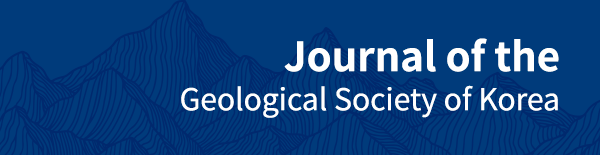
지진발생시 사면 변위 예측을 통한 부산시 산사태 재해평가
초록
지진이 발생함에 따라 지진동, 액상화, 산사태, 지표파열 등과 같은 다양한 유형의 지진재해가 발생한다. 이번 연구는 최근 중규모 이상의 지진이 발생한 한반도 동남부 중 양산단층계 곡부에 도심지가 위치하고 급사면이 발달한 부산광역시를 대상으로 지진성 산사태 재해평가를 실시하였다. 평가 방법은 실제 지진발생시 산사태 사례와 가장 잘 부합한 것으로 알려져 있는 Newmark 변위 모델을 이용하였다. 해석 결과, 연구지역 중북부의 금정산과 백양산, 승학산, 철마면 인근 사면에서 산사태 발생 가능성이 ‘매우 높음’ 단계로 나타났다. 이번 연구에서 사용된 포화도, 점착력, 단위중량, 토층두께 등과 같은 사면의 공학적 특성은 선행연구를 참고하거나 가정 및 추정을 통하여 결정하였기 때문에 불확실성을 내포하고 있을 것으로 판단된다. 향후, 정확한 지질·지반조사자료의 확보 후 지진성 산사태 재해평가가 이루어진다면 방재대책 수립에 유용하게 활용될 것으로 기대된다.
Abstract
Earthquakes cause various seismic hazards such as ground motion, liquefaction, landslides, and surface rupture. We focused on potential seismic landslide hazard assessment in Busan Metropolitan City, Korea. The study area is the largest city close to the epicenter of a recent series of mid-scale earthquakes (Gyeongju and Pohang earthquakes). Three faults of the Yangsan fault system pass through the city, and there are many steep slopes in the city. We chose the Newmark displacement model for estimating, which is known to best match the earthquake-induced landslides. The results reveal that the ‘very high’ grade areas are concentrated in Mt. Geumjeong, Mt. Baekyang, Mt. Seunghak, and the slopes of Cheolma-myeon in the north-central part of the study area. We used parameters such as saturation, cohesion, unit weight, and soil thickness in the Newmark model based on reference and estimation. Therefore the parameters have estimation uncertainties and spatial variability, and the results imply uncertainty. Accurate geological and geotechnical data are required for reliable seismic landslide assessment, which can be useful for mitigating the risk of seismic landslides and for policy.
Keywords:
seismic landslide, Newmark displacement model, seismic hazard, hazard assessment키워드:
지진성 산사태, Newmark 변위 모델, 지진재해, 재해평가1. 서 론
산사태를 발생시키는 가장 큰 요인으로 강우와 지진, 강설, 화산을 들 수 있다. 지진에 의한 산사태는 중국의 쓰촨성, 대만의 가오슝, 일본의 오이타, 인도네시아의 롬복 등 세계 각국의 도시에서 발생했으며, 토석류(debris flow), 땅밀림 산사태(creep), 산사태(landslide) 등과 같은 형태로 보고되고 있다(Huang et al., 2002; Wang et al., 2014; Zhang et al., 2014; Chen et al., 2017a, 2017b; Fan et al., 2017; Ferrario, 2019). 이러한 지진성 산사태는 대규모의 인명피해와 재산피해를 동반하기 때문에 그 피해를 줄이기 위하여 지진발생시 사면의 동적 변위 연구(Jibson et al., 2000), 수치적 모델을 이용한 지진성 산사태 연구(Dreyfus et al., 2013), 지진성 산사태의 취약 지형 특성 정량적 분석(Harp et al., 2011; Guo and Hamada, 2013), 지진성 산사태 붕괴 메커니즘(Huang et al., 2012) 등 여러 연구자에 의해 다양한 관점에서 연구가 수행되고 있다.
한반도에서 지진으로 인한 산사태 역사기록은 9건으로, 특히 1643년과 1810년에 발생한 지진성 산사태는 사상자와 큰 재산피해를 발생시켰다. 1643년 경상남도 합천군에서 최대진도 Ⅷ~IX로 추정되는 지진으로 인해 산사태가 발생해 압사자가 2명 발생하였으며, 1810년에는 함경북도 부령에서 최대진도 Ⅷ~IX로 추정되는 지진으로 주변 가옥 83채가 무너지고 그로 인해 사람과 가축이 압사하였다(Lee et al., 2013; Seo et al., 2019). 계기지진기록에서는 2017년 포항지진(ML 5.4)에 의한 6.5 cm의 땅밀림(Um, 2019)과 2016년 경주지진(ML 5.8)에 의한 석굴암 진입로 대형낙석(Kim and Lee, 2019)이 보고된 바 있다. 이렇듯 역사지진과 계기지진의 기록으로 미루어보아 우리나라 또한 지진으로 인한 산사태 발생 가능성을 배제할 수 없으며, 현재 규모 5.0 이상의 지진 발생빈도가 꾸준히 증가하고 있어 지진 발생을 대비한 산사태 재해평가가 요구된다.
연구지역인 부산광역시는 국내 대도시 중 산악지형이 많고 해안에 접해 있어 자연재해에 많은 취약점을 가지고 있다. 산지 비율이 높고 인구가 밀집되어 있어 주택난 및 교통난 해소를 위해 무분별한 개발을 진행하였고, 그로 인한 산사태 피해가 극심한 편이다. 산림청에서 조사한 최근 10년간(2011년~2021년) 산사태 발생 현황에 따르면 광역시 단위에서 연구지역이 96 ha로 가장 넓은 면적의 피해를 보았으며(Sansatai Information System, 2012), 실제 부산의 많은 사면에는 산사태 방지를 위한 사방시설이 곳곳에 설치되어 있다. 국내 산사태의 주된 요인은 강우로 알려져 있는데(Chae et al., 2016), 이러한 통계 결과는 연구지역이 국내에서 산사태 취약성이 가장 높음을 의미한다. 따라서, 집중호우와 지진이 동시에 발생하는 경우 심각한 재난이 예상된다. 특히 계기지진 기록에 의하면 연구지역 중북부의 금정산에서 2회의 지진이 발생하였고, 역사문헌에서도 진도 Ⅴ이상의 지진이 다수 기록되어 있어(NIMS, 2012) 강우와 지진이 동시에 발생하는 경우를 배제할 수 없다.
이번 연구는 부산광역시를 대상으로 지진으로 인한 산사태 재해평가 방법 중 현재까지 가장 널리 이용되고 있는 방법인 Newmark 변위 모델(Newmark, 1965)을 사용하고 국내 지진 및 지질 특성을 반영하여 재해를 분석하고자 한다. Newmark 변위 모델은 비교적 명확한 사면의 특성 정보를 이용하여 지진발생시 사면의 변위를 지도상에 나타낼 수 있는 장점이 있다. 이러한 장점 때문에 국내에서는 서울지역(Jeon et al., 2014; Lee et al., 2014), 울산지역(Cho et al., 2003)에서 Newmark 변위 모델을 통한 연구가 수행된 바 있다. 하지만, 이들 연구는 일부 사면을 대상으로 수행되었으며 시·군·구 단위에서의 재해평가가 이루어지지 않아 시 차원에서의 효율적인 방재대책을 제시하기에는 무리가 있었다. 따라서 이번 연구는 국내에서 산사태 취약성이 가장 높은 부산광역시를 전역을 대상으로 지진발생시 예상되는 산사태 재해를 분석하고, 취약지역에 대해 향후 지진방재대책 마련에 기여하고자 한다.
2. 분석 기법
Newmark 변위 모델은 지진발생시 사면파괴가 예상되는 파괴토체(sliding mass)를 하나의 활동강성블록(sliding rigid-block)으로 가정하여 사면의 변위를 산정하는 방법이다(그림 1a와 1b). 활동강성블록이 움직이기 시작하는 가속도(g)를 항복가속도(critical acceleration, ac)라고 할 때, 지진발생시 활동강성블록에 작용하는 가속도(a(t), g)가 항복가속도보다 크면 블록이 사면을 따라 미끌림이 발생한다(Newmark, 1965; Jibson et al., 2000)(그림 1b와 1c). 이때, 사면과 활동강성블록은 서로 상대적인 속도차이를 가지는데, 가속도가 항복가속도 이하로 떨어져도 속도가 0이 될 때까지 거동이 지속된다. 이후 가속도가 다시 항복가속도를 초과하기 시작하면 활동강성블록의 멈춤과 활동이 반복된다. 여기서 가속도가 항복가속도를 초과하는 구간에 대해 이중적분으로 변위를 구할 수 있다(Seed et al., 1978)(그림 1c). 이후 Wilson and Keefer (1983), Ambraseys and Menu (1988), Jibson and Keefer (1993), Xu et al. (2012) 등에 의해 실제 지진성 산사태 기록과 Newmark 변위 모델을 기초로 한 경험식이 꾸준히 개발되어왔다. 이 중 Ambraseys and Menu (1988)은 지진발생시 사면 변위에 영향을 주는 다양한 인자들을 분석하였고, 그 결과 가속도비(ac/PGA)가 가장 큰 영향을 주는 인자임을 도출하였다. 이를 반영한 변위 제안식이 식 1이며, 이번 연구에서는 다른 경험식에 비해 부가가정과 입력인자가 적게 들어간 이 식을 이용하였다.
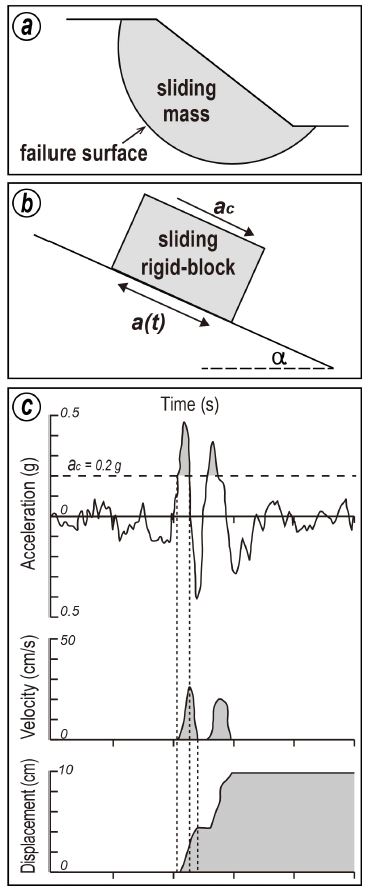
Newmark displacement analysis approach. (a) Sliding mass representation of actual slope during an earthquake. (b) Sliding rigid-block analysis concept in the Newmark displacement model during the earthquake (Kramer, 1996). (c) Development of displacement for earthquake ground motion by critical acceleration (Wilson and Keefer, 1983).
(1) |
여기서 DN은 변위(mm), PGA (peak ground acceleration)는 최대지반가속도(g), t는 변위의 오차범위를 의미한다. Newmark 변위를 산정하기 위한 항복가속도는 식 2로 표현된다(Newmark, 1965). 항복가속도는 분석 내내 일정하게 유지되며, 가속도가 항복가속도보다 작은 경우 변위가 발생하지 않는다고 가정한다. 이때 적용되는 사면의 정적안전율(Factor of safety, FS)은 사면을 무한사면으로 가정하여 계산되며 식 3으로 구할 수 있다.
(2) |
(3) |
여기서 α는 사면의 경사각(°), c는 토질의 점착력(kg/cm2), γ는 토체의 단위중량(kN/m3), γω는 물의 단위중량(kN/m3), h는 토심(m), S는 포화도(%), Φ는 내부마찰각(°)을 의미한다.
3. 해석 조건
3.1 연구지역의 지형 및 지질
연구지역인 부산광역시는 최근 발생한 경주와 포항시에서 발생한 규모 5.0 이상의 중규모 지진의 진앙지와 지리적으로 인접하며, 지형학적으로 태백산맥 말단부에 위치하여(Kim et al., 2004) 국내 대도시 중 산악지형이 발달하고 있는 곳 중 하나이다(그림 2a). 연구지역의 동부는 대운산-달음산-장산이 자리하고, 중부는 금정산-백양산-승학산이 연결된다. 부산에 발달한 사면은 해발 고도 400 m에서 800 m의 고산에서 비교적 급사면을 이루고 있다. 이러한 산악지형을 제외한 곡저부에는 활성단층으로 평가되는 양산단층계의 양산단층, 동래단층, 일광단층이 관통하고 있으며, 이들 단층과 산악지형은 모두 북북동 방향으로 발달하고 있다(그림 2b).
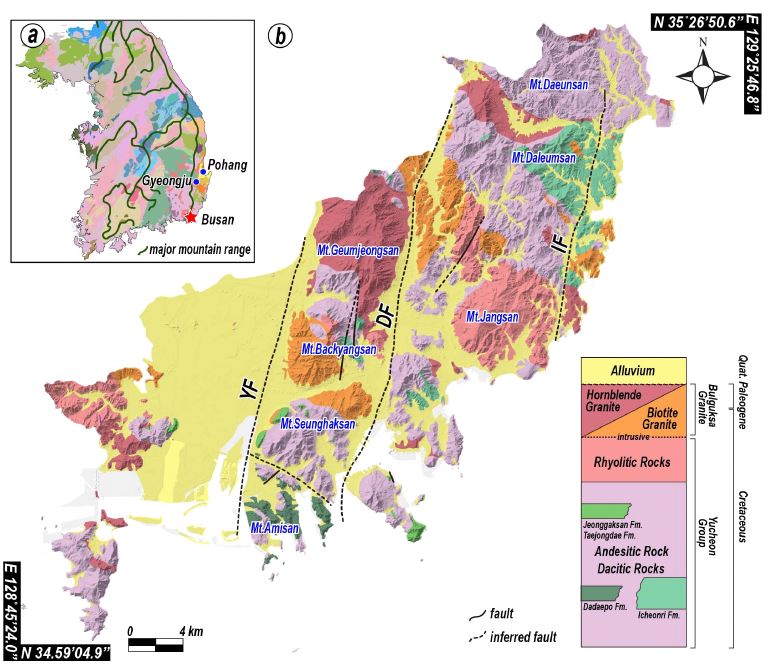
(a) Location of Busan with major mountain ranges in South Korea. (b) Simplified geological map with major mountains in Busan (modified from Lee and Kang, 1964; Lee and Kim, 1964; Park and Yoon, 1968; Son et al., 1978; Chang et al., 1983; Cho et al., 2011). YF: Yangsan Fault, DF: Dongnae Fault, IF: Ilgwang Fault.
연구지역의 단층곡부를 따라 제4기 충적층이 분포하고, 산악지형은 백악기 퇴적암과 화산암류, 이들을 관입한 불국사화강암류와 맥암류로 이루어져 있다. 유천층군 이천리층은 연구지역의 북동부 달음산 일대에 넓게 자리하고, 다대포층과 태종대층은 연구지역의 남부인 아미산과 그 주변 반도에 나타난다. 안산암질 및 데사이트질 화산암류는 연구지역의 산악지형에 고루 분포하는 양상을 보이며, 유문암질 화산암류는 장산 인근에 가장 많이 분포한다. 불국사화강암류는 주로 금정산, 백양산, 대운산과 달음산 사이 지역 일원에서 백악기 화산암류와 접하여 발달한다(Lee and Kang, 1964; Lee and Kim, 1964; Park and Yoon, 1968; Son et al., 1978; Chang et al., 1983; Cho et al., 2011).
3.2 입력인자 선정 및 산정
지진이 발생했을 때 일어나는 사면 변위는 그 지역의 지질, 토질, 사면, 지진 특성에 의해 결정되며(Wilson and Keefer, 1983), 구축 가능한 데이터베이스와 연구지역의 특징을 고려한 Newmark 변위 분석 체계는 그림 3과 같다.

Flowchart of seismic landslide hazard assessment based on the prediction of the Newmark displacement.
사면의 정적안전율(식 3)과 항복가속도(식 2) 산정을 위하여 지질도, 지형도, 현장 실험 정보를 이용하였다. 토체는 암반의 물리적, 화학적 풍화작용, 용해작용 등에 의하여 미세한 조각들로 분리되어 형성된 것으로, 일반적으로 해당 지역에 분포하는 지질과 매우 밀접한 관련이 있다(Hatchinson, 1988). 따라서, Kim (2007)의 부산지역 자연사면의 지질조건에 따른 토질특성의 현장 실험 결과를 이용하였다. 사면을 대상으로 하였기 때문에 제4기 충적층을 제외하였다. 연구지역의 지질을 크게 퇴적암류를 그룹 Ⅰ, 화산암류를 그룹 Ⅱ, 화강암류를 그룹 Ⅲ로 분류하였으며, 공학적 특성과 물성을 1:50,000 지질도에 속성데이터로 부여하였다(그림 4a; 표 1). 점착력과 내부마찰각은 그룹 Ⅰ에서 가장 크고 그룹 Ⅱ와 그룹 Ⅲ 순으로 작게 나타나는 경향을 보인다. 비중(GS)은 그룹별로 거의 유사한 것으로 나타났다. 사면의 정적안전율(식 3) 산정에 필요한 토체의 단위중량은 식 4와 같이 비중과 간극비(e)의 관계로 정의할 수 있다.
(4) |
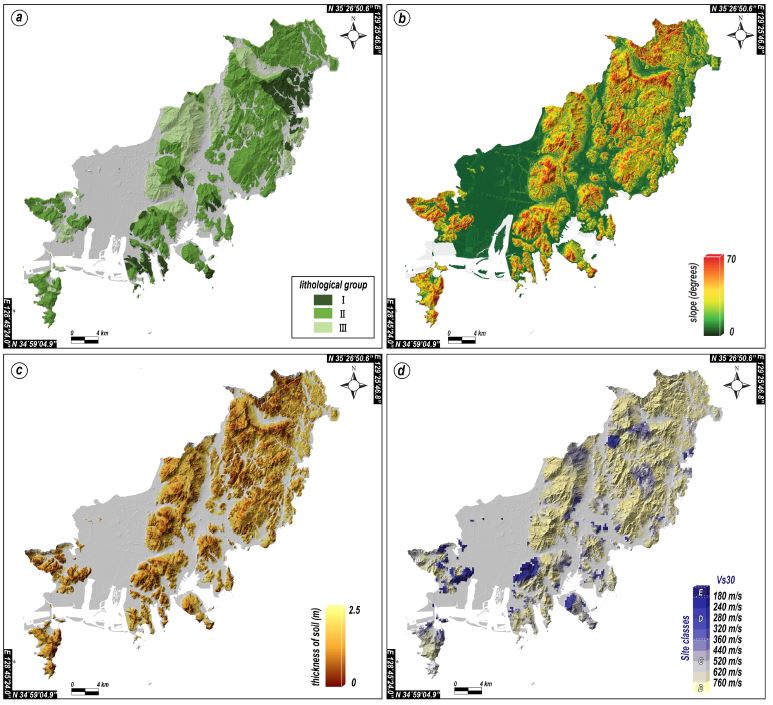
Maps showing (a) lithological groups for soil characteristics, (b) distribution of the slope angles, (c) thicknesses of soil and (d) site classification.

Soil characteristics according to the lithological group of natural slopes in study area proposed by Kim (2007).
1:5,000 수치지형도를 이용하여 DEM (digital el-evation model) 구축 후, 경사도를 분석하였다(그림 4b; 표 2). 부산광역시 전체의 경사도 분석에서는 사면 경사각이 0-10°가 50% 이상을 차지하는데 이는 연구지역의 서편 낙동강 삼각주에 평야가 넓게 형성되어 있기 때문이다. 속성이 부여된 지질도와 동일한 영역으로 분석한 결과, 20-30°의 사면이 33%로 가장 넓은 영역으로 나타났다. 식 3에서의 토심은 기반암과 토층의 경계를 직접 현장에서 조사하는 방법이 가장 유용하나, 사면의 임상, 하천, 계곡 등의 지형적 요인과 시간적 요인, 비용적 요인과 같은 제약이 따른다. 또한, 기존 국립농업과학원의 토심 자료는 3개의 공간도형에 각각의 토심 정보가 범위(예, 20-50 cm, 50-100 cm)로 제공되기 때문에 사용이 불가능하다. 한편, 많은 연구 사례에서는 토심을 토층의 두께로 가정하여 사면의 안전율을 결정해왔으며, 토심 추정모형을 이용하여 토층의 깊이를 추정하기도 했다. 따라서 이번 연구에서는 경사도와 토심의 상관관계를 통하여 토심을 예측하였으며, 상관관계가 국내와 잘 부합하는 것으로 알려져있는 USGS의 제안식(식 5)을 적용하였다(Min et al., 2005; 그림 4c). 이때, 토심의 범위는 0에서 2.5 m이며, 경사각이 70° 이상의 값에서는 토체가 존재하지 않는다고 가정한다.
(5) |
지반증폭계수는 연구지역의 시추정보를 활용하여 전단파속도(shear velocity, VS)로 지반을 분류한 Lim et al. (2020)의 연구결과를 이용하였다(그림 4d; 표 3). 사면 토심의 최댓값이 2.5 m로 매우 얕기 때문에 중장주기의 지진파보다 단주기의 지진파의 영향이 크다고 판단하여, 지반증폭계수는 단주기 증폭계수(Fa)를 이용하였다. 지반분류 결과 또한 속성이 부여된 지질도와 동일한 영역으로 분석하였으며 그 결과, 지반 B (Rock)가 53%로 가장 넓은 영역을 차지하였고 지반 C1 (weathered rock)이 21%, 지반 C2 (shallow stiff soil)가 11% 순으로 나타나 사면의 특징을 잘 반영한다.
4. 지진발생시 사면 변위 예측 결과
지질조건에 따른 토질특성, 경사, 토층두께 등의 정보를 이용하여 항복가속도를 도출하였다(그림 5a). 항복가속도는 지진발생시 지반의 움직임과는 무관하며, 사면의 정적안전율과 경사각으로 계산되기 때문에 연구지역의 산사태 취약성을 나타낸다. 항복가속도가 작은 값을 가질수록 산사태 취약성 또한 높음을 의미한다. 분석 결과, 연구지역의 중북부 금정산의 남동쪽 사면과 백양산의 남서쪽 사면, 달음산과 장산 사이의 철마면 인근 사면에서 항복가속도가 상대적으로 작은 값을 보인다. 이들 사면은 공통적으로 경사각이 크고 그룹 Ⅲ이 분포하는 곳과 일치한다. 최대지반가속도 결정에 있어 국내 내진설계기준(MOCT, 2018)에서 제시된 2,400년의 0.220 g을 기반암 가속도로 설정하고, 지반증폭계수를 통하여 최대지반가속도를 산출하였다(그림 5b). 지반 D (deep stiff soil)가 분포한 동래단층 인근 사면과 승학산의 북서부, 달음산 중부, 철마면 인근 사면에서 최대지반가속도가 높게 나타난다. 항복가속도와 최대지반가속도를 식 1에 입력하여 Newmark 변위를 산출하였다. 도출된 Newmark 변위를 Jibson and Micheal (2009)가 제안한 4개의 등급으로 나누어 그림 6과 같이 도시하였으며, Newmark 변위와 산사태 발생 가능성에 대한 재해등급 설명은 다음과 같다.
- - 150 mm 이상: 산사태 발생가능성 32% 이상으로 ‘매우 높음(very high)’ 단계
- - 50-150 mm: 산사태 발생가능성 15-32%로 ‘높음(high)’ 단계
- - 10-50 mm: 산사태 발생가능성 2-15%로 ‘보통(moderate)’ 단계
- - 0-10 mm: 산사태 발생가능성 0-2%로 ‘낮음(low)’ 단계
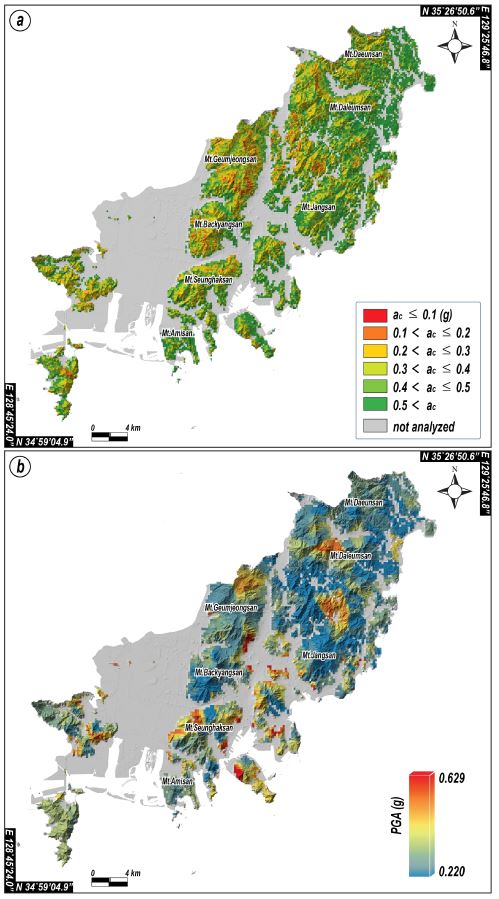
Maps showing susceptibility to seismically triggered landslides in Busan. Susceptibility is portrayed in terms of (a) critical acceleration (ac). The seismic scenario is portrayed in terms of (b) peak ground motion (PGA).
금정산의 동쪽 사면과 승학산 철마면 인근 사면에서 Newmark 변위가 150 mm 이상의 값을 보이며 산사태 발생 가능성이 ‘매우 높음’이 집중적으로 나타난다. 또한 백양산의 남쪽 사면과 가덕도의 동쪽 사면 일부에서도 Newmark 변위가 150 mm 이상을 보이기도 한다. 이는 앞서 설명한 낮은 항복가속도를 가지는 사면과 높은 최대지반가속도를 가지는 사면과 일치하는 곳으로 해석할 수 있다. 연구지역 중부의 금정산, 백양산, 승학산과 연구지역 동부의 달음산 일원의 경우 Newmark 변위가 10 mm 이상 값을 가지며, 산사태 발생 가능성이 ‘보통’을 보인다. 연구지역 동부의 사면은 대부분이 Newmark 변위가 10 mm 이하의 값을 가지며 산사태 발생가능성 ‘낮음’ 단계를 나타낸다.
5. 토의 및 결론
Newmark 변위 모델 분석에는 토질특성 정보, 최대지반가속도 도출을 위한 지진정보, 지반정보와 같은 많은 입력인자와 지질도, 수치지형도, 시추정보와 같은 공간정보가 이용되었다. 이번 연구에서는 이러한 입력인자에 대해 가정 및 추정치를 이용하거나 선행 연구결과를 채택하였고, 기존에 구축되어있는 공간데이터를 활용했다. 그러나 입력인자의 불확실성과 기구축된 데이터 활용에서의 공간적 변동성에 대한 고려가 요구된다(Refice and Capolongob, 2002; Dreyfus et al., 2013). 예를 들어 사면의 정적안전율 산정 시, 지질조건에 따른 토질특성을 크게 세 개의 그룹으로 나누었다. 연구지역의 지질은 그림 2b와 같이 다양한 암상으로 이루어져 있고, 암상의 광물과 암석학적인 특성을 고려했을 때 토질특성에 따른 그룹을 더욱 세분화할 수 있을 것으로 판단된다. 또한 속성데이터를 부여한 지질도는 1:50,000의 지질도폭 4매가 이용되었는데, 서로 인접한 지질도폭의 경계에서는 암석의 종류와 경계가 불일치하거나 암상 및 지질시대 해석이 달라지는 경우가 있다(Lim et al., 2022). 토심 추정에 사용된 USGS 토심 추정식(식 5)의 경우 미국 서부의 사면을 대상으로 도출되었는데, 우리나라의 경우 미국 서부보다 기반암 깊이가 얕고(Sun, 2010), 국립농업과학원에서 제공하는 토심 자료와 차이가 있어 향후 이에 대한 고려가 필요하다. 또한 이번 연구에서 최대지반가속도 도출을 위하여 지반분류에 따른 지반증폭계수를 이용하였다. 그러나 사면의 기하학적 특성을 고려한다면 사면의 지반증폭계수는 수평지반의 지반증폭계수보다 다소 작아질 거나(Park et al., 2014) 커질 수도 있기 때문에(Yin et al., 2021), 국내 사면의 기하와 지진파를 고려한 지반증폭 특성의 추가적인 연구가 요구된다. 이와 같이 입력인자의 가정 및 추정치를 이용했을 때 생기는 불확실성과 기존에 구축된 공간정보의 변동으로 인해 사면 변위 예측의 결과가 어느 정도 달라질 것으로 예상된다.
본 연구에서는 Newmark 변위 모델을 이용하여 지진발생시 부산광역시의 사면 변위 예측을 통한 산사태 발생 가능성을 평가하였다. 분석 결과, 항복가속도가 낮은 사면과 최대지반가속도가 높은 금정산, 승학산 일대에서 Newmark 변위가 150 mm 이상을 보이며 산사태 발생 가능성이 매우 높게 나타났다. 하지만 토심 두께, 포화도, 지질에 따른 토질특성, 사면의 지반증폭계수 등과 같은 정보에 대해서는 가정 및 추정을 통하여 반영된 결과이다. 따라서 향후 정확한 사면 특성 조사와 국내 사면에 적용 가능한 연구를 수정·보완한다면 지진발생전 산사태 예방, 피해지역 예측 및 복구 우선순위 선정 등 산사태 피해저감 대책에 보다 유용하게 활용될 것으로 기대된다.
Acknowledgments
이 연구는 행정안전부 지진 위험분석 및 관리기술 개발사업(2022-MOIS62-001)과 지진방재 전문인력 양성사업 지원으로 수행되었습니다. 심사과정에서 세심하고 건설적인 조언과 비평을 해주신 익명의 심사위원님들께 감사드립니다.
References
-
Ambraseys, N.N. and Menu, J.M., 1988, Earthquake-induced ground displacements. Soil Dynamics and Earthquake Engineering, 16, 985-1006.
[https://doi.org/10.1002/eqe.4290160704]
-
Chae, B.G., Choi, J. and Jeong, H.K., 2016, A Feasibility Study of a Rainfall Triggeirng Index Model to Warn Landslides in Korea. The Journal of Engineering Geology, 26, 235-250 (in Korean with English abstract).
[https://doi.org/10.9720/kseg.2016.2.235]
- Chang, T.W., Kang, P.C., Park, S.W., Hwang, S.K. and Lee, D.W., 1983, Geological report of the Busan-Gadeok sheets (1:50,000). Korea Institute of Energy and Resources (in Korean with English abstract).
-
Chen, C.W., Chen, H., Wei, L.W., Lin, G.W., Iida, T. and Yamada, R., 2017a, Evaluating the susceptibility of landslide landforms in Japan using slope stability analysis: a case study of the 2016 Kumamoto earthquake. Landslides, 14, 1793-1801.
[https://doi.org/10.1007/s10346-017-0872-1]
-
Chen, W., Xie, X., Wang, J., Pradhan, B., Hong, H., Bui, D.T., Duan, Z. and Ma, J., 2017b, A comparative study of logistic model tree, random forest, and classification and regression tree models for spatial prediction of landslide susceptibility. Catena, 151, 147-160.
[https://doi.org/10.1016/j.catena.2016.11.032]
- Cho, H., Kim, J.S., Son, M., Sohn, Y.K. and Kim, I.S., 2011, Petrography and 40Ar/39Ar ages of volcanic rocks in the Cretaceous Dadaepo Basin, Busan: Accumulation time and correlation of the Dadaepo Formation. Journal of the Geological Society of Korea, 47, 1-18 (in Korean with English abstract).
- Cho, S.W., Chung, E.S. and Kim, M.M., 2003, Estimation of slope movement by earthquake loading in the region of moderate seismicity. Journal of Civil and Environmental Engineering Research, 23, 259-264 (in Korean with English abstract).
-
Dreyfus, D., Rathje, E.M. and Jibson, R.W., 2013, The influence of different simplified sliding-block models and input parameters on regional predictions of seismic landslides triggered by the Northridge earthquake. Engineering geology, 163, 41-54.470.
[https://doi.org/10.1016/j.enggeo.2013.05.015]
-
Fan, X., Xu, Q., van Westen, C.J., Huang, R. and Tang, R., 2017, Characteristics and classification of landslide dams associated with the 2008 Wenchuan earthquake. Geoenvrionmental Disaster, 4, 1-15.
[https://doi.org/10.1186/s40677-017-0079-8]
-
Ferrario, M.F., 2019, Landslides triggered by multiple earthquakes: insights from the 2018 Lombok (Indonesia) events. Natural hazards, 98, 575-592.
[https://doi.org/10.1007/s11069-019-03718-w]
-
Guo, D. and Hamada, M., 2013, Qualitative and quantitative analysis on landslide influential factors during Wenchuan earthquake: a case study in Wenchuan County. Engineering Geology, 152, 202-209.
[https://doi.org/10.1016/j.enggeo.2012.10.012]
-
Harp, E.L., Keefer, D.K., Sato, H.P. and Yagi, H., 2011, Landslide inventories: the essential part of seismic landslide hazard analyses. Engineering Geology, 122, 9-21.
[https://doi.org/10.1016/j.enggeo.2010.06.013]
- Hatchinson, J.N., 1988, Morphological and geotechnical parameters of landslides in relation to geology and hydrology. Proceeding 5th International Symposium on Landslides. Lausanne, July 10-15, 3-35.
-
Huang, C.S., Chen, M.M. and Hsu, M.I., 2002, A preliminary report on the Chiufenershan Landslide triggered by the 921 Chichi earthquake in Nantou, Central Taiwan. Terrestrial, Atmospheric and Oceanic Sciences, 13, 387-395.
[https://doi.org/10.3319/TAO.2002.13.3.387(CCE)]
-
Huang, R., Pei, X., Fan, X., Zhang, W., Li, S. and Li, B., 2012, The characteristics and failure mechanism of the largest landslide triggered by the Wenchuan earthquake, May 12, 2008, China. Landslides, 9, 131-142.
[https://doi.org/10.1007/s10346-011-0276-6]
-
Jeon, S., Kim, D., Choi, W. and Choi, E., 2014, Prediction of the landslide and effect on heritage located in mountain induced earthquake. Journal of the Korean Society of Hazard Mitigation, 14, 139-148 (in Korean with English abstract).
[https://doi.org/10.9798/KOSHAM.2014.14.2.139]
-
Jibson, R.W. and Keefer, D.K., 1993, Analysis of the seismic origin of landslides: examples from the New Madrid seismic zone. Geological Society of America Bulletin, 105, 521-536.
[https://doi.org/10.1130/0016-7606(1993)105<0521:AOTSOO>2.3.CO;2]
-
Jibson, R.W., Harp, E.L. and Michael, J.A., 2000, A method for producing digital probabilistic seismic landslide hazard maps. Engineering Geology, 58, 271-289.
[https://doi.org/10.1016/S0013-7952(00)00039-9]
-
Jibson, R.W. and Michael, J.A., 2009, Maps showing seismic landslide hazards in Anchorage, Alaska. US Geological Survey, 11 p.
[https://doi.org/10.3133/sim3077]
- Kim, K.S., 2007, Soil characteristics according to the geological condition of natural slopes in Busan Area. The Journal of Engineering Geology, 17, 471-481 (in Korean with English abstract).
- Kim, S.H. and Lee, K.W., 2019, A Study on Stabilization of the Collapsed Slope due to Gyeongju Earthquake at Seokguram Access Road based on Geological Investigation. Journal of the Korean Geosynthetics Society, 18, 225-242 (in Korean with English abstract).
- Kim, Y.P., Lim, E.S. and Kim, Y.J., 2004, Restructuring mountain systems in Korean peninsula: Focusing on mountain range analysis. Korea Research Institute for Human Settlements, 208 p (in Korean).
- Kramer, S.L., 1996, Geotechnical earthquake engineering. Pearson Education India, 673 p.
-
Lee, C., Seo, J. and Kang, Y., 2013, Analysis of Landslide Characteristic During Joseon Dynasty through Historical Literature Survey. Journal of the Korean Society of Hazard Mitigation, 13, 161-165 (in Korean with English abstract).
[https://doi.org/10.9798/KOSHAM.2013.13.6.161]
- Lee, H.Y. and Kim, S.W., 1964, Explanatory text of the geological map of Gimhae sheets, 1: 50,000. Korea Research Institute of Geoscience and Mineral Resources (in Korean with English abstract).
-
Lee, J., Han, J.T., Baek, Y., Park, D., Lee, J. and Park, I. J., 2014, Development of Prediction Method Considering Geometrical Amplification Characteristics of Slope Ⅱ: Construction of Landslide Hazard Map during Earthquakes in Seoul. Journal of the Korean Society of Hazard Mitigation, 14, 85-92 (in Korean with English abstract).
[https://doi.org/10.9798/KOSHAM.2014.14.5.85]
- Lee, M.S. and Kang, P.J., 1964, Explanatory text of the geological map of Yangsan sheets, 1: 50,000. Korea Research Institute of Geoscience and Mineral Resources (in Korean with English abstract).
- Lim, H., Jeong, R.-Y., Oh, D., Kang, H. and Son, M., 2020, Liquefaction hazard assessment according to seismic recurrence intervals using simple estimating Method in Busan city, Korea. The Journal of Engineering Geology, 30, 589-602 (in Korean with English abstract).
-
Lim, H., Song, C.W., Ha, S., Kim, M.-C. and Son, M., 2022, Policy suggestions for geological and geotechnical information management in earthquake hazard mitigation measures by local governments. Journal of Korean Earth Science Society, 43, 176-187 (in Korean with English abstract).
[https://doi.org/10.5467/JKESS.2022.43.1.176]
- MOCT (Ministry of Construction and Transportation), 2018, Seismic design standard: KDS 17 10 00 (in Korean).
-
Newmark, N.M., 1965, Effects of earthquakes on dams and embankments. Geotechnique, 15, 139-160.
[https://doi.org/10.1680/geot.1965.15.2.139]
- NIMS (National Institute of Meteorological Sciences), 2012, Historical earthquake records in Korea (2~1904). National Institute of Meteorological Sciences, Seoul, 279 p (in Korean).
- Min, C.S., Jang, B.U. and Cha, G.S., 2005, Comparison between Models for Thickness of Soils. In Proceedings of the Korean Society of Agricultural Engineers Conference, The Korean Society of Agricultural Engineers, Oct 28, 399-403 (in Korean with English abstract).
-
Park, D., Lee, J., Ahn, J.-K., Han, J.-T., Lee, J. and Park, I.-J., 2014, Development of Prediction Method Considering Geometrical Amplification Characteristics of Slope I : Analysis about Amplification Characteristics of Mountain Slopes in Seoul. Journal of Korean Society of Hazard Mitigation, 14, 77-84 (in Korean with English abstract).
[https://doi.org/10.9798/KOSHAM.2014.14.5.77]
- Park, Y.D. and Yoon, H.D., 1968, Geologic map of Korea (Bangeojin sheet 1:50,000). Geological Survey of Korea (in Korean with English abstract).
-
Refice, A. and Capolongo, D., 2002 Probabilistic modeling of uncertainties in earthquake-induced landslide hazard assessment. Computers and Geosciences, 28, 735-749.
[https://doi.org/10.1016/S0098-3004(01)00104-2]
- Sansatai Information System, 2012, http://sansatai.forest.go.kr/, (September 10, 2022).
-
Seed, H.B., Makdisi, F.I. and De Alba, P., 1978, Performance of earth dams during earthquakes. Journal of the Geotechnical Engineering Division, 104, 967-994.
[https://doi.org/10.1061/AJGEB6.0000675]
- Seo, J., Kim, D., Woo, C. and Lee, C., 2019, Analysis of Landslide and Deep-seat Landslide Characteristic During Joseon Dynasty through Historical Literature Survey. Journal of The Korea Society of Forest Engineering and technology, 17, 16-27 (in Korean).
- Son, C.M., Lee, S.M., Kim, Y.K., Kim, S.W. and Kim, H.S., 1978, Explanatory text of the geological map of Dongrae and Weolnae sheets, 1: 50,000. Korea Research Institute of Geoscience and Mineral Resources (in Korean with English abstract).
- Sun, C.G., 2010, Suggestion of additional criteria for site categorization in Korea by quantifying regional specific characteristics on seismic response. Geophysics and Geophysical Exploration, 13, 203-218 (in Korean with English abstract).
- Um, K.-H., 2019, Disaster monitoring for areas with a high tisk of a deep-seated landslide during an earthquake episode. Ph.D. thesis, Kangwon National University, Kangwon, 36 p (in Korean with English abstract).
-
Wang, G., Suemine, A., Zhang, F., Hata, Y., Fukuoka, H. and Kamai, T., 2014, Some fluidized landslides triggered by the 2011 Tohoku Earthquake (MW 9.0), Japan. Geomorphology, 208, 11-21.
[https://doi.org/10.1016/j.geomorph.2013.11.009]
-
Wilson, R.C. and Keefer, D.K., 1983, Dynamic analysis of a slope failure from the 6 August 1979 Coyote Lake. California, earthquake. Bulletin of the Seismological Society of America, 73, 863-877.
[https://doi.org/10.1785/BSSA0730030863]
-
Xu, C., Dai, F., Xu, X. and Lee, Y.H., 2012, GIS-based support vector machine modeling of earthquake-triggered landslide susceptibility in the Jianjiang River watershed, China. Geomorphology, 145, 70-80.
[https://doi.org/10.1016/j.geomorph.2011.12.040]
-
Yin, C., Li, W.-H. and Wang, W., 2021, Evaluation of ground motion amplification effects in slope topography induced by the arbitrary directions of seismic waves. Energies, 14, 6744.
[https://doi.org/10.3390/en14206744]
-
Zhang, S., Zhang, L.M., and Glade, T., 2014, Characteristics of earthquake-and rain-induced landslides near the epicenter of Wenchuan earthquake. Engineering Geology, 175, 58-73.
[https://doi.org/10.1016/j.enggeo.2014.03.012]