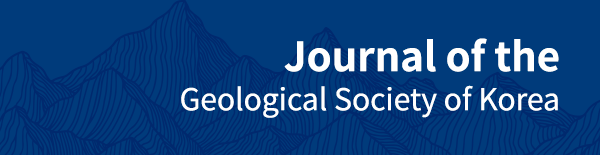
한반도 충북지역에서 최초로 발견된 고지진의 층서학적 기록





초록
국내 고지진학 연구는 한반도 동남부에 집중되어왔으나, 최근 수행된 중부 내륙을 관통하는 공주단층계에 대한 조사결과 중 하나로 충청북도의 두 지점(외북지점과 백마령지점)에서 고지진 지표파열의 층서학적 기록들을 처음으로 발견하였다. 각 지점에서의 특징을 요약하면 다음과 같다. (1) 청주 외북지점의 경우, 서쪽으로 경사하는 지표파열면을 따라 역이동성 고지진 지표파열이 1회 확인되었으며 수직변위량은 15.5±1.7 cm이다. 변위된 가장 젊은 지층과 변위되지 않은 가장 오래된 지층의 퇴적 연대를 근거로 51±4 – 45±3 ka 사이에 지표파열을 동반한 지진이 발생한 것으로 해석된다. (2) 음성군 백마령지점에서는 저각으로 서쪽 경사하는 지표파열면을 따라 고지진 지표파열이 관찰되었다. 백마령지점의 지표파열에 의해 변위된 부정합 및 여러 층의 경계를 기준으로 측정된 수직변위량에 대한 누적변위확률밀도를 분석한 결과, 16.8 cm와 21.6 cm의 수직변위량을 동반한 고지진 지표파열이 총 2회 발생한 것으로 해석된다. 이 조사결과들은 한반도 충북지역에서 고지진에 의한 층서학적 변위 기록을 최초로 보고하는 것으로, 한반도 중부 내륙에서도 신기지구조 환경하에서 규모 6 내외에 이르는 중규모 지진이 발생했음을 지시한다.
Abstract
Paleoseismological studies in Korea have mainly been conducted on the southeastern part of the Korean Peninsula. However, stratigraphic records of paleoearthquake surface rupture were detected at two sites (Oebuk and Baekmaryeong) in the Chungbuk region as one of the results of a recent survey on the Gongju Fault system that penetrates the central part of the Korean Peninsula. We summarized the characteristics of the paleoearthquake surface rupture at two sites: (1) Surface rupture with about 15.5±1.7cm of vertical offset occurred on a low-angle dipping rupture plane associated with one reverse-slip earthquake event at the Oebuk(OB) site, Cheongju-si. The timing of the paleoearthquake at this site was estimated to have occurred between 51±4 and 45±3 ka based on depositional ages of sedimentary strata yielded by the optically stimulated luminescence (OSL). (2) Surface ruptures were observed on a reverse-slip rupture plane dipping to the west at the Baekmaryeong (BMR) site, Eumseong-gun. We estimated their cumulative offset probability density (COPD) using vertical offsets of each layer. The result shows that two events of surface rupturing earthquakes, with about 16.8 cm and about 21.6 cm of vertical offset in respective events, occurred on the rupture plane. The primary purpose of this study is to report new stratigraphic evidence of paleoearthquakes around the Chungbuk area. We conclude that this area experienced moderate (M = ~6) earthquakes under the present tectonic stress condition.
Keywords:
paleoearthquake, stratigraphic offset, vertical offset, reverse-slip, surface rupture키워드:
고지진, 층서변위, 수직변위, 역이동, 지표파열1. 서 론
한반도의 신기지각변형은 주로 소규모 지진을 통해 응력이 해소되며 따라서 비교적 안정한 지괴로 여겨져 왔다(Jin and Park, 2006). 이에 따라 미소지진 자료 분석을 통해 한반도의 지구조적 특성을 이해하려는 시도들이 이어지고 있다(Kim, J. et al., 2017; Kim, K.-H. et al., 2018; Geological Society of Korea, 2019; Lim et al., 2021; Sheen, 2021; Hong et al., 2022a; Kwak et al., 2022). 그러나 한편으로는 한반도 신기지각변형이 주로 중대규모 지진에 의해 진행되고 있다는 의견이 제시되었다(Kim et al., 2011, 2020a). 즉 과거 지표파열을 수반한 대규모 지진기록을 추적하는 고지진학적 연구의 중요성이 부각되었으며(Choi et al., 2017; Kim et al., 2020a), 특히 2016년 경주지진과 2017년 포항지진으로 인해 고지진학적 연구가 더욱 활발히 수행 중이다(e.g., Cheon et al., 2020; Song et al., 2020). 한반도 신기지각변형이 중대규모 지진을 수반한 주요 단층시스템에 의해 주도된다면, 지진재해평가에 있어 국내 주요 단층시스템의 분절(구간)화 및 각 분절별 고지진 정보(재발주기, 최후기 운동시기 등)를 도출하고, 이를 근거로 지진단층의 재발모델을 규명하는 것이 매우 중요하다(Choi et al., 2017). 이러한 연구에서 핵심적인 자료는 지표 퇴적층에 기록되어 있는 선사시대 고지진 자료이다.
선사시대 고지진 자료는 크게 지형학적 기록(e.g., Klinger et al., 2017; Kim and Seong, 2021), 층서학적 기록(e.g., Cheon et al., 2020) 및 단층암 연대기록(e.g., Lee and Schwarcz, 2001)으로 구분된다. 지형학적 기록은 대규모 지진에 수반된 지표파열을 기록하는 지형학적 변형기록으로, 가장 대표적인 예로 현재의 지구조환경이 유지되어온 제4기에 형성된 지형학적 지시자(선상지, 단구, 하천 등)에서 관찰되는 천이점(knickpoint), 단층애(fault scarp), 새그폰드(sag-pond), 셔터리지(shutter-ridge)등을 들 수 있으며, 지형학적 기록은 고지진 지표파열의 자취를 추적하는데 이용되며, 주로 누적변위량 및 지형학적 변형지시자의 형성시기에 대한 연대분석을 통해 변위율 도출에 활용된다. 하지만 암상에 따른 경연차이 혹은 지질구조를 따른 차별 침식으로 인해 변형의 크기가 과대평가되거나 이와 반대로 활발한 침식, 퇴적 작용에 의해 변형의 크기가 과소평가되거나 그 흔적이 남아 있지 않는 경우가 있다. 층서학적 기록의 경우, 신기지구조환경에서 퇴적된 지층의 변위 기록을 뜻하며, 비록 이러한 변위가 화산활동이나 산사태와 같이 지진이 아닌 다른 기원에 의해 발생할 수 있으나, 주변지역의 단층(대)와 유사한 기하와 특성을 보이는 지표변위의 경우 고지진의 증거로 활용할 수 있다. 보통 고지진을 겪은 가장 젊은 지층과 겪지 않은 가장 오래된 지층의 퇴적연대분석을 통해 고지진의 발생시기를 제한한다. 따라서 층서학적 기록은 관찰지점에서의 고지진 지표파열의 존재뿐만 아니라 고지진 발생횟수, 재발주기, 최후기 운동시기 등과 같은 시간적인 정보를 도출하는데 활용된다. 단층암 기록은 단층암의 전자회전공명(electron spin resonance; ESR) 신호를 활용한 연대 측정 방법이며, 일정 압력 조건이 충족될 때, 단층의 순간적인 고속 전단활동에 의해 단층암(단층비지)에 포함된 석영입자의 ESR 신호가 초기화 되는 원리에 기반한다(Lee and Schwarcz, 1993). 주로 최후기 지진성 단층운동의 시기를 지시하며, 중첩된 단층활동으로 형성된 여러 매의 단층암 시료가 존재한다면 이론적으로 각각의 단층운동시기를 분석 가능하다. 다만, ESR 신호가 충분히 초기화 될 온도압력 조건이 갖춰지지 않는 경우 기존의 ESR 신호가 남아있어 오래된 연대가 도출될 가능성이 존재한다(Kim and Lee, 2020).
기존에 한반도에서 수행된 고지진학적 연구는 주로 양산단층과 울산단층을 중심으로 동남권에 집중되었으며, 고지진 지표파열에 의해 발달한 지형 기록, 층서 기록, 단층암 기록 등은 다수 보고되어 왔다(그림 1; e.g., Okada et al., 1994; Kyung, 2003; Kim and Jin, 2006; Kee et al., 2007; Choi et al., 2012; Lee et al., 2015; Cheon et al., 2020; Kim et al., 2020b; Song et al., 2020; Kim and Seong, 2021). 그러나 동남권 이외의 지역에서의 고지진학적 연구는 비교적 제한적이다(Choi et al., 2015; Kim and Lee, 2020; Shin et al., 2020). 한편 한반도 중부 내륙에서도 피해를 일으킨 지진이 역사문헌에 기록된 바 있으며(KMA, 2012), 최근 충북 괴산지역에서 지역규모 4.1의 지진이 발생하였다(KMA, 2022). 따라서 동남권 이외의 지역에서 꾸준히 중소규모의 지진이 발생하고 있어 고지진 조사의 필요성이 제기되고 있다(그림 1a).
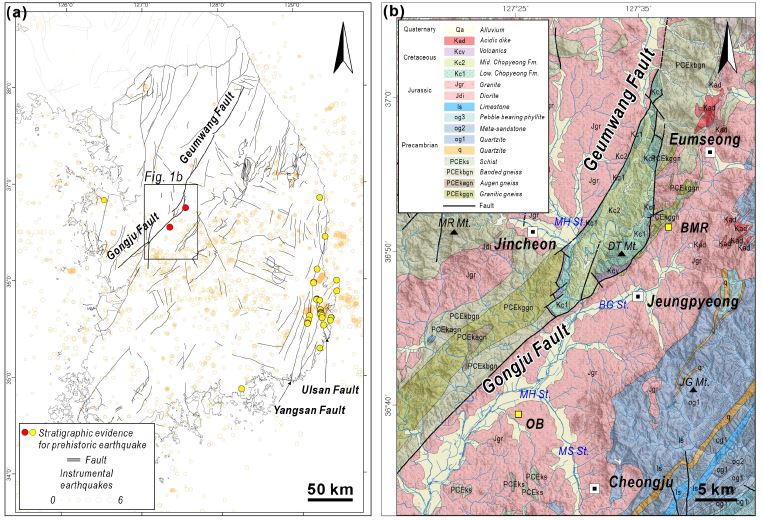
(a) Fault map (modified from Kee et al., 2019) and instrumental seismicity (from 1978 to 2021; KMA) of South Korea. Yellow circles are locations of pre-reported stratigraphic evidence of paleoearthquake surface rupture (Choi, S.J. et al., 2012; Choi, P.-Y. et al., 2015; Lee et al., 2017). (b) 1:250k geological map around the study area (modified from Lee et al., 1996; OB: Oebuk, BMR: Baekmaryeong, MH St.: Miho Stream, BG St.: Bogang Stream, MS St.: Musim Stream, DT Mt.: Doota Mountain, MR Mt.: Manroi Mountain, JG Mt.: Jwagu Mountain).
이에 맞춰 한국지질자원연구원 활성지구조연구센터를 중심으로 공주단층계 연구사업이 수행 중에 있으며, 그 결과의 일환으로 충북지역에서 선사지진의 층서학적 증거들을 발견하였다(e.g., Ryoo et al., 2022). 따라서 이 논문에서는 충북의 2개 지점을 대상으로 각 지점별 획득된 고지진 정보를 설명하고, 지진지표파열 기록의 고지진학적 의미 및 중요성을 고찰하고자 한다.
2. 연구 지역
한반도에는 양산단층과 울산단층 외에도 추가령단층와 공주단층를 비롯한 대규모 단층(계)들이 다수 발달한다(그림 1a; Kee et al., 2019). 강원도 인제에서 북동-남서 방향으로 충북 진천까지 이어지는 금왕단층과 충북 증평에서 충남 부여에 이르는 북동-남서 방향의 공주단층 등은 주향이동단층이다(Ryang, 2013). 연구지역은 신원생대와 고생대 변성퇴적암류와 변성화성암류, 이들을 광역적으로 관입한 쥬라기 반상화강암 및 조립질 복운모 화강암이 분포하며(Lee and Kim, 1971; Kwon and Jin, 1974; Cheong et al., 1976; Kim et al., 2006), 이들을 절단하는 북동-남서 방향의 금왕단층과 공주단층의 단층연결부(fault linkage) 사이에 음성분지가 발달하고 있다. 음성분지는 주로 백악기 화산암류 및 퇴적암류로 충전되어 있다(Choi, 1996; Ryang, 2003, 2013). 백악기의 산성질 관입암류가 연구지역 전반에 걸쳐 남-북 내지 북동-남서 방향으로 관입하고 있으며, 제4기 충적층이 피복하고 있다(그림 1b; Lee et al., 1996). 음성분지를 비롯해 금왕 및 공주단층을 따라 발달하는 소규모 백악기 소분지들의 기하, 분지퇴적물의 층후, 퇴적상 및 경계단층의 특성에 기초해 이들 소분지들은 금왕단층 및 공주단층의 좌수향 주향이동운동에 의해 발달하는 당겨-열림분지(pull-apart basins)로 알려져 있으며(Kim et al., 1994; Lambiase and Bosworth, 1995; Choi, 1996; Lee, 1998; Lee and Kim, 2003; Ryang, 2003, 2013; Choi and Choi, 2007) 후기 백악기 내지 고원기(Paleogene)부터 우수향 주향이동 운동을 경험한 것으로 해석되고 있다(Kim and Lee, 2016, 2020). 특히 최근에는 금왕단층과 공주단층을 따라 ESR 연대측정 연구결과(e.g., Lee, 2010; Choi et al., 2012; Hong and Lee, 2012; Hong, 2013; Bae and Lee, 2014; Kim and Lee, 2016, 2017, 2020; Kim, 2018; Weon and Lee, 2018; Min, 2020)와 지형학적 연구(Hong et al., 2022b)를 통해 지질학적으로 비교적 최근에 지표파열을 수반한 단층거동의 가능성이 제기된 바 있다.
주요한 지형적 특성은 두타산(해발고도 598 m), 만뢰산(해발고도 611 m), 좌구산(해발고도 657.7 m) 등 상대적으로 높은 고도의 산지지형이 발달하는 반면, 미호천, 보강천, 무심천 등 금강의 주요 지류하천 주변으로는 넓고 낮은 저지대가 발달하고 있어 이들의 기복차이는 현저하게 인지된다. 주로 쥬라기 화강암류가 분포하는 구간이 낮은 저지를 이루고 있고, 음성분지의 퇴적암류나 선캠브리아 내지 시대미상의 편암-편마암류로 구성된 지역이 상대적으로 산지지형을 이루고 있다(그림 1b). 연구지역의 주요 수계로는 진천과 음성의 서편에서 발원하여 남쪽으로 흐르는 미호천, 증평에서 발원하여 서쪽으로 흐르는 보강천, 청주에서 발원하여 북쪽으로 흐르는 무심천 등이 있으며, 이 하천들 주변으로 넓은 범람원 지형이 형성 되어있다. 산지와 저지의 경계에는 완경사를 이루는 선상지가 선형적으로 발달한다.
연구지역은 행정구역상 충청북도 청주-증평-음성에 이르는 구간의 공주단층 북동부 근처이며, 고지진 조사지점의 위치는 각각 충청북도 청주시 흥덕구 외북동, 충청북도 음성군 원남면 문암리에 속한다. 이 논문에서 외북동에서 발견된 고지진 기록 지점은‘외북지점’으로, 그리고 문암리 백마령 고개 근처에서 발견된 고지진 기록 지점은 ‘백마령지점’으로 통칭한다.
3. 외북지점
3.1 조사위치 및 연구 방법
외북지점은 충북 청주시 흥덕구 외북동 일원의 구석기 유물 발굴조사[(재)미래문화재연구원]가 진행된 곳으로, 조사현장 주변의 35번 고속도로(중부고속도로)에서 동쪽으로 약 100 m 떨어진 지점이다(그림 2). 유물 발굴 간에 지진지표파열이 관찰되어, 지표파열의 기하학적 및 운동학적 특성을 규명하고, 고지진학적 발달사를 해석하기 위한 기재를 수행하였다. 노출된 단면의 크기는 약 1.5 m 내외로 조사단면에 격자를 설치하지 않고 기재하였다. 퇴적물의 입도, 색, 조직적 특성에 기초해 퇴적층을 구분하였으며, 단층의 절단관계를 바탕으로 고지진 변형사를 해석하였다. 고지진 발생시기를 규명하기 위해 광여기루미네선스(optically stimulated luminescence; 이하 OSL) 연대측정을 수행하였으며, 원통형 파이프를 활용해 샘플을 채취해 한국지질자원연구원에 설비된 암실에서 전처리 및 등가선량 측정을 수행하였다. 또한 지표파열면에 의해 절단된 퇴적층에서 발견된 목탄시료를 대상으로 방사성 탄소연대측정을 수행하였다. 시료의 전처리 및 분석은 한국지질자원연구원에서 수행하였으며, 측정된 탄소연대는 IntCal20 (Reimer et al., 2020)을 활용하여 보정하였으며, 이때 OxCal4.4 보정프로그램(http://c14.arch.ox.ac.uk/oxcal.html)을 사용하였다(Bronk Ramsey, 2009).
3.2 지표파열 발달특성
외북지점에서는 무인항공기(unmanned aerial vehicle; UAV) 정사영상 및 현장에서 풍화된 화강암과 이를 관입한 북북동-남남서 내지 북동-남서 주향의 중성질 암맥들이 잘 관찰되며, 지진지표파열의 자취(trace) 또한 쉽게 인지된다(그림 2). 굴착 단면에서는 화강암 상위에 미고결 퇴적층이 피복하고 있으며 상위로부터 유기물 함량이 많은 적갈색의 실트질 퇴적물로 구성되는 표토층(10), 녹색 내지 황토색의 실트층(20), 그리고 가장 하부에 기반암을 피복하는 적색 내지 녹회색의 모래질 점토층(30) 등 크게 3개의 단위 퇴적층으로 구분하였다(그림 3). 또한 20번 퇴적층과 30번 퇴적층 상부에는 수직 방향의 토양 쐐기가 발달한다. 30번 퇴적층은 퇴적물의 입도와 색의 변화에 따라 상부에서부터 쐐기가 발달하는 녹회색 내지 황갈색의 실트층(31)과 황색의 조립질 모래층(32) 그리고 적색의 점토층(33)으로 세분된다(그림 3b).
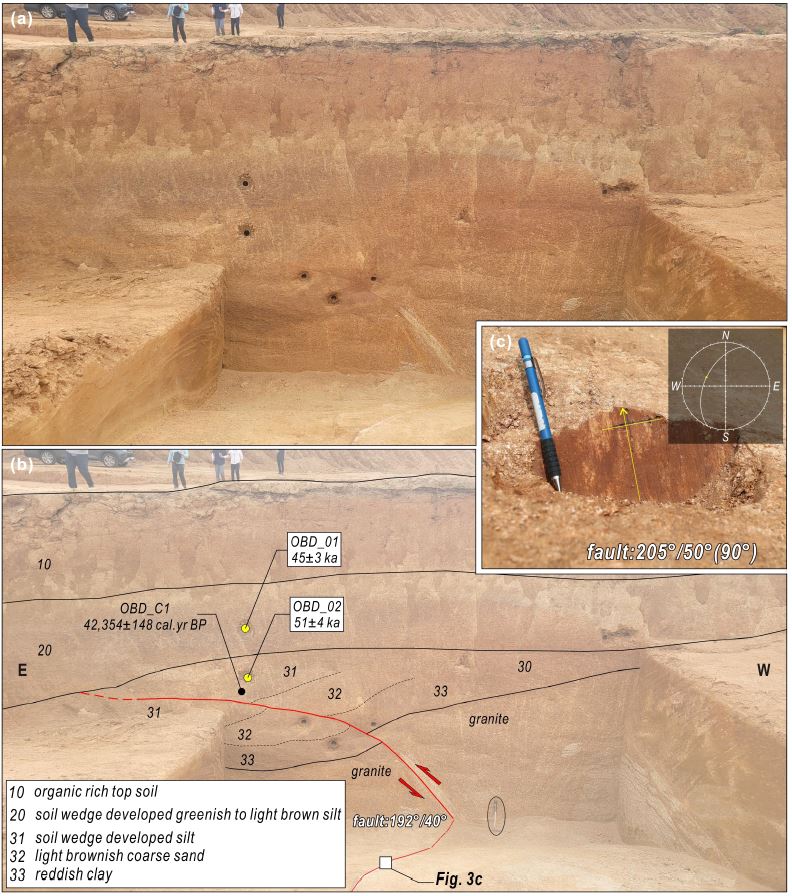
Section view (a) and its sketch (b) of the southern wall at OB site. Three main unconsolidated sedimentary layers are classified as unit-10, -20, and -30, and unit-30 was subdivided into three sub-units based on their lithologic features. The yellow circles show the OSL sample locations. The black circle indicates the radiocarbon sample location. (c) Close-up photo of the slip surface and slicken line. The measured fault surface and striation are projected on the stereo-net in the inset.
기반암 내에는 저각으로 서쪽 경사하는 수 매의 평행한 역단층들이 발달하며, 이들 가운데 하나의 단층이 30번대 퇴적층을 절단한다. 이 고지진 파열면의 주향은 측정위치에 따라 차이가 있으나, 대체로 북북동-남남서 방향이 우세하다(그림 3). 굴착바닥의 화강암 내에서 측정된 이 단층면의 자세는 N25°E/50°NW로 자세로 발달하며, 기반암에서 약 40-50°내외의 경사를 가지나 상부의 미고결 퇴적층 내에서는 저각으로 변화한다. 31-33번 퇴적층 모두 약 15.5±1.7 cm 내외의 일관된 수직 낙차를 가지며 역이동성 운동감각으로 변위되어 있다. 변위된 층의 두께가 상하반에서 일정한 점을 바탕으로 순수한 역이동성 지진을 경험한 것으로 보인다. 기반암 단층면에 발달하는 단층조선 또한 선주각이 거의 수직에 가까워 순수한 경사이동운동을 간접적으로 지시한다(그림 3c). 지표파열면이 분기되는 양상 없이 단일한 면만 관찰됨에 따라 적어도 이 지점에서 인지할 수 있는 지표파열의 횟수는 1회로 판단된다.
고지진의 활동시기를 제한하기 위해 변위된 미고결 퇴적층 중 가장 젊은 퇴적층(31)과 변위되지 않은 가장 오래된 퇴적층(20)에서 각각 51±4 ka와 45±3 ka의 OSL 연대가 도출되었다(그림 3b; 표 1). 또한 31번 퇴적층에서 획득한 목탄시료를 이용하여 방사성 탄소연대측정 결과 42,354±148 cal. yr BP의 연대가 산출되었다(표 2).
3.3 외북지점의 최후기 운동시기
외북지점에서 확인된 고지진 지표파열의 최후기 운동시기는 OSL연대에 기초해 51±4 ka - 45±3 ka 사이로 제한할 수 있다(그림 3). 외북지점 31번 퇴적층에서 획득된 방사성탄소연대측정 결과는 OSL 연대측정결과 보다 오차범위 밖에서 약 5 ka 젊게 확인되지만, 방사성탄소연대의 분석 상한에 가까워 이를 최소연령으로 해석하는 것이 합리적이라 생각된다. 즉 외북지점에서의 최후기 운동은 51±4 ka - 45±3 ka 사이에 한 번 발생하였을 것으로 해석된다. Choi et al. (2012)은 외북지점에서 북쪽으로 약 6.6 km 떨어진 공주단층의 단층암에서 채취한 시료의 ESR 연대측정 결과 약 51만년 전에 거동이 있었음을 보고한 바 있다. 또한 충남 공주시 의당면에서 수행된 ESR 연대결과는 약 87만 년 전, 충남 부여군 석성면에 발달하는 단층의 ESR 연대는 약 52-56 만 년, 충남 당진에서 발견된 정단층의 ESR 연대는 약 43만 년, 65 만 년으로 도출 된 바 있다(Choi, S.J. et al., 2012; Choi, P.-Y. et al., 2015). 하지만 ESR 연대측정에는 충분한 압력조건이 갖춰진 상태에서 단층이 거동해야만 제대로 된 연대결과를 제시할 수 있기 때문에 약 10만 년 이내의 젊은 단층활동을 분석하기에 제약이 따른다(Cheong et al., 2003; Lee and Yang, 2003; Kim and Lee, 2020). 이번 연구의 외북지점에서 제한한 단층의 활동시기는 충청권에서 보고된 조사결과 중 가장 젊고, 이 때에 지표파열이 동반되었다는 사실은 고지진학적으로 큰 의미를 가진다.
4. 백마령지점
4.1 조사위치 및 연구 방법
연구 지점은 증평과 음성 사이를 잇는 36번 국도의 백마령 터널의 증평쪽 출구에서 약 1 km 남서쪽에 위치한 공사 현장이다(그림 4a). 공사 절개사면은 북동-남서 방향으로 약 80 m가량 노출되어 있으며, 남동쪽 사면의 중앙 부분에서 조사를 수행하였다(그림 4b). 백마령지점에서는 풍화가 많이 진행된 화강암을 기반으로 그 상위에 미고결 퇴적층이 발달한다(그림 4c). 백마령지점에서는 퇴적층 분대, 단층의 기하와 운동학적 특성 분석 등을 수행하였으며, 특히 세세하게 분대한 퇴적층의 경계면을 기준으로 변위량을 측정하여 이를 고지진 이력해석에 활용하였다.
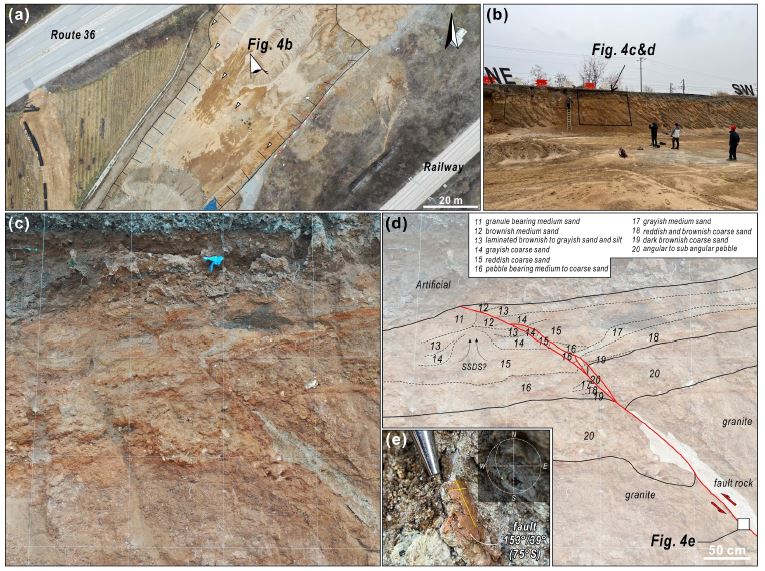
Photos of the BMR site. (a) Map view images, derived by UAV, of the studied site. Paleoearthquake surface rupture marked by white triangles. (b) Overall view of the fault outcrop. Section view (c) and its sketch (d). Unconsolidated sedimentary layers can be classified into two units (unit-10 and -20), and unit-10 is subdivided into nine sub-units based on their lithological features for measuring displacement. (e) Close photo of the slip surface and slicken line. The measured fault surface and striation are projected on the stereo net in the inset.
일반적으로 지진의 규모는 변위량에 비례한다(Wells and Coppersmith, 1994). 지형학적 변위는 최후기 지진에 의한 변위량 뿐만 아니라 반복된 고지진 지표파열에 의한 누적된 변위량을 지시할 수 있는데, 이들을 구분할 수 있다면 고지진 지표파열의 횟수 및 각각의 지진규모를 추정할 수 있다(McGill and Sieh, 1991; Zielke et al., 2010; Klinger et al., 2011). 하지만 단층 변형의 이방성때문에 변위 측정에 불확실성이 존재한다(Nicol et al., 2002; Rockwell et al., 2002; Long and Imber, 2010; Miliner et al., 2015; Kim et al., 2020b). 이러한 불확실도를 활용해 변위량을 확률밀도함수(probability density function; PDF)로 표현할 수 있다(McGill and Sieh, 1991). 변위량에 대한 PDFs를 누적하여 누적변위확률밀도함수(cumulative offset probability density function; COPD)를 도시하면 특정 범위의 변위량에 대한 피크가 발생하는데, 이를 활용해 변위량 및 변형횟수의 도출이 가능하다(McGill and Sieh, 1991; Zielke et al., 2010; Kurtz et al., 2018). 본 연구에선 백마령지점 노두상에서 관찰되는 미고결 퇴적층의 경계를 수직변위를 측정하기 위한 지시자로 활용하였고, 단층면과 접하는 층리를 기준으로 직접 측정한 값을 최소변위(Vmin), 층이 수평하다는 가정으로 전반적인 트렌드를 추정하여 단층면에 투영 후 측정한 값을 최대변위(Vmax)로 설정하였으며, 끌림습곡을 고려해 변형 이전의 층리를 단층면에 투영하여 측정한 변위량을 대표값(preferred separation)으로 사용하였다(그림 5a의 삽화). 그 결과는 표 3에 정리하였고, 이를 활용하여 매트랩코드를 사용해 COPD를 작도하였다(그림 5). 하나의 지표파열면이 아닌 2개 이상의 지표파열면에 의해 각각 변위가 인지되는 경우, 동일한 지층 경계를 기준으로 한 변위는 각 지표파열면을 따라 각각의 변위량을 따로 측정하고, 이를 합산하여 총 변위량으로 판단하였다(그림 5b; 표 3).
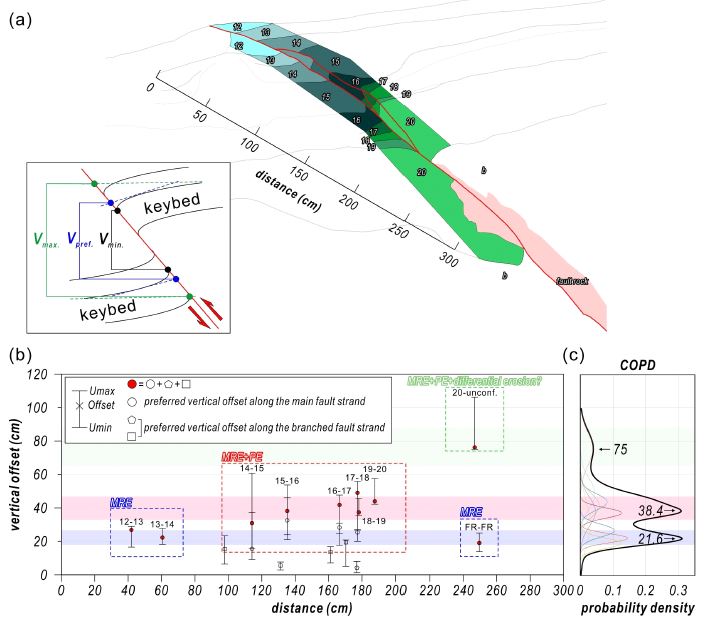
Corelative key beds of the vertical separation along the fault trace in the BMR site (a) and measured vertical separation along the fault (b). Uncertainties of the vertical separation were measured using the general trend of each stratum (Vmax), and the shortest distance along the fault (Vmin) (inset in Fig. 5a). (c) The probability density function for each separation and its uncertainties were constructed and we stacked these PDFs as a cumulative offset probability density. Three picks are recognized for COPD at 21.6 cm, 38.4 cm, and 75 cm, respectively.
4.2 지표파열 발달특성
미고결 퇴적층은 최상부의 아스팔트와 그 하위의 매립층을 제외하면 실트질 모래층(10)과 그 하위의 자갈층(20)으로 구분된다. 실트질 모래층(10)은 약 1 m 내외의 층후를 보이며, 주로 조립질에서 세립질까지 다양한 입자크기를 가지는 모래층이 발달하며 부분적으로 실트질 퇴적층도 확인된다. 실트질 모래층 하위에 발달하는 자갈층(20)은 약 50-60 cm의 층후를 보이며 각형 내지 아각형의 원마도를 가지는 왕자갈 내지 잔자갈로 구성된다. 실트질 모래층(10)은 횡적 연장성이 양호하지 않으나 단층면을 중심으로 입도 및 색의 변화가 비교적 뚜렷하게 인지됨에 따라 9개의 퇴적층으로 세분된다. 세분된 퇴적층은 위에서부터 잔자갈을 포함하는 중립질 모래층(11), 갈색의 중립질 모래층(12), 엽층리가 발달하는 갈색과 회색이 혼재된 실트 내지 세립질 모래층(13), 회색의 조립질 모래층(14), 적색의 조립질 모래층(15), 자갈을 포함한 중립 내지 조립질의 모래층(16), 회색의 중립질 모래층(17), 적색 내지 갈색의 조립질 모래층(18), 암갈색의 조립질 모래층(19)으로 구성된다(그림 4d). 13-15번 퇴적층이 발달하는 구간에서는 하부의 15번 퇴적층이 상부의 13번 퇴적층으로 밀려 올라간 연질퇴적변형구조(soft-sediments deformation structure, SSDS; 그림 4d)가 관찰된다.
백마령지점의 고지진 지표파열면은 완만하게 서쪽으로 경사하며 매립층을 제외한 모든 미고결 퇴적층과 기반암을 절단한다. 화강암 내에서는 하나의 전단면이 발달하는 반면, 미고결 퇴적층에서는 수 매의 지표파열면으로 분기되었다가 다시 하나로 합쳐지는 양상이 관찰되며, 경사는 더 완만한 특징을 보인다. 기반암에서는 15-20 cm 내외의 두께로 단층 점토가 발달하나 20번 퇴적층 내에서 30 cm 내외로 두꺼워지는 양상을 보인다. 이는 미고결 퇴적층을 절단하는 단층운동 시에 하부 기반암 내에 발달하던 단층물질이 미끌림 면을 따라 상부의 미고결 퇴적층으로 주입(squeezing)된 결과로 추정된다. 또한 20번 층 내에 발달하는 단층비지 역시 고지진 지표파열에 의해 변위되어 있다. 변위된 지층은 단층 주변에서 휘어져 있으며, 주로 역이동성 운동감각을 지시하는 끌림습곡으로 해석된다. 단층에 의해 절단된 상하반 퇴적층의 두께가 일정한 점을 근거로 순수한 역단층 운동을 겪었을 것으로 판단된다(그림 4d). 기반암에서 측정된 미끌림면의 자세는 N27°W/39°SW 이며, 단층조선의 선주각은 75-80°S 내외인 점을 비춰봤을 때, 거의 순수한 역단층을 뒷받침한다(그림 4e)
세분된 퇴적층의 경계를 활용해 측정한 변위량은 다음과 같다. 12-13 층의 경계와 13-14 층의 경계는 약 22-27 cm가 도출되었고, 14-15층은 약 31 cm의 변위가 도출되었다. 15-16 층의 경계는 38.2 cm, 16-17층의 경계는 약 42 cm, 17-18 층의 경계는 약 49 cm, 18-19 층의 경계는 37 cm, 19-20 층의 경계는 44 cm, 기반암과 20번 층의 부정합은 약 76 cm가 도출되었다. 20번 층으로 밀려 들어간 단층비지대는 약 19.2 cm의 변위량을 가진다. 측정된 변위와 이들의 불확실도를 바탕으로 COPD를 도시한 결과, 작은 변위량부터 차례로 21.6 cm, 38.4 cm, 75.0 cm 등 3개의 정점이 인지된다(그림 5c).
4.3 백마령지점의 고지진 이력
COPD분석결과 확인되는 세 개의 정점은 세 번의 변형 작용을 지시한다. 각 정점에 해당하는 경계를 살펴보면, 우선 가장 변위량이 작은 21.6 cm의 변위를 가지는 운동은 12-13번 층의 경계와 13-14번 층의 경계, 그리고 20번층 내에 주입된 단층암이다. 단층암을 제외하면 사면에서 인지되는 자연 퇴적물 중 최상부에 발달하는 층으로 판단된다. 두번째로 큰 변위량을 지시하는 38.4 cm의 정점에 해당하는 경계는 14번층부터 20번층까지의 경계이다. 가장 큰 75 cm의 변위를 가지는 경계는 20번 층과 기반암의 부정합면이다. 20번 퇴적층 내에 주입된 단층암이 다시 절단된 양상은 20번 퇴적층이 퇴적된 이후 단층암이 주입이 되는 사건이 발생하였고, 이후 한 번 더 단층운동에 의해 주입된 단층암이 절단되었음을 지시한다. 즉 주입된 단층암에서 측정된 19.2 cm의 변위량은 최후기운동(most recent earthquake)의 변위량으로 추정된다. 이 변위량은 최상위 퇴적층의 변위량과 일치한다. 즉 12번 퇴적층이 퇴적된 이후 약 20 cm 내외의 수직변위량을 가지는 역이동 지진 지표변위가 발생 것으로 해석된다. 두 번째 COPD 정점인 38.4 cm 내외의 변위량은 앞서 최후기운동의 변위량인 약 20 cm의 거의 두 배이다. 15-19번 퇴적층 발달 이후 약 20 cm 내외의 수직변위량을 가지는 지진지표파열이 한 번 더 있었고, 40 cm 내외의 변위량은 두 번의 지진으로 인해 누적된 변위를 지시한다. COPD의 가장 큰 변위량 피크인 75.0 cm는 화강암과 미고결 퇴적층의 부정합경계를 기준으로 측정되었다. 이 결과에 대해서는 두 가지의 가능성을 고려할 수 있다. 첫째로 미고결 퇴적층이 퇴적되기 이전의 변위가 누적되어 기록되어 있었을 가능성과, 두번째는 단층대 주변으로 차별침식에 의해 누적 변위량이 과평가 되었을 가능성이 있다. 만약 전자에 의한 결과라면 기반암 직상부에 발달하는 20번 층이 발달할 당시 단층주변으로 단층애에 의한 지형기복이 존재했어야 하며, 이러한 급격한 낙차는 상하반에서 입도변화 등 퇴적학적 특성에 차이가 발생했을 것이다. 후자의 경우, 단층에서 차별침식이 활발하게 일어나 점이적인 지형기복을 가진다면 퇴적상의 변화가 거의 없을 수 있다. 따라서 지표파열 상하반의 퇴적층서가 거의 유사하다는점, 75.0 cm 피크는 하나의 변위자료에서 도출된 점, 피크 자체가 다른 두 피크에 비해 현저히 낮은 점 등을 토대로, 후자의 경우 합리적이며 이를 지표변위에서 제외하는 것이 타당하다고 판단된다.
이를 종합하여 백마령지점의 고지진 이력을 해석하면 그림 6과 같다. 최초 단층대 주변에는 차별침식으로 부정합면의 고도가 낮은 지형이 발달하였고, 이 부정합면을 피복하는 20번층 부터 15번 층이 퇴적되었다. 이후 약 20 cm의 수직변위량을 가지는 고지진 지표파열이 있었고, 이때 단층암 물질이 미고결 퇴적층 내부로 주입되었을 것으로 판단된다. 지표파열 이후에 14번부터 11번 퇴적층이 새롭게 퇴적되고, 이후 다시 한번 약 20 cm 내외의 변위량을 가지는 지진이 발생하였다. 이를 통해 최후기 직전 운동(penultimate earthquake)과 최후기 운동을 모두 겪은 20번 층에서 15번 층까지는 약 40 cm 내외의 누적 변위량을 기록하고, 최후기 운동만 경험한 14-11번 층 및 최후기 직전 운동때 주입된 단층비지는 20 cm 내외의 단일 변위량을 기록하게 된다. 마지막으로 도로의 건설로 현재의 노두 양상이 발달했을 것으로 해석된다.
5. 결론 및 시사점
본 연구를 통해 고지진에 의한 지표파열을 지시하는 지표지질의 층서 변위가 충북지역의 두 지점에서 처음 확인되었다. 두 지점에서 도출된 고지진 지표파열의 단일 수직변위량은 15.5-21.6 cm 내외이며, 이를 지진규모-변위량 경험식(Wells and Coppersmith, 1994)에 대입하면 6.16-6.23의 지진규모가 계산된다. 한편 이 경험식은 주로 판 경계부에 발생한 지진을 중심으로 추정된 식이므로, 판내부의 느린 변형지역에서의 적용을 위해서는 추가적인 연구가 뒷받침되어야 한다. 하지만 적어도 규모 6 내외에 이르는 중규모 지진이 발생했음은 확실해 보이며, 이는 현재의 한반도 신기지구조환경에서 중규모 지진을 수반한 단층활동이 충청권에서 산발적 혹은 반복적으로 발생했음을 의미한다.
과거 역사지진 기록에서는 1437년 2월 충청도 충주 근처에서 추정진도 VI내외의 지진이 충주, 전주, 강원도, 경상도, 경기도 등에서 감진되었다는 기록이 존재하는 등 역사문헌에는 충청권 및 한반도 중부지역의 지진이 다수 기록되어 있다(KMA, 2014). 이와 더불어 1978년 홍성에서 규모 5.2의 지진이 발생하였으며(Lee et al., 1986; Kwon et al., 2003), 속리산지진으로 알려진 경상북도 상주시 화북면에서 1978년 규모 5.2의 지진(Shin, 2021), 2007년 규모 4.8의 오대산지진(Yun, 2008), 2022년 충북 괴산에서 규모 4.1의 지진(KMA, 2022)등이 발생해 계기지진 관측 이후에도 중규모 지진이 꾸준히 발생하고 있다. 특히 최근 괴산에서 발생한 지진의 경우, 이번 연구지역 중 하나인 백마령지점에서 동쪽으로 불과 20여 km 떨어진 곳에서 발생하였다. Song and Chang (2021)은 한반도 동남부의 응력 자료, 2016 경주지진, 2017 포항지진의 지진자료와 단층 정보를 바탕으로 미끌림 경향도 분석(slip tendency plot; Moeck et al., 2009)을 수행하였으며, 두 지진을 일으킨 지진원 단층의 자세가 양산단층의 주방향보다 더 쉽게 미끌어질 가능성이 높다고 제시한바 있다. 반면, 계기지진에서 한반도 중부지역에서의 중규모 지진은 지표파열을 수반하지 않았고, 역사지진에서도 지표파열의 기록은 보고되지 않았지만, 기존의 고지진 연구결과(e.g., Cheon et al., 2020; Song et al., 2020)와 이번 연구결과는 한반도에서 지표파열을 수반한 중대규모 지진이 발생하였음을 지시한다. 따라서 한반도와 같이 지구조적으로 느린 변형지역에서의 지진특성을 이해하기 위해서는 계기/역사지진 분석 및 GPS 등 측지학적 자료를 바탕으로 지속적인 모니터링이 필요함과 더불어 긴 주기를 가지는 대규모 지표파열의 고지진학적 기록 및 정보가 상호 유기적으로 토의되어야 할 것이다.
Acknowledgments
이 연구는 한국지질자원연구원 기본과제 “판내부 활성지구조특성 연구 및 단층분절모델 개발(GP2020-014)”에 의해 수행되었습니다. 이 논문을 위해 세심한 검토와 건설적인 조언을 해 주신 편집위원장님 및 두 분의 심사위원, 그리고 연구를 진행하는데 많은 도움을 주신 ㈜라드피온 정봉구 과장님께 진심으로 감사드립니다.
References
-
Bae, H.K. and Lee, H.K., 2014, Space-time patterns of fault activity of the Keumwang Fault developed in the JincheonEumseong-gun, Chungcheongbuk-do. Journal of the Geological Society of Korea, 50, 735-752 (in Korean with English abstract).
[https://doi.org/10.14770/jgsk.2014.50.6.735]
-
Bronk Ramsey, C., 2009, Bayesian analysis of radiocarbon dates. Radiocarbon, 51, 337-360.
[https://doi.org/10.1017/S0033822200033865]
-
Cheon, Y., Choi, J.-H., Kim, N., Lee, H., Choi, I., Bae, H., Rockwell, T.K., Lee, S.R., Ryoo, C.-R., Choi, H. and Lee, T.-H., 2020, Late Quaternary transpressional earthquakes on a long-lived intraplate fault: A case study of the Southern Yangsan Fault, SE Korea. Quaternary International, 553, 132-143.
[https://doi.org/10.1016/j.quaint.2020.07.025]
- Cheong, C.H., Park, Y.A. and Kim, H.M., 1976, Geological map of the Eumseong sheet (1:50,000). Korea Institute of Geoscience and Mineral Resources (in Korean with English abstract).
-
Cheong, C.S., Hong, D.G., Lee, K.S., Kim, J.W., Choi, J.H., Murray, A.S., Chwae, U., Im, C.B., Chang, C.J. and Chang, H.W., 2003, Determination of slip rate by optical dating of fluvial deposits from the Wangsan fault. Quaternary Science Revies, 22, 1207-1211.
[https://doi.org/10.1016/S0277-3791(03)00020-9]
-
Choi, J.-H., Kim, Y.-S. and Klinger, Y., 2017, Recent progress in studies on the characteristics of surface rupture associated with large earthquakes. Journal of the Geological Society of Korea, 53, 129-157 (in Korean with English abstract).
[https://doi.org/10.14770/jgsk.2017.53.1.129]
- Choi, P.-Y. and Choi, Y.S., 2007, Stress Model of the Opening of the Cretaceous Eumseong Basin. KIGAM Bulletin, 11, 3-16 (in Korean with English abstract).
-
Choi, P.-Y., Hwang, J.H., Bae, H., Lee, H.-K. and Kyung, J.B., 2015, Kinematics and ESR Ages for Fault Gouges of the Quaternary Jingwan Fault, Dangjin, western Korea. Journal of the Korean Earth Science Society, 36, 1-15 (in Korean with English abstract).
[https://doi.org/10.5467/JKESS.2015.36.1.1]
- Choi, S.J., Jeon, J.S., Song, K.Y., Kim, H.C., Kim, Y.H., Choi, P.Y., Choi, W.C., Han, J.G., Ryoo, C.R., Sun, C.G., Jun, M.S., Kim, G.Y., Kim, Y.B., Lee, H.J., Shin, J.S., Lee, Y.S., Gi, W.S., Lee, H.K., Song, Y.G., Kim, Y.S., Kang, T.S., Hong, D.G. and Kim, S.K., 2012, Active Fault Map and Seismic Harzard Map. Natural Hazards Mitigation Research Group, National Emergency Management Agency.
- Choi, Y.S., 1996, Structural evolution of the Cretaceous Eumseong Basin, Korea. Ph.D. thesis, Seoul National University, Seoul, Korea, 159 p (in Korean with English abstract).
-
Geological Society of Korea, 2019, Summary report of the Korean Government Commission on Relations between the 2017 Pohang Earthquake and EGS Project, 127 p.
[https://doi.org/10.22719/KETEP-20183010111860]
- Hong, N.R., 2013, Structural features and movement history of the Keumwang Fault at Hongcheon-gun, Kangwon-do, Korea. M.S. thesis, Kangwon National University, Chuncheon (in Korean with English abstract).
- Hong, N.R. and Lee, H.K., 2012, Structural features and ESR dating of the Keumwang fault at Eumseong-gun, Chungcheongbuk-do. Journal of the Geological Society of Korea, 48, 473-489 (in Korean with English abstract).
-
Hong, T.-K., Lee, J., Park, S. and Kim, W., 2022a, Major influencing factors for the nucleation of the 15 November 2017 Mw 5.5 Pohang earthquake. Physics of the Earth and Planetary Interiors, 323, 106833.
[https://doi.org/10.1016/j.pepi.2021.106833]
-
Hong, Y.-M., Choi, J.-H., Ryu, H.-G. and Shin, J.-R., 2022b, Geomorphological Development and Neotectonic Crustal Deformation along the Geumwang Fault (I): Developmental Characteristics and Distribution of the Quaternary Landforms. Journal of the Korean Geographical Society, 57, 81-95 (in Korean with English abstract).
[https://doi.org/10.16968/JKGA.28.1.67]
-
Jin, S. and Park, P.-H., 2006, Strain accumulation in South Korea inferred from GPS measurements. Earth, Planets and Space, 58, 529-534.
[https://doi.org/10.1186/BF03351950]
- Kee, W.-S., Kim, B.C., Hwang, J.H., Song, K.-Y. and Kihm, Y.-H., 2007, Structural Characteristics of Quaternary reverse faulting on the Eupcheon Fault, SE Korea. Journal of the Geological Society of Korea, 43, 311-333 (in Korean with English abstract).
- Kee, W.-S., Kim, S.W., Kim, H., Hong, P., Kwon, C.W., Lee, H.-J., Cho, D.-L., Koh, H.J., Song, K.-Y., Byun, U.H., Jang, Y. and Lee, B.C., 2019, Geologic Map of Korea (1:1,000,000). Korea Institute of Geoscience and Mineral Resources.
- Kim, D.-E. and Seong, Y.B., 2021, Cumulative slip rate of the Southern Yangsan fault from geomorphic indicator and numerical dating. Journal of the Korean Geographical Society, 56, 201-213 (in Korean with English abstract).
-
Kim, J., Woo, J.-U., Rhie, J. and Kang, T.-S., 2017, Automatic determination of first-motion polarity and its application to focal mechanism analysis of microseismic events. Geosciences Journal, 21, 695-702.
[https://doi.org/10.1007/s12303-017-0022-8]
- Kim, J.H., Lee, J.Y. and Kee, W.S., 1994, Structural evolution of the Cretaceous Puyeo Basin, Korea. Journal of the Geological Society of Korea, 30, 182-192 (in Korean with English abstract).
-
Kim, K.-H., Ree, J.-H., Kim, Y., Kim, S., Kang, S.Y. and Seo, W., 2018, Assessing whether the 2017 Mw 5.4 Pohang earthquake in South Korea was an induced event. Science, 360, 1007-1009.
[https://doi.org/10.1126/science.aat6081]
- Kim, M.J., 2018, Activity features of the Keumwang Fault in the Inje-gun area, Gangwon-do. M.S. thesis, Kangwon National University, Chuncheon (in Korean with English abstract).
-
Kim, M.-J. and Lee, H.K., 2016, Internal Structure and Movement History of the Keumwang Fault. Journal of Petrology Society of Korea, 25, 211-230 (in Korean with English abstract).
[https://doi.org/10.7854/JPSK.2016.25.3.211]
-
Kim, M.J. and Lee, H.K., 2017, Quaternary activity patterns of the Keumwang Fault in the Wonju-si area, Gangwon-do. Journal of the Geological Society of Korea, 53, 79-94 (in Korean with English abstract).
[https://doi.org/10.14770/jgsk.2017.53.1.79]
-
Kim, M.-J. and Lee, H.K., 2020, ESR dating of fault gouge - review. Journal of the Geological Society of Korea, 56, 211-234 (in Korean with English abstract).
[https://doi.org/10.14770/jgsk.2020.56.2.211]
-
Kim, N., Choi, J.-H., Park, S.-I., Lee, T.-H. and Choi, Y., 2020b, Cumulative offset analysis of the Central-Southern Yangsan Fault based on topography of Quaternary fluvial terrace. Journal of the Geological Society of Korea, 56, 135-154 (in Korean with English abstract).
[https://doi.org/10.14770/jgsk.2020.56.2.135]
-
Kim, S.W., Oh, C.W., Ryu, I.-C., Williams, I.S., Sajeev, K., Santosh, M. and Rajesh, V.J., 2006, Neoproterozoic Bimodal Volcanism in the Okcheon Belt, South Korea, and Its Comparison with the Nanhua Rift, South China: Implications for Rifting in Rodinia. The Journal of Geology, 114, 717-733.
[https://doi.org/10.1086/507616]
- Kim, Y.-S. and Jin, K., 2006, Estimated earthquake magnitude from the Yugye Fault displacement on a trench section in Pohang, SE Korea. Journal of the Geological Society of Korea, 42, 79-94 (in Korean with English abstract).
- Kim, Y.-S., Jin, K., Choi, W.-H. and Kee, W.-S., 2011, Understanding of active faults: A review for recent researches. Journal of the Geological Society of Korea, 47, 723-752 (in Korean with English abstract).
-
Kim, Y.-S., Son, M., Choi, J.-H., Choi, J.-H. and Lee, J., 2020a, Processes and challenges for the production of Korean active faults map. Journal of the Geological Society of Korea, 56, 113-134 (in Korean with English abstract).
[https://doi.org/10.14770/jgsk.2020.56.2.113]
-
Klinger, Y., Choi, J.-H. and Vallage, A., 2017, Fault Branching and Long-Term Earthquake Rupture Scenario for Strike-Slip Earthquakes. Fault Zone Dynamic Processes, 217-228.
[https://doi.org/10.1002/9781119156895.ch11]
-
Klinger, Y., Etchebes, M., Tapponnier, P. and Narteau, C., 2011, Characteristic slip for five great earthquakes along the Fuyun fault in China. Nature Geoscience, 4, 389-392.
[https://doi.org/10.1038/ngeo1158]
- KMA (Korean Meteorological Administration), 2014, Historical Earthquake Records in Korea. Korean Meteorological Archives Series 5, 292.
- KMA, 2022, https://www.weather.go.kr/w/eqk-vol/search/korea.do, (October 30, 2022).
-
Kurtz, R., Klinger, Y., Ferry, M. and Ritz, J.-F., 2018, Horizontal surface-slip distribution through several seismic cycles: The Eastern Bogd fault, Gobi-Altai, Mongolia. Tectonophysics, 734-735, 167-182.
[https://doi.org/10.1016/j.tecto.2018.03.011]
-
Kwak, D., Kim, S., Sheen, D.-H. and Kim, S., 2022, Seismological characteristics of microearthquake sequence near Suncheon, South Korea, from 2009 to 2020. Geosciences Journal, 26, 609-620.
[https://doi.org/10.1007/s12303-022-0010-5]
- Kwon, B.-D., Lee, H., Lee, C.-K., Park, G. and Oh, S., 2003, Electric and Electromagnetic Surveys of the Hongseong Fault. Journal of Korean Earth Science Society, 24, 361-368 (in Korean with English abstract).
- Kwon, Y.I. and Jin, M.S., 1974, Geologic map of Cheongju sheet (1:50,000). Korea Research Institute of Geoscience and Mineral Resources.
- Kyung, J.B., 2003, Paleoseismology of the Yangsan Fault, southeastern part of the Korean Peninsula. Annals of Geophysics, 46, 983-996.
-
Lambiase, J.J. and Bosworth, W.P., 1995, Structural development and stratigraphy of the Kyokpo pull-apart basin, South Korea and tectonic implications for inverted extensional basins. In: Buchanan, J.G. and Buchanan, P.G. (eds.), Basin Inversion. The Geological Society of London, Special Publication, 88, 457-471.
[https://doi.org/10.1144/GSL.SP.1995.088.01.24]
- Lee, B.J. and Kim, B.C., 2003, Geology and structural development of the Cretaceous Gongju Basin, Korea. Journal of the Geological Society of Korea, 39, 161-170 (in Korean with English abstract).
- Lee, B.-J., Kim, D.-H., Choi, H.-I., Kee, W.-S. and Park, K.-H., 1996, Geological report of the Daejeon sheet (1:250,000). Korea Institute of Geology, Mining and Materials, 59 p (in Korean with English abstract).
- Lee, C.H. and Kim, J.H., 1971, Geologic map of Korea, Jeungpyeong Sheet (1:50,000). Geological Survey of Korea, 21 p.
- Lee, H.K., 1998, Structural analysis of the Cretaceous Pungam Basin. Journal of the Geological Society of Korea, 34, 122-136 (in Korean with English abstract).
- Lee, H.K., 2010, Structural features of the Keumwang fault zone at Sangnam-myon, Inje-gun, Gangwon-do. Journal of the Geological Society of Korea, 46, 561-576 (in Korean with English abstract).
-
Lee, H.K. and Schwarcz, E.P., 1993, An experimental study of shear-induced zeroing of ESR signals in quartz. Applied Radiation and Isotopes, 44, 191-195.
[https://doi.org/10.1016/0969-8043(93)90218-Y]
-
Lee, H.-K. and Schwarcz, E.P., 2001, ESR dating of the subsidiary faults in the Yangsan fault system, Korea. Quaternary Science Reviews, 20, 999-1003.
[https://doi.org/10.1016/S0277-3791(00)00055-X]
-
Lee, H.K. and Yang, J.S., 2003, ESR dating of the Wangsan fault, South Korea. Quaternary Science Reviews, 22, 1339-1343.
[https://doi.org/10.1016/S0277-3791(03)00018-0]
-
Lee, J., Rezaei, S., Hong, Y., Choi, J.-H., Choi, J.-H., Choi, W.-H., Rhee, K.-W. and Kim, Y.-S., 2015, Quaternary fault analysis through a trench investigation on the northern extension of the Yangsan fault at Dangu-ri, Gyungjusi, Gyeongsanbuk-do. Journal of the Geological Society of Korea, 51, 471-485 (in Korean with English abstract).
[https://doi.org/10.14770/jgsk.2015.51.5.471]
- Lee, K, Jeong, B. and Kim, K.H., 1986, Seismicity of the Korean peninsula (I): Seismicity of the Hongsung Area. Journal of the Geological Society of Korea, 22, 164-175.
-
Lee, Y., Cheon, Y., Ha, S., Kang, H.-C., Choi, J.-H. and Son, M., 2017, Geometric and kinematic characteristics of the Quaternary fault at Seooe site, in Goseong-gun, Gyeongsangnam-do. Journal of the Geological Society of Korea, 53, 115-127 (in Korean with English abstract).
[https://doi.org/10.14770/jgsk.2017.53.1.115]
-
Lim, H., Kim, Y., Kwon, K.B., Han, J., Ahn, B.S., Chai, G., Choi, Y., Heo, D., Jung, Y., Kang, H., Koh, M.H., Kwon, J., Park, J.Y., Seo, M.-S., Son, Y.O., Han, S., Kang, T.-S., Rhie, J., Kim, K.-H. and Ree, J.-H., 2021, Deployment of the linear array with geophones on the fault zone of the 2016 Mw 5.5 Gyeongju earthquake. Journal of the Geological Society of Korea, 57, 741-746 (in Korean with English abstract).
[https://doi.org/10.14770/jgsk.2021.57.5.741]
-
Long, J.J. and Imber, J., 2010, Geometrically coherent continuous deformation in the volume surrounding a seismically imaged normal fault-array. Journal of Structural Geology, 32, 222-234.
[https://doi.org/10.1016/j.jsg.2009.11.009]
-
McGill, S.F. and Sieh, K., 1991, Surficial offsets on the Central and Eastern Garlock Fault associated with prehistoric earthquakes. Journal of Geophysical Research, 96, 21597-21621.
[https://doi.org/10.1029/91JB02030]
-
Milliner, C.W.D., Dolan, J.F., Hollingsworth, J., Leprince, S., Ayoub, F. and Sammis, C.G., 2015, Quantifying near-field and off-fault deformation patterns of the 1992 Mw 7.3 Landers earthquake. Geochemistry, Geophysics, Geosystems, 16, 1577-1598.
[https://doi.org/10.1002/2014GC005693]
- Min, G.H., 2020, Structural features and activity patterns of the Keumwang Fatul developed in the Saenggeuk-myeon and Gamgok-myeon, Eumseong-gun, Chungcheongbuk-do. M.S. thesis, Kangwon National University, Chuncheon (in Korean with English abstract).
-
Moeck, I., Kwiatek, G. and Zimmermann, G., 2009, Slip tendency analysis, fault reactivation potential and induced seismicity in a deep geothermal reservoir. Journal of Structural Geology, 31, 1174-1182.
[https://doi.org/10.1016/j.jsg.2009.06.012]
-
Nicol, A., van Dissen, R., Vella, P., Alloway, B. and Melhuish, A., 2002, Growth of contractional structures during the last 10 m.y. at the southern end of the emergent Hikurangi forearc basin, New Zealand. New Zealand Journal of Geology and Geophysics, 45, 365-385.
[https://doi.org/10.1080/00288306.2002.9514979]
-
Okada, A., Watanabe, M., Sato, H., Jun, M.-S., Jo, W.-R., Kim, S.-K., Jeon, J.-S., Chi, H.-C. and Oike, K., 1994, Active Fault Topography and Trench Survey in the Central Part of the Yangsan Fault, Southeast Korea. Journal of Geography, 103, 111-126 (in Japanese with English abstract).
[https://doi.org/10.5026/jgeography.103.2_111]
-
Olley, J.M., Murray, A.S. and Roberts, R.G., 1996, The effects of disequilibria in the uranium and thorium decay chains on burial dose rates in fluvial sediments. Quaternary Science Reviews, 15, 751-760.
[https://doi.org/10.1016/0277-3791(96)00026-1]
- Rees-Jones, J., 1995, Optical dating of young sediments using fine-grain quartz. Ancient TL, 13, 9-14.
-
Reimer, P.J., Austin, W.E.N., Bard, E., Bayliss, A., Blackwell, P.G., Bronk Ramsey, C., Butzin, M., Cheng, H., Edwards, R.L., Friedrich, M., Grootes, P.M., Guilderson, T.P., Hajdas, I., Heaton, T.J., Hogg, A.G., Hughen, K.A., Kromer, B., Manning, S.W., Muscheler, R., Palmer, J.G., Pearson, C., van der Plicht, J., Reimer, R.W., Richards, D.A., Scott, E.M., Southon, J.R., Turney, C.S.M., Wacker, L., Adolphi F., Büntgen, U., Capano, M., Fahrni, S.M., Fogtmann-Schulz, A., Friedrich, R., Köhler, P., Kudsk, S., Miyake, F., Olsen, J., Reinig, F., Sakamoto, M., Sookdeo, A. and Talamo, S., 2020, The IntCal20 Northern Hemisphere Radiocarbon Age Calibration Curve (0-55 cal kBP). Radiocarbon, 62, 725-757.
[https://doi.org/10.1017/RDC.2020.41]
-
Rockwell, T.K., Lindvall, S., Dawson, T., Langridge, R., Lettis, W. and Klinger, Y., 2002, Lateral Offsets on Surveyed Cultural Features Resulting from the 1999 Izmit and Düzce Earthquakes, Turkey. Bulletin of the Seismological Society of America, 92, 79-94.
[https://doi.org/10.1785/0120000809]
-
Ryang, W.H., 2003, Contrasting basin fills in a strike-slip setting, Eumsung Basin (Cretaceous), Korea. Geosciences Journal, 7, 263-275.
[https://doi.org/10.1007/BF02910293]
- Ryang, W.H., 2013, Characteristics of strike-slip basin formation and sedimentary fills and the Cretaceous small basins of the Korean Peninsula. Journal of the Geological Society of Korea, 49, 31-45 (in Korean with English abstract).
- Ryoo, C.-R., Kim, T., Cheon, Y., Kim, C.-M., Choi, Y., Kim, D.-E., Lee, H., Lee, T.-H., Lee, H., Bae, H. and Choi, J.-H., 2022, Active fault in the northeastern part of the Gongju Fault zone around Munam-ri, Umseong, Korea: Baekmaryeong Fault. Proceedings of the Annual Joint Conference, the Petrological Society of Korea and the Mineralogical Society of Korea, Seoul, June 15, 20-21.
-
Sheen, D.-H., 2021, Analysis of the 2020 Haenam, Korea, earthquake sequence. Geoscience Journal, 25, 33-42.
[https://doi.org/10.1007/s12303-020-0038-3]
- Shin, E.J., 2021, Study on the Source Parameters of Earthquakes Occurred in Songnisan Area from 2012 to 2020. M.S. thesis, Korea National University of Education, Chung-buk, 93 p (in Korean with English abstract).
-
Shin, S., Cheon, Y., Choi, J.H., Cheong, D., Choi, S.Y., Lim, H.S., Bae, H. and Lee, H.K., 2020. Late Pleistocene sedimentary environment and reverse faulting along the Chugaryung Fault in the central Korean Peninsula: A case study on the Cheorwon Basin. Geosciences Journal, 24, 615-623.
[https://doi.org/10.1007/s12303-020-0026-7]
-
Song, S.G. and Chang, C., 2021, Dynamic earthquake rupture modeling considering regional crustal stress conditions in southeastern Korea. Geosciences Journal, 25, 211-222.
[https://doi.org/10.1007/s12303-020-0015-x]
-
Song, Y., Ha, S., Lee, S., Kang, H.-C., Choi, J.-H. and Son, M., 2020, Quaternary structural characteristics and paleoseismic interpretation of the Yangsan Fault at Dangu-ri, Gyeongju-si, SE Korea, through trench survey. Journal of the Geological Society of Korea, 56, 155-173 (in Korean with English abstract).
[https://doi.org/10.14770/jgsk.2020.56.2.155]
-
Wells, D.L. and Coppersmith, K.J., 1994, New Empirical Relationships among Magnitude, Rupture Length, Rupture Width, Rupture Area, and Surface Displacement. Bulletin of the Seismological Society of America, 84, 974-1002.
[https://doi.org/10.1785/BSSA0840040974]
-
Weon, H.J. and Lee, H.K., 2018, ESR age and characteristic features of the Keumwang Fault developed in the Ssangjeong-ri, Eumseong-gun, Chungcheongbuk-do, Korea. Journal of the Geological Society of Korea, 54, 335-357 (in Korean with English abstract).
[https://doi.org/10.14770/jgsk.2018.54.4.335]
- Yun, K.-H., 2008, Fault rupture directivity of Odaesan Earthquake (M=4.8, '07. 1. 20). Geophysics and Geophysical Exploration, 11, 137-147 (in Korean with English abstract).
-
Zielke, O., Arrowsmith, J.R., Ludwig, L.G. and Akçiz, S.O., 2010, Slip in the 1857 and Earlier Large Earthquakes Along the Carrizo Plain, San Andreas Fault. Science, 327, 1119-1122.
[https://doi.org/10.1126/science.1182781]