를 모니터링 하기 위해서 S 함량을 함께 측정하였다. S 함량은 한 개의 시료(JHD-2)를 제외하고는 100 ppm에서 1,710 ppm으로(표 2) 유리질 중앙해령현무암(S = ~400-1,800 ppm)이나 해양도현무암(S = ~700-2,200 ppm)의 값(Mathez, 1976; Yi et al., 2000; Stolper et al., 2004; Kendrick et al., 2014)에 준한다. 재가열 실험을 거친 일부의 멜트 포유물 속에서 구형의 황화물이 관찰되는 것(그림 7)과 또 시료 JHD-2의 멜트 성분이 동일 시료내 타 멜트 포유물의 것과 비교했을 때 유의미한 차이가 관찰되지 않는 점(표 2, 4, 5) 등으로 미루어 보았을 때 일부 낮은 S 함량을 가지는 시료는 레이저 점 분석 동안에 포유물 전체를 한꺼번에 분석하지 못해서 발생한 문제인 것으로 파악된다.
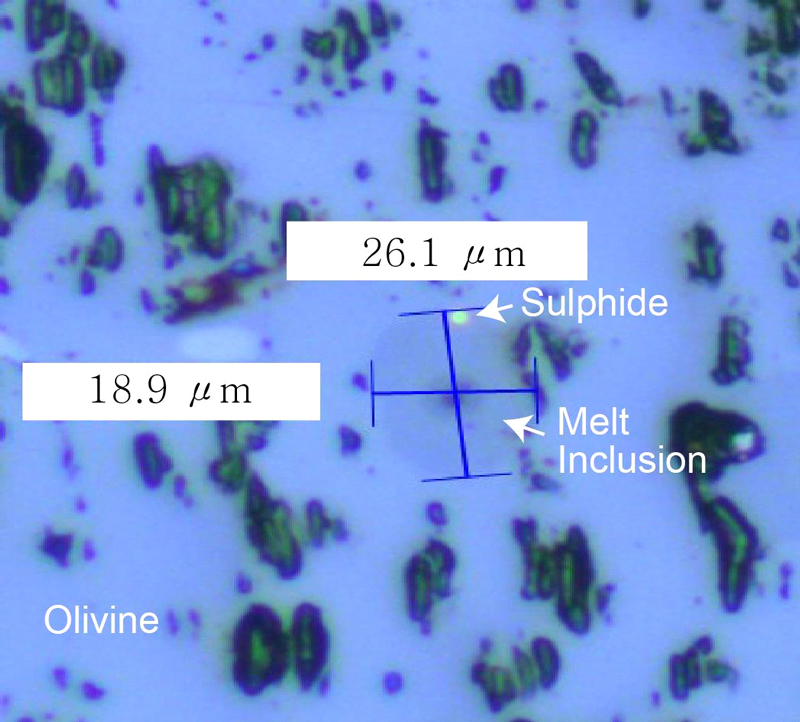
Representative reflected light image of sulphide globule in reheated melt inclusion in olivine phenocryst (JNWH1-10b).

Corrected major element concentrations (wt.%) of reheated melt inclusions in olivine phenocrysts from Jeju basaltic rocks.
감람석과 멜트 포유물 사이의 평형상태 여부를 점검하기 위하여, 멜트 포유물(Liq)과 모체 감람석(Ol) 사이의 Mg-Fe 분배계수 KD [= (Fe2+/Mg)Ol/(Fe2+/Mg)Liq]를 계산하였다. 계산된 KD 값은 0.34-0.58로 1기압에서 공인된 값인 0.30 ± 0.03 (Roeder and Emslie, 1970) 보다 매우 높은 편이다. 포획된 후 냉각되는 동안 멜트 포유물과 모광물 사이의 경계를 따라서 모광물이 추가 정출하기도 한다. 이 과정은 포획된 멜트와 모광물 사이의 비평형상태를 초래한다. 감람석에 포획된 멜트 포유물의 경우, 철 손실(즉, KD 증가)이라는 재평형 작용이 다수의 사례에서 관찰되었다(e.g., Sobolev and Danyushevsky, 1994; Danyushevsky et al., 2000). 이러한 효과를 보정하고 모체와 평형을 이루도록 복원하는 방법이 Danyushevsky and Plechov (2011)에 의해 개발되었으며, 이렇게 복원된 조성은 포획 당시의 포유물의 원래 조성에 가까운 근사치라고 추정된다. 다만 계산 과정에서 포획 당시 멜트 포유물의 초기 FeOT 성분이 가정되어야 하는 문제가 있으나, 제주도의 경우 MgO 함량이 4 wt.% 이하가 될 때까지는 Fe2O3T의 양이 약 12 wt.%로 일정하게 유지된다는 선행연구 결과(e.g., Kim et al., 2019)가 있으므로, 본 연구에서는 멜트 포유물의 FeOT를 10.8 wt.%로 가정하여 Danyushevsky and Plechov (2011)가 제안한 “Petrolog3” 프로그램을 사용하여 멜트 포유물의 초기 성분 값을 복원하였다. 그 결과는 표 5에 나타내었다. 감람석과 보정된 멜트 포유물 성분 간의 KD 값은 0.30-0.34로 실험으로 구해진 값 범위 내에 있다.
멜트 포유물의 SiO2 함량은 47.2-54.5 wt.%이며, Na2O와 K2O는 각각 0.5-3.7 wt.% 및 0.6-3.2 wt.%이다(그림 8). TAS도(그림 2)에서 멜트 포유물은 대체로 아알칼리계열의 현무암-현무암질 안산암에 도시된다. 그러나 일부의 시료(JHD15a, b)는 알칼리계열의 조면현무암으로 분류된다(그림 2). MgO 함량은 5.5-8.7 wt.%이며, Al2O3와 CaO 함량은 각각 14.1-16.8 wt.% 및 7.6-11.0 wt.%로 다양하다(그림 8).
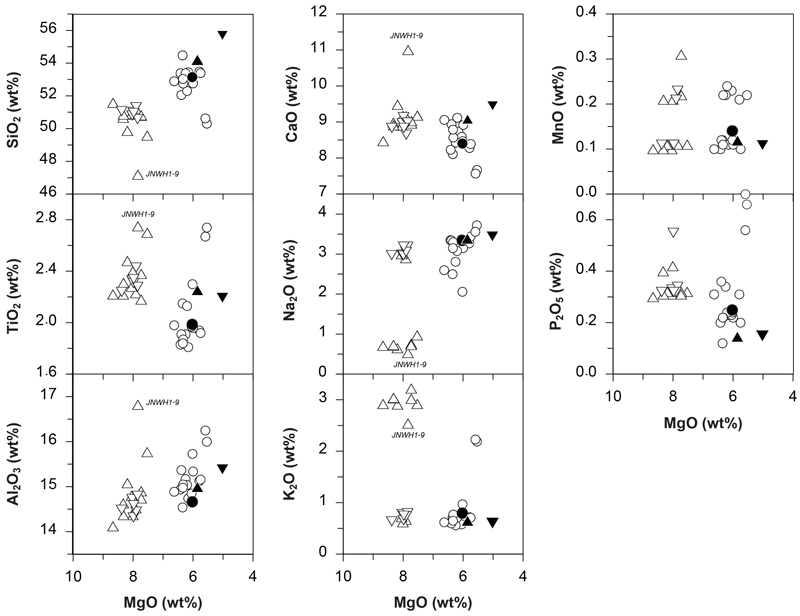
Major oxide variations of corrected melt inclusions in olivines and host basaltic rocks. MI = melt inclusion. Symbols as in Fig. 2.
멜트 포유물의 미량원소 성분은 표 4에 나타내었다. 중희토류 원소에 비해 경희토류 원소에 부화된 특징을 보이며, (La/Yb)N 값은 대개 5.1-9.2나 일부의 시료(JHD15a, b)는 16.1-16.2로 높은 편이다. 거미도(그림 4)에서, OIB나 전암 현무암 성분처럼 불호정성이 매우 높은 원소에 부화되어 있고, (Nb/La)N 비는 1보다 크다. 그러나 전체적으로 Pb에서 양의 이상이 관찰되며, Hf에 비해 Zr에 부화되어 있다. 일부의 시료는 Ba, K, Sr 및 P에서 양의 이상을 보인다.
4. 토 의
현재 연구 대상 감람석은 이들을 포함하고 있는 현무암질 마그마와 평형상태이다(그림 6). 즉, 분석된 감람석은 현무암질 마그마의 고결정(antecryst) 이나 포획결정(xenocryst)이 아니라 반정이다. 따라서 감람석 반정에 포획된 멜트 포유물은 이들을 운반한 현무암질 마그마가 지각의 마그마 방에서 혼합되기 이전의 성분에 관한 정보를 제공할 수 있다(e.g., Maclennan, 2008a).
제주 멜트 포유물의 주성분과 미량원소 성분 함량은 전암에 비해서 넓은 변화 폭의 성분 범위를 가진다(그림 4, 8). 예를 들어 멜트 포유물의 SiO2, Al2O3, K2O 함량은 47.2-54.5 wt.%, 14.1-16.8 wt.% 및 0.6-3.2 wt.%로 전암의 함량(SiO2 = 53.2-55.7 wt.%, Al2O3 = 14.7-15.4 wt.%, K2O = 0.6-0.8 wt.%)에 비해서 다양하다. (La/Yb)N 비는 5.1-16.2로 전암 값(2.4-5.5)에 비해서 넓은 범위를 띤다(표 4).
주성분원소 함량의 다양성은 현무암질 마그마의 근원 맨틀이 암상 또는 성분적으로 이질성이 있으며, 멜트는 맨틀에서 분별용융되어 생성되고, 차후 지각 마그마 방에서 혼합된다는 것을 나타낸다(e.g., Anderson, 1976; Maclennan et al., 2003; Maclennan, 2008a, 2008b). 즉, 감람석 정출작용이 시작되기 전에 마그마 혼합이 완료되지는 않았다. 함덕 시료(JHD)의 경우 JHD15a, b를 제외하고는 (La/Yb)N 비가 5.2-7.9로 전암의 값(5.5)과 거의 유사하다(표 4; 그림 3a). 그러나 남원 시료(JNWH)의 경우는 전암의 (La/Yb)N 비는 2.4-3.0인데 반해, 멜트 포유물의 것은 5.1-9.2로 상당히 높은 편이다(표 4; 그림 3b, 3c). (La/Yb)N 비는 부분용융 정도를 반영하는 값으로 부분용융 정도가 낮을수록 그 값은 커진다(e.g., Kim et al., 2019). 즉, 남원 멜트 포유물은 용융 초기 단계에서 낮은 정도로 분별용융된 멜트가 감람석 반정에 포획된 것이다. 반면에 전암 현무암은 다양한 분별용융 멜트의 혼합물이다. 함덕 시료 중 JHD15a, b의 (La/Yb)N 비는 16.1-16.2로 전암에 비해 매우 높고(표 4; 그림 3a), 아알칼리 계열인 전암과 달리 알칼리계열의 조면현무암으로 분류된다(그림 2). 알칼리계열의 멜트가 아알칼리계열의 전암 속에 포획되어 있다는 사실은 제주도의 알칼리와 아알칼리 계열 화산암류가 유사한 근원물질이 서로 다른 정도로 부분용융되어 생성된 것이라는 선행연구의 결과(e.g., Kim et al., 2019)를 지지한다.
남원 현무암류는 멜트 포유물과 달리 Eu과 Sr에서 매우 특징적인 양의 이상을 보인다(그림 3, 4). 따라서 전암에서의 Eu과 Sr 이상은 초기 마그마의 생성과정에서 획득된 것이 아니라 분화하는 동안 지각과의 혼염에 의해서 생성되었을 가능성이 크다. Eu과 Sr은 희토류원소 분포도나 거미도에서 인접한 희토류원소에 비해 상대적으로 사장석에 호정적인 원소이다(e.g., DEu = ~0.6; DSr = ~2.0; Rollinson, 1993). Eu-Sr에서의 양의 이상이 관찰되는 점, 전형적인 OIB와 달리 주어진 SiO2 함량 대비 상대적으로 높은 Al2O3 함량을 가지는 점, 그리고 제주도 남원 일대에서 회장암 단괴가 현무암에 포획되어 있다는 사실 등에 비추어, 제주도 현무암류가 지각에서 분화하는 동안 회장암질 암석을 혼염하였을 가능성이 제기된 바 있다(Tastumi et al., 2005; Kim and Choi, 2012; Baek et al., 2014; Kim et al., 2019). 제주도 화산암은 세 개의 소그룹 즉, 쏠리아이트(TH), 고-알루미나 알칼리(HAA), 저-알루미나 알칼리(LAA)로 분류된다(Tatsumi et al., 2005; Brenna et al., 2010, 2012; Baek et al., 2014; Kim et al., 2019) (그림 9). 이때 회장암질암과의 혼염 정도는 LAA-HAA-TH 순으로 증가하는 경향이 있다(Baek et al., 2014; Kim et al., 2019). 연구대상 현무암류는 모두 TH 소그룹에 속하므로(그림 2), 현재의 자료는 선행연구 결과를 뒷받침한다. 한편 전암과는 달리 일부 멜트 포유물에서 관찰되는 K, Ba 또는 P에서의 양의 이상(그림 4)이나 Al2O3 함량의 농집(JNWH1-9; 그림 8, 9)은 주목할 만 하다. 특히 JNWH1-9 시료는 전암이나 타 멜트포유물과는 달리 HAA 소그룹으로 분류되며(그림 9), Eu에서 양의 이상도 관찰된다(그림 3b).
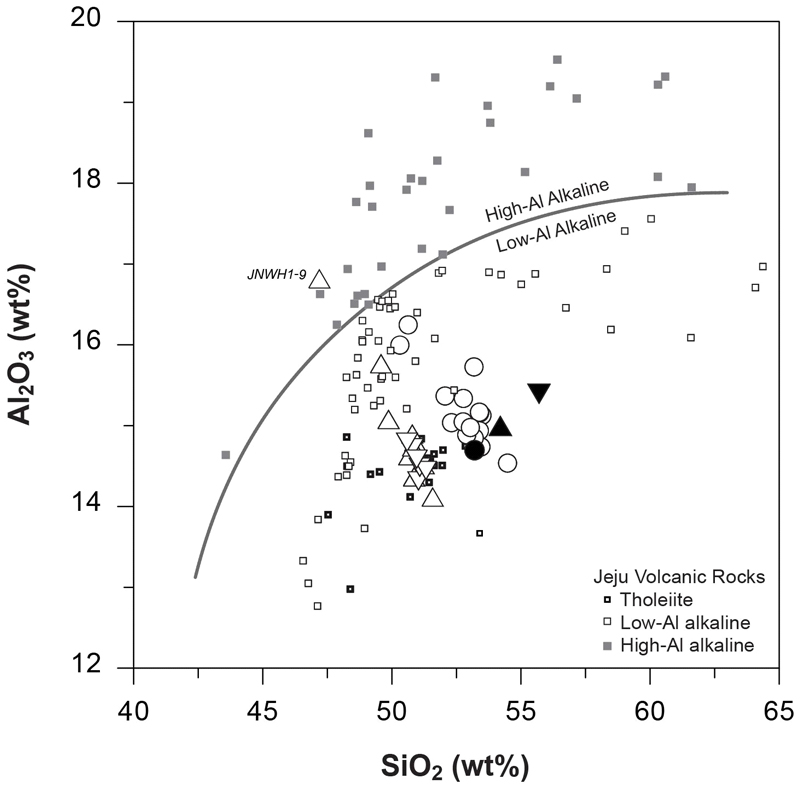
Al2O3 vs. SiO2 plot of the corrected melt inclusions in olivines and host basaltic rocks. The empirical line between the high-Al alkali and low Al-alkali suites is from Baek et al. (2014). Data for Jeju volcanic rocks is from Choi (2022). Symbols as in Fig. 2.
멜트 포유물에서 관찰되는 미량원소 함량의 다양성은 마그마가 하부지각에서 집적암(cumulate rocks) 내지는 반려암질 결정죽(crystal mush)과의 국부적 반응작용 또는 마그마 방이나 화도 주변의 규장질 지각암석과의 혼염 과정에 의해서 생성될 수도 있다(e.g., Danyushevsky et al., 2004; Yaxley et al., 2004). 예를 들어, Sr 또는 Ba에서의 부화작용은 사장석과의 반응에 의해 국지적으로 생성될 수 있다. 그러나 이런 과정에서 생성된 멜트는 수반된 광물에 불호정적인 원소에는 매우 결핍되어 있는 경향이 있다(e.g., Danyushevsky et al., 2004). 예를 들어 사장석과의 반응의 경우, 사장석에 불호정적인 원소인 중희토류원소(HREE)에는 상대적으로 결핍되어 있으면서 Sr과 Ba에는 매우 부화되어 있는 멜트가 생성될 수 있는 것이다. 그러나 K에서 양의 부화를 보이는 제주 멜트에서 타멜트 성분 대비 중희토류원소 등의 상대적 결핍은 관찰되지는 않는다는 점(그림 4)에서 (함)K 광물(예, 정장석)과의 반응에 의한 성분변화의 가능성은 배제될 필요가 있다.
제주 회장암 단괴는 주로 사장석(An39-69)과 소량의 단사휘석으로 구성되어 있으며, 정장석은 포함되어 있지 않다(Kim and Choi, 2012). 제주 회장암 단괴와 영남육괴의 산청·하동 회장암체의 광물 조성 및 Sr 동위원소 자료에 기반하여, Kim et al. (2019)는 산청·하동 회장암이 제주도 지각 하부로 연장되어 있을 가능성을 제시한 바 있다. 영남육괴의 산청·하동 회장암은 주로 사장석(An56-72)으로 구성되어 있고, 소량의 각섬석과 티탄철석 및 저어콘, 배덜리아이트(baddeleyite), 사방휘석 등으로 구성되어 있다(Ko, 2006; Lee et al., 2017). 산청 회장암의 사방휘석 내 Al2O3 함량으로 추정된 정치(emplacement) 깊이는 약 0.3-0.4 GPa이며, 생성연대는 약 1.9 Ga이다(Lee et al., 2017). 이는 범 지구 원생대 회장암체가 약 30-40 km 깊이의 모호면에 걸린 현무암질 마그마 방에서 결정화작용에 의해 생성된 부유성 사장석 위주의 결정죽이 중간 지각 깊이에서 응집되어 생성되었다는 주장(e.g., Ashwal and Bybee, 2017)과도 일치한다. 주성분과 미량원소 성분에 기초한 모델링에 의하면, 제주 현무암 마그마가 맨틀에서 분리된 깊이는 LAA 소그룹의 경우 약 2.5-3.5 GPa이며 HAA와 TH 소그룹의 경우는 약 1.5-2.5 GPa로 추정된다(Tatsumi et al., 2005; Brenna et al., 2010, 2012). 지구물리 자료로 측정된 제주도의 지각 두께는 약 25-35 km이다(Yoo et al., 2007; Kim et al., 2015). 한편 단사휘석 지압계와 감람석의 미량원소 성분으로 추정된 제주도 현무암 마그마 방의 깊이는 약 0.7 GPa이다(Yang et al., 2012; Kim et al., 2021). 제주 회장암 단괴 및 산청·하동 회장암체 속에 정장석이 포함되어 있지 않다는 사실과 이들 회장암류는 중간 지각 깊이에 위치한다는 사실 등에 미루어 볼 때, 제주 멜트 포유물에서 관찰되는 K, Ba, Pb에서의 양의 이상과 Al2O3 부화 등은 근원 맨틀 속에 존재하는 광물의 특성으로 추정된다. 일부의 연구에서는(Yaxley et al., 2004), 멜트 포유물의 미량원소 함량의 다양성이 석영-장석이 풍부한 화강암질 지각물질의 부분용융 산물과의 혼합 과정에서 생성되었을 가능성을 제기하였다. 그러나 K, Ba, Pb, Al2O3 함량 등에서 이상이 관찰되는 대표 시료인 JNWH1-9의 경우, 화강암질 마그마와의 혼합으로 설명하기에는 SiO2 함량이 매우 낮고 MgO 함량은 상대적으로 높아서(그림 8a), 이런 가능성 역시 배제된다. 반면에 K-hollandite는 K, Ba, Pb 등에 호정적이다(Rapp et al., 2008). 따라서 K, Ba, Pb 등에서의 양의 이상과 높은 Al2O3 함량은 근원 맨틀에 K-hollandite가 포함되어 있으면 생성될 수 있는 지구화학적 특징이다(Kuritani et al., 2011). 비교를 위해 제주 시료의 Ce 대 Ce/Pb 비를 그림 10에 나타내었다. 멜트 포유물의 상당수는 해양 현무암류에 비해서 낮은 Ce/Pb 비를 가지며, 이는 K-hollandite의 특징과 부합한다(그림 10). 아울러 정장석의 Eu에 대한 분배계수는 약 1.1-4.5 (Rollinson, 1993)로 사장석(DEu = ~0.6; Rollinson, 1993) 보다도 더 호정성이 높다는 사실을 고려할 때, 시료 JNWH1-9에서 관찰되는 Eu에서의 양의 이상(그림 3b) 역시 이런 주장을 뒷받침한다. 지구물리와 탄성파 탐사 자료에 의하면, 한반도를 포함한 동아시아의 맨틀 전이대에는 고속도 이상대가 있고, 이는 정체되어 있는 태평양판으로 추정된다(e.g., Fukao et al., 2001; Huang and Zhao, 2006). K-hollandite는 대표적인 대륙기원 퇴적물로 해양판과 함께 섭입되어 맨틀 전이대에 정체하고 있다가, 추후 습윤 플룸 등에 동반되어 상승하면서 한반도 화산활동에 연루되었을 수 있다(Richard and Iwamori, 2010; Choi et al., 2017, 2020; Kuritani et al., 2019; Choi and Liu, 2022).
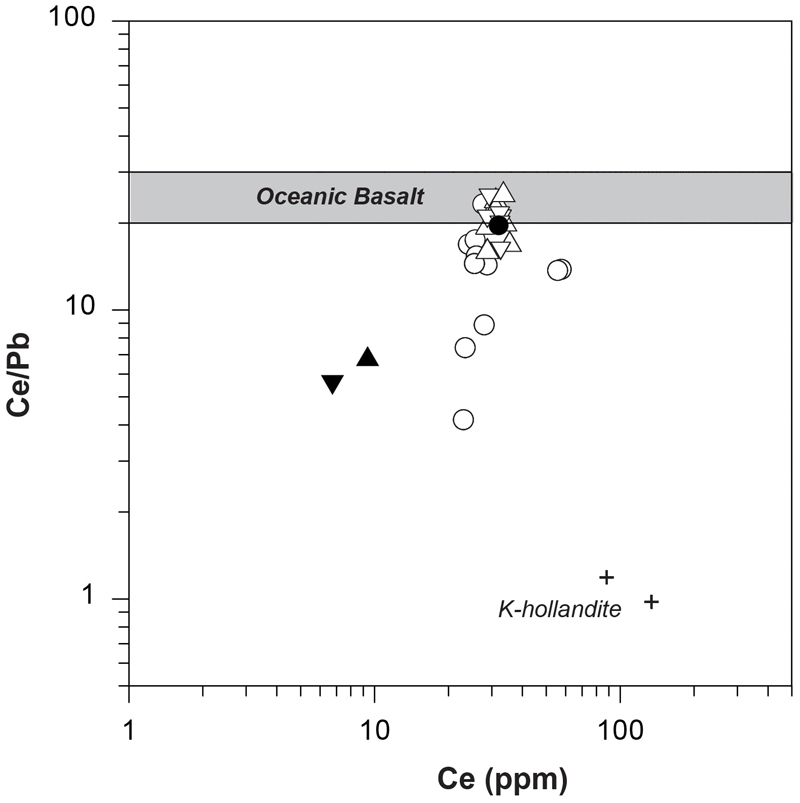
Ce/Pb vs. Ce (ppm) contents for melt inclusions in olivines and host basaltic rocks. The shaded field represents the range of oceanic basalts (Ce/Pb = 25 ± 5; Hofmann et al., 1986). Data for K-hollandite is from Rapp et al. (2008). Symbols as in Fig. 2.
상당수 멜트 포유물에서는 P에서의 양의 이상이 관찰되며(그림 4), 이는 근원 맨틀의 특성으로 간주된다. 흥미롭게도 백두산 현무암의 멜트 포유물과 전암에서도 동일한 특징이 관찰된다(Choi et al., 2017, 2020). 석류석 러어졸라이트(lherzolite)를 포함하여 지구 암석을 구성하는 광물 중 대표적인 (함)P 광물로는 인회석을 들 수 있다(e.g., Murayama et al., 1986; Konzett and Frost, 2009; Konzett et al., 2012). 인회석은 약 8 GPa 이상의 압력에서는 튜이트(tuite; γ-Ca3 (PO4)2)로 상전이한다(Murayama et al., 1986; Konzett and Frost, 2009; Konzett et al., 2012; Thompson et al., 2013). 제주 멜트 포유물에서 관찰되는 P 이상은 근원 맨틀에 (함)P 광물 즉, 인회석 또는 그 고압상이 포함되어 있음을 시사한다. 다만 백두산에 비해서 제주도 현무암류에서 관찰되는 인회석 또는 그 고압상 및 K-hollandite와 관련된 지구화학적 강도는 상대적으로 약하다. 이는 한반도 화산활동을 유발한 근원 맨틀 속에 존재하는 섭입된 퇴적물의 상대 분포량에 지역성이 있음을 나타낸다.
한편, 제주 현무암류와 멜트 포유물은 전형적인 OIB와 달리 모두 양의 값으로 분별된 (Zr/Hf)N 비를 가진다(그림 4). 단사휘석(DZr < DHf)과 석류석(DZr > DHf)의 상반된 Zr과 Hf 분배계수(D)에 기초하여 Kim et al. (2019)는 이를 섭입된 후 맨틀 전이대에 정체해 있는 해양판(예, (고-)태평양판)) 기원 에클로자이트(eclogite)가 재활성화되어 생성된 특징일 가능성을 제기한 바 있다. JNWH1-9 시료에서 관찰되는 높은 CaO와 TiO2 그리고 매우 낮은 SiO2 함량은(그림 8) (함)탄산염 에클로자이트의 활성화 가능성을 시사하며(Dasgupta et al., 2006), 이는 선행연구에서 제주 현무암이 전형적인 맨틀의 δ26Mg 값(-0.25 ± 0.04‰; Teng, 2017)과 δ66Zn 값(0.18 ± 0.06‰; Wang et al., 2017b)에 비해 매우 낮은 δ26Mg 값(-0.53‰~-0.20‰)과 높은 δ66Zn 값(0.37 ± 0.06‰)을 가진다는 사실에서 제기된 근원 맨틀 내 탄산염 물질의 존재 가능성 제안(Kim et al., 2019; Choi and Liu, 2022)과도 일치하는 결과이다.
5. 결 론
제주도(함덕리와 남원읍) 현무암류에서 감람석을 분리하여 그 속에 포획된 멜트 포유물의 주성분과 미량원소 성분을 분석한 결과 전암에 비해 지화학적 다양성이 발견되었다. 이로부터 현무암질 마그마의 근원 맨틀이 암상 또는 성분적으로 이질성이 있으며, 멜트는 분별용융 산물임이 유추된다. 알칼리계열의 멜트 포유물이 아알칼리계열의 전암 속에 포획되어 있다는 사실에서 제주도의 알칼리와 아알칼리 계열 화산암류는 유사한 근원물질이 서로 다른 정도로 부분용융되어 생성된 것으로 추정된다. 일부 멜트 포유물에서 K, Ba, Eu, Pb 또는 P에서의 양의 이상이나 Al2O3 함량의 농집이 관찰된다. 아울러 멜트 포유물은 양의 값으로 분별된 (Zr/Hf)N 비를 가지는 것이 특징이다. 이로부터 제주도 현무암류를 형성한 근원 맨틀 속에 K-hollandite, 인회석 또는 그 고압상과 (함)탄산염 에클로자이트가 존재할 가능성이 유추된다. 이들은 맨틀 전이대에 정체되어 있는 (고-)태평양판 물질에서 유래하였을 가능성이 크다. 그러나 백두산에 비해서 제주도 현무암류에서 관찰되는 이런 부화물질과 관련된 지구화학적 강도는 상대적으로 약하다. 이는 한반도 화산활동을 유발한 근원 맨틀 속에 존재하는 섭입된 퇴적물의 상대 분포량에 지역성이 있음을 나타낸다.
Acknowledgments
이 연구는 충남대학교 학술연구비에 의해 지원되었습니다. (함)감람석 현무암류 시료 채취를 위한 제주 지역 야외조사에 도움을 주신 故 송시태 박사께 깊은 감사의 마음을 전합니다. 건설적인 비평을 해주신 익명의 두 분 심사위원께 감사드립니다.
References
-
Anderson, A.T., 1976, Magma mixing: petrological process and volcanological tool. Journal of Volcanology and Geothermal Research, 1, 3-33.
[https://doi.org/10.1016/0377-0273(76)90016-0]
-
Ashwal, L.D. and Bybee, G.M., 2017, Crustal evolution and the temporality of anorthosites. Earth-Science Reviews, 173, 307-330.
[https://doi.org/10.1016/j.earscirev.2017.09.002]
-
Baek, S., Choi, S.H., Lee, S.-G., Lee, S.R. and Lee, H.M., 2014, Geochemistry of anorthositic xenolith and host tholeiite basalt from Jeju Island, South Korea. Geosciences Journal, 18, 125-135.
[https://doi.org/10.1007/s12303-013-0060-9]
-
Brenna, M., Cronin, S.J., Smith, I.E.M., Maas, R. and Sohn, Y.K., 2012, How small-volume basaltic magmatic systems develop: a case study from the Jeju Island Volcanic Field, Korea. Journal of Petrology, 53, 985-1013.
[https://doi.org/10.1093/petrology/egs007]
-
Brenna, M., Cronin, S.J., Smith, I.E.M., Sohn, Y.K. and Németh, K., 2010, Mechanisms driving polymagmatic activity at a monogenetic volcano, Udo, Jeju Island, South Korea. Contributions to Mineralogy and Petrology, 160, 931-950.
[https://doi.org/10.1007/s00410-010-0515-1]
-
Choi, H.-O., Choi, S.H., Lee, Y.S., Ryu, J.-S., Lee, D.-C., Lee, S.-G., Sohn, Y.K. and Liu, J., 2020, Petrogenesis and mantle source characteristics of the late Cenozoic Baekdusan (Changbaishan) basalts, North China Craton. Gondwana Research, 78, 156-171.
[https://doi.org/10.1016/j.gr.2019.08.004]
-
Choi, H.-O., Choi, S.H., Schiano, P., Cho, M., Cluzel, N., Devidal, J.-L. and Ha, K., 2017, Geochemistry of olivine-hosted melt inclusions in the Baekdusan (Changbaishan) basalts: Implications for recycling of oceanic crustal materials into the mantle source. Lithos, 284-285, 194-206.
[https://doi.org/10.1016/j.lithos.2017.04.006]
- Choi, S.H., 2022, Mantle source lithologies of late Cenozoic basaltic rocks and two varieties of enriched mantle in the Korean Peninsula. Korean Journal of Mineralogy and Petrology, 35, 183-197 (in Korean with English abstract).
-
Choi, S.H. and Liu, S.-A., 2022, Zinc isotopic systematics of the Mt. Baekdu and Jeju Island intraplate basalts in Korea, and implications for mantle source lithologies. Lithos, 416-417, 106659.
[https://doi.org/10.1016/j.lithos.2022.106659]
-
Choi, S.H., Mukasa, S.B., Kwon, S.-T. and Andronikov, A.V., 2006, Sr, Nd, Pb and Hf isotopic compositions of late Cenozoic alkali basalts in South Korea: evidence for mixing between the two dominant asthenospheric mantle domains beneath East Asia. Chemical Geology, 232, 134-151.
[https://doi.org/10.1016/j.chemgeo.2006.02.014]
-
Choi, S.H., Schiano, P., Chen, Y., Devidal, J.-L., Choo, M.K. and Lee, J.-I., 2013, Melt inclusions in olivine and plagioclase phenocrysts from Antarctic-Phoenix Ridge basalts: Implications for origin of N- and E-type MORB parent magmas. Journal of Volcanology and Geothermal Research, 253, 75-86.
[https://doi.org/10.1016/j.jvolgeores.2012.12.008]
-
Cottrell, E., Spiegelman, M. and Langmuir, C.H., 2002, Consequences of diffusive re-equilibration for the interpretation of melt inclusions. Geochemistry, Geophysics, Geosystems, 3, 2001 GC000205.
[https://doi.org/10.1029/2001GC000205]
-
Danyushevsky, L.V., Della-Pasqua, F.N. and Sokolov, S., 2000, Re-equilibration of melt inclusions trapped by magnesian olivine phenocrysts from subduction-related magmas: petrological implications. Contributions to Mineralogy and Petrology, 138, 68-83.
[https://doi.org/10.1007/PL00007664]
-
Danyushevsky, L.V., Leslie, R.A., Crawford, A.J. and Durance, P., 2004, Melt inclusions in primitive olivine phenocrysts: the role of localized reaction processes in the origin of anomalous compositions. Journal of Petrology, 45, 2531-2553.
[https://doi.org/10.1093/petrology/egh080]
-
Danyushevsky, L.V., McNeill, A.W. and Sobolev, A.V., 2002, Experimental and petrological studies of melt inclusions in phenocrysts from mantle-derived magmas: an overview of techniques, advantages and complications. Chemical Geology, 183, 5-24.
[https://doi.org/10.1016/S0009-2541(01)00369-2]
-
Danyushevsky, L.V. and Plechov, P., 2011, Petrolog3: integrated software for modeling crystallization processes. Geochemistry Geophysics Geosystems, 12, Q07021.
[https://doi.org/10.1029/2011GC003516]
-
Dasgupta, R., Hirschmann, M.M. and Stalker, K., 2006, Immiscible transition from carbonate-rich to silicate-rich melts in the 3 GPa melting interval of eclogite+CO2 and genesis of silicate-undersaturated ocean island lavas. Journal of Petrology, 47, 647-671.
[https://doi.org/10.1093/petrology/egi088]
-
Fukao, Y., Widiyantoro, S. and Obayashi, M., 2001, Stagnant slabs in the upper and lower mantle transition region. Reviews of Geophysics, 39, 291-323.
[https://doi.org/10.1029/1999RG000068]
-
Gagnon, J.E., Fryer, B.J., Samson, I.M. and Williams-Jones, A.E., 2008, Quantitative analysis of silicate certified reference materials by LA-ICPMS with and without an internal standard. Journal of Analytical Atomic Spectrometry, 23, 1529-1537.
[https://doi.org/10.1039/b801807n]
-
Hofmann, A.W., Jochum, K.P., Seufert, M. and White, W.M., 1986, Nb and Pb in oceanic basalts: new constraints on mantle evolution. Earth and Planetary Science Letters, 79, 33-45.
[https://doi.org/10.1016/0012-821X(86)90038-5]
-
Huang, J. and Zhao, D., 2006, High-resolution mantle tomography of China and surrounding regions. Journal of Geophysical Research, 111, B09305.
[https://doi.org/10.1029/2005JB004066]
-
Irvine, T.N. and Baragar, W.R.A., 1971, A guide to the chemical classification of the common volcanic rocks. Canadian Journal of Earth Sciences, 8, 523-548.
[https://doi.org/10.1139/e71-055]
- Jarosewich, E., Nelen, J.A. and Norberg, J.A., 1979, Electron Microprobe Reference Samples for Mineral Analyses. Smithsonian Contributions to the Early Sciences, 22, 68-72.
-
Kelemen, P.B., Shimizu, N. and Salters, V.J.M., 1995, Extraction of mid-ocean-ridge basalt from the upwelling mantle by focused flow of melt in dunite channels. Nature, 375, 747-753.
[https://doi.org/10.1038/375747a0]
-
Kendrick, M.A., Jackson, M.G., Kent, A.J.R., Hauri, E.H., Wallace, P.J. and Woodhead, J., 2014, Contrasting behaviours of CO2, S, H2O and halogens (F, Cl, Br, and I) in enriched mantle melts from Pitcairn and Society seamounts. Chemical Geology, 370, 69-81.
[https://doi.org/10.1016/j.chemgeo.2014.01.019]
-
Kent, A.J.R., 2008, Melt inclusions in basaltic and related volcanic rocks. Reviews in Mineralogy and Geochemistry, 69, 273-331.
[https://doi.org/10.2138/rmg.2008.69.8]
-
Kim, D., Lee, H., Lee, W., Kim, J., Oh, J., Song, J.-H., Jung, H. and Stuart, F.M., 2021, Helium isotopes and olivine geochemistry of basalts and mantle xenoliths in Jeju Island, South Korea: Evaluation of role of SCLM on the Cenozoic intraplate volcanism in East Asia. Lithos, 390-391, 106123.
[https://doi.org/10.1016/j.lithos.2021.106123]
-
Kim, J.I., Choi, S.H., Koh, G.W., Park, J.B. and Ryu, J.-S., 2019, Petrogenesis and mantle source characteristics of volcanic rocks on Jeju Island, South Korea. Lithos, 326-327, 476-490.
[https://doi.org/10.1016/j.lithos.2018.12.034]
-
Kim, J.I., Choi, S.H. and Lee, D.-C., 2023, Hf isotope compositions of basaltic rocks from Jeju Island, South Korea: Implications for the origin of the dichotomous isotopic distribution of late Cenozoic magmatism in East Asia. Lithos, 442-443, 107047.
[https://doi.org/10.1016/j.lithos.2023.107047]
-
Kim, S.K. and Choi, S.H., 2021, Geochemical studies on the mantle source lithologies of late Cenozoic alkali basalt from Baengnyeong, Pyeongtaek, and Asan in the Korean Peninsula. Lithos, 404-405, 106434.
[https://doi.org/10.1016/j.lithos.2021.106434]
-
Kim, Y.H., Lee, C. and Kim, S.S., 2015, Tectonics and volcanism in East Asia: insights from geophysical observations. Journal of Asian Earth Sciences, 113, 842-856.
[https://doi.org/10.1016/j.jseaes.2015.07.032]
- Kim, Y.S. and Choi, S.H., 2012, Plagioclase-rich nodules in Taeheungri basalt from Namwon, Jeju Island and its implications for tholeiite magmatism. Journal of the Geological Society of Korea, 48, 313-324 (in Korean with English abstract).
- Ko, B., 2006, Petrological and geochemical studies of the petrogenesis of the Hadong-Sancheong anorthosite complex. Ph.D. thesis, Kangwon National University, Chuncheon, 154 p (in Korean with English abstract).
- Koh, G.W., Park, J.B., Kang, B.-R., Kim, G.-P. and Moon, D.C., 2013, Volcanism in Jeju Island. Journal of the Geological Society of Korea, 49, 209-230 (in Korean with English abstract).
-
Konzett, J. and Frost, D.J., 2009, The high P-T stability of hydroxyl-apatite in natural and simplified MORB-an experimental study to 15 GPa with implications for transport and storage of phosphorus and halogens in subduction zones. Journal of Petrology, 50, 2043-2062.
[https://doi.org/10.1093/petrology/egp068]
-
Konzett, J., Rhede, D. and Frost, D.J., 2012, The high P-T stability of apatite and Cl partitioning between apatite and hydrous potassic phases in peridotite: an experimental study to 19 GPa with implications for the transport of P, Cl and K in the upper mantle. Contributions to Mineralogy and Petrology, 163, 277-296.
[https://doi.org/10.1007/s00410-011-0672-x]
-
Kuritani, T., Kimura, J.-I., Ohtani, E., Miyamoto, H. and Furuyama, K., 2013, Transition zone origin of potassic basalts from Wudalianchi volcano, Northeast China. Lithos, 156-159, 1-12.
[https://doi.org/10.1016/j.lithos.2012.10.010]
-
Kuritani, T., Ohtani, E. and Kimura, J.-I., 2011, Intensive hydration of the mantle transition zone beneath China caused by ancient slab stagnation. Nature Geosciences, 4, 713-716.
[https://doi.org/10.1038/ngeo1250]
-
Kuritani, T., Xia, Q.-K., Kimura, J.-I., Liu, J., Shimizu, K., Ushikubo, T., Zhao, D., Nakagawa, M. and Yoshimura, S., 2019, Buoyant hydrous mantle plume from the mantle transition zone. Scientific Reports, 9, 6549.
[https://doi.org/10.1038/s41598-019-43103-y]
- Lee, M.W., 1982, Petrology and geochemistry of Jeju Volcanic Island, Korea. The Science Reports of the Tohoku University, Series 3, 177-256.
-
Lee, Y., Cho, M. and Yi, K., 2017, In situ U-Pb and Lu-Hf isotopic studies of zircons from the Sancheong-Hadong AMCG suite, Yeongnam Massif, Korea: implications for the petrogenesis of ~1.86 Ga massif-type anorthosite. Journal of Asian Earth Sciences, 138, 629-646.
[https://doi.org/10.1016/j.jseaes.2017.02.038]
-
Maclennan, J., 2008a, Concurrent mixing and cooling of melts under Iceland. Journal of Petrology, 49, 1931-1953.
[https://doi.org/10.1093/petrology/egn052]
-
Maclennan, J., 2008b, Lead isotope variability in olivine-hosted melt inclusions from Iceland. Geochimica et Cosmochimica Acta, 72, 4159-4176.
[https://doi.org/10.1016/j.gca.2008.05.034]
-
Maclennan, J., McKenzie, D., Grönvold, K., Shimizu, N., Eiler, J.M. and Kitchen, N., 2003, Melt mixing and crystallization under Theistareykir, northeast Iceland. Geochemistry Geophysics Geosystems, 4, 8624.
[https://doi.org/10.1029/2003GC000558]
-
Mathez, E.A., 1976, Sulfur solubility and magmatic sulfides in submarine basalt glass. Journal of Geophysical Research, 81, 4269-4276.
[https://doi.org/10.1029/JB081i023p04269]
-
Murayama, J.K., Nakai, S., Kato, M. and Kumazawa, M., 1986, A dense polymorph of Ca3(PO4)2: a high pressure phase of apatite decomposition and its geochemical significance. Physics of the Earth and Planetary Interiors, 44, 293-303.
[https://doi.org/10.1016/0031-9201(86)90057-9]
- Park, J.-B. and Kwon, S.-T., 1993, Geochemical evolution of the Cheju volcanic island: Petrography and major element chemistry for stratigraphically-controlled lavas from the northern part of Cheju Island. Journal of the Geological Society of Korea, 29, 39-60 (in Korean with English abstract).
-
Rapp, R.P., Irifune, T., Shimizu, N., Nishiyama, N., Norman, M.D. and Inoue, T., 2008, Subduction recycling of continental sediments and the origin of geochemically enriched reservoirs in the deep mantle. Earth and Planetary Science Letters, 271, 14-23.
[https://doi.org/10.1016/j.epsl.2008.02.028]
-
Richard, G.C. and Iwamori, H., 2010, Stagnant slab, wet plumes and Cenozoic volcanism in East Asia. Physics of the Earth and Planetary Interiors, 183, 280-287.
[https://doi.org/10.1016/j.pepi.2010.02.009]
-
Roedder, E., 1984, Fluid inclusions. Review in Mineralogy, Mineralogical Society of America, 12, 646 p.
[https://doi.org/10.1515/9781501508271]
-
Roeder, P.L. and Emslie, R.F., 1970, Olivine-liquid equilibrium. Contributions to Mineralogy and Petrology, 29, 275-289.
[https://doi.org/10.1007/BF00371276]
- Rollinson, H.R., 1993, Using Geochemical Data: Evaluation, Presentation, Interpretation. Longman Scientific & Technical, Essex, p. 352.
-
Saal, A.E., Hart, S.R., Shimizu, N., Hauri, E.H. and Layne, G.D., 1998, Pb isotopic variability in melt inclusions from oceanic island basalts, Polynesia. Science, 282, 1481-1484.
[https://doi.org/10.1126/science.282.5393.1481]
-
Schiano, P., 2003, Primitive mantle magmas recorded as silicate melt inclusions in igneous minerals. Earth-Science Reviews, 63, 121-144.
[https://doi.org/10.1016/S0012-8252(03)00034-5]
-
Schmidt, M.W., 1996, Experimental constraints on recycling of potassium from subducted oceanic crust. Science, 272, 1927-1930.
[https://doi.org/10.1126/science.272.5270.1927]
-
Sobolev, A.V. and Danyushevsky, L.V., 1994, Petrology and geochemistry of boninites from the North Termination of the Tonga Trench: constraints on the generation conditions of primary high-Ca boninite magmas. Journal of Petrology, 35, 1183-1211.
[https://doi.org/10.1093/petrology/35.5.1183]
- Sobolev, A.V., Dmitriev, L.V., Barsukov, V.L., Nevsorov, V.N. and Slutsky, A.B., 1980, The formation conditions of the high-magnesium olivines from the monomineralic fraction of Luna 24 regolith. Lunar and Planetary Science Conference Proceedings, Pergamon Press, New York, USA, 11, 105-116.
-
Sobolev, A.V. and Shimizu, N., 1993, Ultra-depleted primary melt included in an olivine from the Mid-Atlantic Ridge. Nature, 363, 151-154.
[https://doi.org/10.1038/363151a0]
-
Spandler, C., O’Neill, H.St.C. and Kamenetsky, V.S., 2007, Survival times of anomalous melt inclusions from element diffusion in olivine and chromite. Nature, 447, 303-306.
[https://doi.org/10.1038/nature05759]
-
Stolper, E., Sherman, S., Garcia, M., Baker, M. and Seaman, C., 2004, Glass in the submarine section of the HSDP2 drill core, Hilo, Hawaii. Geochemistry, Geophysics, Geosystems, 5, Q07G15.
[https://doi.org/10.1029/2003GC000553]
-
Sun, S.-S. and McDonough, W.F., 1989, Chemical and isotopic systematics of oceanic basalts: implications for mantle composition and processes. In: Saunders, A.D. and Norry, M.J. (Eds.), Magmatism in the Ocean Basins. Geological Society Special Publications, 42, 313-345.
[https://doi.org/10.1144/GSL.SP.1989.042.01.19]
-
Tatsumi, Y., Shukuno, H., Yoshikawa, M., Chang, Q., Stato, K. and Lee, M.W., 2005, The petrology and geochemistry of volcanic rocks on Jeju Island: Plume magmatism along the Asian continental margin. Journal of Petrology, 46, 523-553.
[https://doi.org/10.1093/petrology/egh087]
-
Teng, F.-Z., 2017, Magnesium isotope geochemistry. Reviews in Mineralogy and Geochemistry, 82, 219-287.
[https://doi.org/10.2138/rmg.2017.82.7]
-
Thompson, R.M., Xie, Z., Zhai, S., Downs, R.T. and Yang, H., 2013, A comparison of the Ca3(PO4)2 and CaSiO3 systems, with a new structure refinement of tuite synthesized at 15 GPa and 1300℃. American Mineralogist, 98, 1585-1592.
[https://doi.org/10.2138/am.2013.4435]
-
Thompson, R.N. and Gibson, S.A., 2000, Transient high temperatures in mantle plume heads inferred from magnesian olivines in Phanerozoic picrites. Nature, 407, 502-506.
[https://doi.org/10.1038/35035058]
-
Thornber, C.R., Sherrod, D.R., Siems, D.F., Heliker, C.C., Meeker, G.P., Oscarson, R.L. and Kauahikaua, J.P., 2002, Whole-rock and glass major-element geochemistry of Kilauea Volcano, Hawaii, near-vent eruptive products: September 1994 through September 2001 (RPRT). Open-File Report, US Geological Survey.
[https://doi.org/10.3133/ofr0217]
-
Wallace, P.J., Plank, T., Bodnar, R.J., Gaetani, G.A. and Shea, T., 2021, Olivine-hosted melt inclusions: a microscopic perspective on a complex magmatic world. Annual Review of Earth and Planetary Sciences, 49, 465-494.
[https://doi.org/10.1146/annurev-earth-082420-060506]
-
Wang, X.-J., Chen, L.-H., Hofmann, A.W., Mao, F.-G., Liu, J.-Q., Zhong, Y., Xie, L.-W. and Yang, Y.-H., 2017a, Mantle transition zone-derived EM1 component beneath NE China: geochemical evidence from Cenozoic basalts. Earth and Planetary Science Letters, 465, 16-28.
[https://doi.org/10.1016/j.epsl.2017.02.028]
-
Wang, Z.-Z., Liu, S.-A., Liu, J., Huang, J., Xiao, Y., Chu, Z.-Y., Zhao, X.-M. and Tang, L., 2017b, Zinc isotope fractionation during mantle melting and constraints on the Zn isotope composition of Earth’s upper mantle. Geochimica et Cosmochimica Acta, 198, 151-167.
[https://doi.org/10.1016/j.gca.2016.11.014]
-
Yang, K., Arai, S., Yu, J.-E., Yun, S.-H., Kim, J.-S. and Hwang, J.-Y., 2012, Gabbroic xenoliths and megacrysts in the Pleisto-Holocene alkali basalts from Jeju Island, South Korea: The implications for metasomatism of the lower continental crust. Lithos, 142-143, 201-215.
[https://doi.org/10.1016/j.lithos.2012.03.006]
-
Yaxley, G.M., Kamenetsky, V.S., Kamenetsky, M., Norman, M.D. and Francis, D., 2004, Origins of compositional heterogeneity in olivine-hosted melt inclusions from the Baffin Island picrites. Contributions to Mineralogy and Petrology, 148, 426-442.
[https://doi.org/10.1007/s00410-004-0613-z]
-
Yi, W., Halliday, A.N., Alt, J.C., Lee, D.-C., Rehkämper, M., Garcia, M.O., Langmuir, C.H. and Su, Y., 2000, Cadmium, indium, tin, tellurium, and sulfur in oceanic basalts: implications for chalcophile element fractionation in the Earth. Journal of Geophysical Research: Solid Earth, 105, 18927-18948.
[https://doi.org/10.1029/2000JB900152]
-
Yoo, H.J., Herrmann, R.B., Cho, K.H. and Kee, K., 2007, Imaging the three-dimensional crust of the Korean Peninsula by joint inversion of surface-wave dispersion and teleseismic receiver functions. Bulletin of the Seismological Society of America, 97, 1002-1011.
[https://doi.org/10.1785/0120060134]