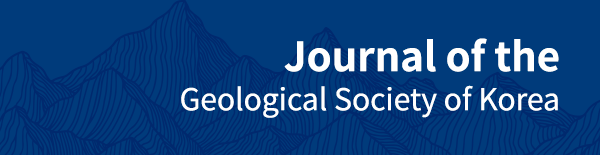
EA-IRMS와 HT-EA-IRMS를 이용한 황산염의 황과 산소동위원소 온라인 분석법


Copyright © 2017 The Geological Society of Korea
This is an Open Access article distributed under the terms of the Creative Commons Attribution Non-Commercial License (http://creativecommons.org/licenses/by-nc/3.0/ which permits unrestricted non-commercial use, distribution, and reproduction in any medium, provided the original work is properly cited.
초록
자연계에서 쉽게 관찰되는 주요 음이온인 황산염(SO4)에 대한 황과 산소 동위원소 비(δ34S와 δ18O)는 인위적 오염물질과 자연기원 물질을 추적할 수 있는 효과적인 도구로 사용되어 왔다. 국내에서는 진공 전처리장치를 이용하여 이산화황 기체를 모은 후 동위원소질량분석기로 황 동위원소만을 측정할 수 있는 분석 방법이 이용되어 왔다. 최근 원소분석기와 동위원소질량분석기를 직접 연결한 자동화시스템이 한국기초과학지원연구원에 구축되어 황산염의 황과 산소의 동위원소 분석이 가능해졌다. 이 연구에서는 황산염의 황 동위원소 분석을 위한 EA-IRMS와 산소 동위원소 분석을 위한 HT-EA-IRMS의 온라인 분석법에 대한 신뢰성 검증을 실시하였다. 이를 위해 서로 다른 동위원소 비를 갖는 3종의 국제 공인 표준물질인 NBS-127 (δ34S=20.3±0.4‰, δ18O= 8.7±0.2‰), IAEA-SO5 (δ34S=0.5±0.2‰, δ18O=12.0±0.2‰)와 IAEA-SO6 (δ34S=–34.1±0.2‰, δ18O=–11.3± 0.2‰)에 대한 반복분석을 수행하였다. NBS-127, IAEA-SO5와 IAEA-SO6에 대해 분석된 황 동위원소 비는 각각20.3±0.1‰, 0.5±0.2‰와–34.1±0.2‰로 측정되었으며, 산소 동위원소 비는 각각 8.7±0.2‰, 12.0±0.2‰와 –11.3±0.2‰로 측정되어 국제 공인 표준물질의 인증 값과 통계적으로 유의미한 차이가 없음을 확인하였다. 이번 연구에서 확립한 황산염의 황과 산소의 동위원소에 대한 온라인 분석법은 향후 국내의 황 순환 연구 및 기원 추적 연구에 도움이 될 것으로 기대된다.
Abstract
Sulfate (SO4) is ubiquitous in environment, and its dual isotopic compositions (δ34S and δ18O) have been efficiently used to trace natural and anthropogenic sources. For SO2 gas stripped from a sample using a vacuum-extraction line, δ34S value has been only analyzed by a dual-inlet isotope ratio mass spectrometry (DI-IRMS) in South Korea. In this study, we first introduce an on-line analytical method to determine δ34S and δ18O values of sulfate using an elemental analyzer (EA) interfaced with IRMS and with a high temperature conversion-EA-IRMS (HT-EA-IRMS) installed in the Korea Basic Science Institute. Three international reference materials, NBS-127 (δ34S=20.3±0.4‰, δ18O=8.7±0.2‰), IAEA-SO5 (δ34S=0.5±0.2‰, δ18O=12.0±0.2‰) and IAEA-SO6 (δ34S=–34.1±0.2‰, δ18O=–11.3±0.2‰), were utilized to evaluate an analytical quality for the on-line method. The measured isotopic values for NBS-127 (δ34S=20.3±0.1‰, δ18O=8.7±0.2‰), IAEA-SO5 (δ34S=0.5±0.2‰, δ18O=12.0±0.2‰) and IAEA-SO6 (δ34S=–34.1±0.2‰, δ18O=–11.3±0.2‰) were in agreement with the assigned values within the acceptable error range. We expect that the analytical method helps the isotopic community in Korea studying the sulfur cycle and sulfate sources in various environments.
Keywords:
sulfur isotope, oxygen isotope, on-line analytical method, EA-IRMS, HT-EA-IRMS키워드:
황 동위원소, 산소 동위원소, 온라인 분석법, EA-IRMS, HT-EA-IRMS1. 서 론
황은 광화작용(mineralization), 황화광물의 산화(oxidation of sulfide), 박테리아에 의한 황산염 환원(bacterial sulfate reduction), 침출(leaching) 등과 같은 변형 현상(transformation processes)을 포함 하는 황 순환(sulfur cycle) 과정을 거침으로써 다양한 화합물(e.g., elemental sulfur, sulfide, sulfate) 형태로 자연계에 존재한다. 특히 황산염(SO4)은 산화 환경에 존재하는 주요 음이온으로써 황의 주요 근원물질과 변형 현상에 따라 황 동위원소 비(δ34S)가 결정된다(Thode, 1991). 따라서 다양한 인위적 오염물질에 대한 황 동위원소 비를 조사함으로써 오염 물질 및 자연기원 물질이 환경에 미치는 영향을 효율적으로 파악할 수 있다. 예를 들어, Vitòria et al. (2004)에 의해 정리된 화학비료의 황 동위원소 비(–6.5‰~21.4‰)는 많은 연구에서 참고자료로 사용되고 있으며, Hosono et al. (2007)은 오폐수의 황 동위원소 비를 이용하여 하천수에 미치는 인위적 오염물질의 기여도를 측정하였다. 국내에서는 Shin et al. (2015)이 도심 하천에 대한 인위적 오염물질의 기여도를 측정하기 위하여 세제, 화학비료 및 오폐수의 황 동위원소 비를 이용하였다. 또한 산성 광산 배수(acid mine drainage)가 하천의 수리 화학적 특성에 미치는 영향을 산정하는 연구에도 황 동위원소 비가 효과적인 방법으로 사용되고 있다(Edraki et al., 2005; Migaszewski et al., 2013; Shin et al., 2014). 한편, 뚜렷한 동위원소분별 현상 없이 근원물질 사이에서 일어나는 황 화합물의 이동 및 형태변화는 서로 다른 근원물질로부터 기원한 황이 같은 동위원소 범위를 나타내는 결과를 초래한다. 예를 들어, 토양 내에 분포하는 황산염이 대기에 존재하는 황산염으로부터 기원하게 되면 토양 황산염과 대기 기원 황산염은 비슷한 황 동위원소 범위(–2‰~10‰)를 나타낸다(Krouse and Mayer, 2000).
황산염 중 황 원소가 뚜렷한 근원물질로부터 기원하는 것과 달리 산소 원소는 주로 황산염이 형성될 당시 주변 환경에 존재하는 기체 산소 및 물로부터 기원한다. Holt and Kumar (1991)에 따르면 이산화황 기체가 물과 만나 동위원소 평형상태에 도달하게 되면 이때 생성되는 황산염의 산소 4개중 3개는 물로부터 기원하며, 황화광물 산화에 의해 생성된 황산염의 산소 동위원소 비는 반응에 참여한 물과 기체의 산소에 의해 결정된다(Taylor et al., 1984). 또한 환원환경에서 황화광물은 질산염에 의해 산화되어 황산염을 생성하기도 하며(예: 5FeS2+14NO3-+ 4H+→5Fe2++10SO42-+7N2+2H2O, Hiscock et al., 1991), 환원환경에서 박테리아에 의한 황산염의 환원은 남아 있는 황산염의 산소 동위원소 비에 변화를 초래한다(Mayer, 2005). 따라서 황산염의 산소 동위원소 비(δ18O)를 황 동위원소 비와 함께 측정함으로써 보다 정확한 황산염에 대한 근원물질을 밝히고 생성기작을 설명할 수 있다(Taylor et al., 1984; Brunner et al., 2005).
2000년대 이전까지만 하더라도 황 동위원소 분석은 다양한 전처리 방식에 따라 순수한 이산화황 기체(SO2)를 포집하는 과정을 거쳤다. Holt and Engelkemeir (1970)와 Bailey and Smith (1972)는 석영관(quartz tube) 안에 넣은 시료를 약 800℃에서 연소시킨 후 진공라인에서(~0.1 mtorr) 어는점을 이용하여 순수한 이산화황 기체를 포집하였다(약 75% 회수율, De Groot, 2004). Yanagisawa and Sakai (1983)은 V2O5와 SiO2를 시료(예: BaSO4)와 함께 연소시킴으로써 이산화황 기체의 회수율을 95%~99%까지 높였으며, 보다 낮은 온도(~1,000℃)에서도 시료의 완전한 연소가 일어날 수 있는 방법을 제시하였다; V2O5를 사용하지 않고 BaSO4와 SiO2를 혼합하여 연소시킬 경우 <1,400℃의 온도에서 반응이 잘 일어나지 않는다. 또한 암석 및 광물시료의 경우 280℃의 온도로 유지되는 진공관에서 Kiba reagent (Sn2+를 포함하는 인산, Kiba et al., 1955)와 함께 연소시킨 후 어는점을 이용하여 이산화황 기체를 포집하였다(Ueda and Sakai, 1983); 전처리 과정 중 발생하는 H2S 기체는 산화제인 Cu2O와 반응하여 이산화황 기체로 변환된다. 이와 같이 전처리 과정을 거쳐 포집된 이산화황 기체는 동위원소질량분석기(isotope ratio mass spectrometry, IRMS)에서 표준 기체(reference gas)와 교대로 측정되는 DI-IRMS (dual inlet-IRMS) 방식에 의해 동위원소 비가 결정 된다(Halas, 1979; Meijer et al., 2000; Halas and Szaran, 2001). 산소 동위원소 비는 흑연(graphite)과 황산염 시료를 약 1,000℃의 고온에서 반응시켜 이산화탄소(CO2)를 생성하는 전처리 과정을 거친 다음 동위원소 비를 측정하는 탄소 환원법(carbon reduction method)이 주로 사용되었다(Lloyd, 1967; Longinelli and Craig, 1967; Rafter, 1967; Mizutani, 1971; Sakai and Krouse, 1971).
전처리 과정 없이 원소분석기(elemental analyzer, EA)에서 분석 대상 시료를 연소시켜 발생하는 이산화황 기체를 동위원소질량분석기로 유입시켜 황 동위원소 비를 분석할 수 있는 온라인 분석법(on-line method)은 Pichlmayer and Blochberger (1988)에 의해 처음 개발되었다. 온라인 분석법을 이용하여 토양과 식물에 대한 황 동위원소 비가 Giesemann et al. (1994)에 의해 보고된 이후 대부분의 황 동위원소 관련 연구는 온라인 방식을 채택하고 있다. 산소 동위원소 비는 ~1,500℃의 고온으로 유지되는 high temperature conversion-elemental analyzer (HT-EA)에서 시료를 연소시켜 일산화탄소(CO)를 생성시킨 후 동위원소질량분석기에서 동위원소 비를 측정할 수 있는 온라인 분석법이 1990년대 후반에 보고되었다(Kornexl et al., 1999; Morrison, 1999).
전처리 과정을 거쳐 준비된 이산화황 기체를 분석하는 DI-IRMS 방식은 전처리에 많은 시간과 노동력을 소모할 뿐만 아니라 CF (continuous flow) 방식으로 이루어지는 온라인 분석법(<400 μg, BaSO4)에 비해 상대적으로 많은 양의 시료(~10 mg, BaSO4)가 필요하다(Mayer and Krouse, 2004; Mayer, 2005). 산소 동위원소 비를 측정하기 위하여 복잡한 전처리 과정을 거쳐 포집한 이산화탄소는 전처리 과정 중 사용되는 석영관의 산소성분과 고온에서 동위원소적인 교환반응을 하게 되어 재현성 있는 산소 동위원소 값을 얻기 어렵다(Sakai and Krouse, 1971). 이와 대조적으로 전처리 과정 없이 산소 동위원소를 분석할 수 있는 온라인 분석법의 경우 시료 이외의 산소 공급원이 없기 때문에 보다 정확한 산소 동위원소 비를 쉽게 얻을 수 있다.
이에 본 연구에서는 최근 한국기초과학지원연구원에서 황산염의 황과 산소 동위원소 비 분석을 위해 운용되고 있는 EA-IRMS와 HT-EA-IRMS의 분석 자료에 대한 신뢰도를 확보하기 위해 3종의 국제표준물질인 NBS-127, IAEA-SO5, IAEA-SO6에 대한 반복분석 결과를 소개하고자 한다.
2. 분석방법
2.1 분석 시료 준비
황산염 이온의 황과 산소의 동위원소 분석법을 검증하기 위하여 서로 다른 동위원소 비를 갖는 국제표준물질인 황산바륨(BaSO4) 3종 NBS-127 (δ34S=20.3± 0.4‰, δ18O=8.7±0.2‰), IAEA-SO5 (δ34S=0.5±0.2‰, δ18O=12.0±0.2‰), IAEA-SO6 (δ34S=–34.1±0.2‰, δ18O=–11.3±0.2‰)과 실험실표준물질(BaSO4, δ34S= 5.82‰)을 사용하였다. 원소분석기와 온라인으로 연결된 동위원소질량분석기를 이용하여 동위원소 비를 분석하는 경우, 시료의 양과 동위원소 비는 일정한 함수관계를 보인다(Avak and Fry, 1999; Fry, 2007). 따라서 시료의 양에 따른 황 동위원소 비 변화에 대한 값을 보정하기 위하여 실험실표준물질을 약 50~1,000 μg 범위에서 양을 달리하여 준비하였다. 황 동위원소 분석을 위해 초정밀 저울을 이용하여 시료 400~500 μg을 주석 캡슐(tin capsule, 3×5 mm, Thermo scientific)에 담아 준비하였으며, 산소 동위원소 측정을 위한 시료는 은 캡슐(silver capsule, 3×5 mm, Thermo scientific)에 200~300 μg을 담아 준비하였다. 캡슐에 싸인 시료는 분석 전까지 데시케이터(desiccator)에 보관하여 건조 상태를 유지하였다.
2.2 황 동위원소
황 동위원소는 한국기초과학지원연구원에서 보유하고 있는 elemental analyzer (EA, vario PYRO cube, Elementar)와 직접 연결된 동위원소질량분석기(Isoprime 100, Isoprime Ltd)를 이용하여 분석하였다. 시료는 원소분석기에 설치된 자동 시료주입기(auto-sampler)를 통해 1,150℃의 온도로 유지되는 연소로 내에 설치된 연소관(combustion tube)에 주입된다(그림 1a). 촉매제로 이용되는 삼산화텅스텐(WO3)이 채워져 있는 연소관에 산소기체가 주입됨으로써 시료는 원활하게 연소되어 이산화황 기체로 산화된다(Fourel et al., 2014). 이산화황 기체는 운반 기체(carrier gas, He)와 함께 연소관과 연결된 환원관(reduction tube, 850℃)으로 이동된다. 환원관에 채워져 있는 환원구리는 연소관에서 시료의 연소 시 발생할 수 있는 삼산화황(SO3)을 이산화황으로 변환시켜줌으로써 동위원소 비 측정에 영향을 줄 수 있는 삼산화황의 영향을 제거하게 된다(Ueda and Krouse, 1986). 또한 환원관에 실버 울(silver wool)을 넣어 시료 전처리과정(예: 염산과 염화바륨을 이용한 황산바륨 침전) 중 시료에 포함되어 있을 수 있는 염소를 제거하였다. 이후 수분제거장치(water trap, SICAPENTTM)를 거친 뒤, 220℃로 유지되는 이산화황 탈착관(desorption unit)에서 순수한 이산화황 기체가 배출되어 동위원소질량분석기로 유입된 후 동위원소 비가 측정된다(그림 1a). 황 동위원소 비는 다음 식 (1)과 같이 VCDT (Vienna Canyon Diablo Troilite)에 상대적인 비교를 통한 델타 표시법(δ-notation)을 적용하였으며, 단위는 천분율(‰)로 나타내었다.
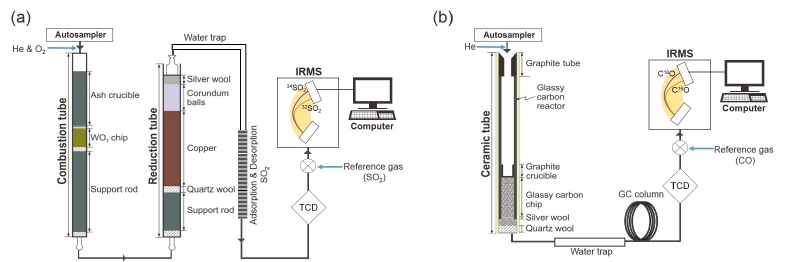
Schematic diagrams showing the analytical processes to determine δ34S and δ18O values of sulfate. δ34S (a) and δ18O (b) values were analyzed by an isotope ratio mass spectrometry interfaced with an elemental analyzer and with a high temperature conversion-elemental analyzer, respectively.
(1) |
2.3 산소 동위원소
산소 동위원소 분석은 한국기초과학지원연구원에서 운용되고 있는 high temperature conversion-elemental analyzer (HT-EA, Flash 2000, Thermo scientific)와 직접 연결된 동위원소질량분석기(Thermo delta V advantage, Thermo scientific)를 사용하였다. 시료는 원소분석기에 설치된 자동 시료주입기(No Blank Sampling Device, Thermo scientific)를 통해 대기 기체와 완전히 차단된 상태에서 1,450~1,500℃의 온도로 유지되는 연소로의 연소관으로 주입된다; Kornexl et al. (1999)에 따르면 1,050~1,300℃의 온도에서 촉매제(예: 니켈)와 반응시켜 무기물 시료를 연소시킬 경우 반응속도가 느릴 뿐만 아니라 생성되는 CO 피크의 꼬리끌림(tailing) 현상이 발생하여 재현성 있는 데이터를 얻기 어렵다. 연소관은 외부의 세라믹관과 과량의 탄소 조각(glassy carbon chip)으로 채워진 흑연관(glassy carbon reactor)으로 구성되어 있다(그림 1b); 높은 온도에서 세라믹관(ceramic tube, Al2O3)의 산소 성분은 분석대상물질의 산소 동위원소 비에 영향을 줄 수 있기 때문에 흑연관을 이용하여 산소의 영향을 차단하였다(Prot and Monty, 1996; Kornexl et al., 1999). 탄소조각은 황산염시료가 연소될 때 이산화탄소의 생성을 방지함으로써 시료를 모두 일산화탄소 기체로 전환시킨다. 연소과정에서 발생한 일산화탄소 기체는 수분제거장치와 GC (gas chromatography) 컬럼을 거치게 됨으로써 순수한 일산화탄소 기체만 연결된 동위원소질량분석기로 주입된다(그림 1b). 산소 동위원소 비는 다음 식 (2)와 같이 VSMOW (Vienna Standard Mean Ocean Water)에 상대적인 비교를 통한 델타 표시법(δ-notation)을 적용하였으며, 단위는 천분율(‰)로 나타내었다.
(2) |
2.4 동위원소 비 보정
이 실험에서 사용된 시료의 양과 동위원소 비 사이에 뚜렷한 함수관계가 관찰되지 않았다. 예를 들어, 기기 측정 시 시료의 양에 따라 일정하게 증가하는 peak height와 황 동위원소 조성은 δ34S=0.0008× (peak height)+5.8195 (R2=0.0002)의 함수식으로 표현되었다. 시료의 양을 달리하여 측정한 황 동위원소 값은 5.78‰~5.85‰ (평균 5.82±0.02‰, n=7)의 상당히 좁은 범위에서 변화하였다.
따라서 시료 양에 따른 동위원소 값의 변화는 없는 것으로 판단하였으며, 국제 표준 시료 3종(IAEA-SO5, IAEA-SO6와 NBS-127)의 공인된 값과 측정값과의 관계만을 이용하여 동위원소 값을 보정하였다. 황 동위원소의 경우 IAEA에서 제공하고 있는 각 시료에 대한 공인 값을 사용하였다.
산소 동위원소의 경우 표준시료를 제공하고 있는 IAEA에서 IAEA-SO5와 IAEA-SO6의 산소 동위원소 비에 대한 공인 값을 제공하고 있지 않고 있기 때문에 이전 연구의 반복분석에 의해 범용적으로 사용되는 값을 공인된 값으로 간주하였다(Böhlke et al., 2003). 또한 NBS-127의 산소 동위원소 비는 IAEA에서 제공하는 공인 값(9.3±0.4‰)이 아닌 이전 연구에서 온라인 분석법에 의해 보고된 값 8.7‰ (Kornexl et al., 1999)을 사용하였다. 이에 대한 이유는 아래에 자세히 기재하였다.
IAEA에서 제공하고 있는 NBS-127에 대한 산소 동위원소 비는 오프라인 방식에 의해 분석된 9.3±0.4‰ (Gonfiantini et al., 1995)을 공인 값으로 채택하고 있다. 이전 연구에서 오프라인 방식을 이용하여 분석한 NBS-127의 산소 동위원소 비 또한 공인 값과 비슷한 값(8.9‰~9.5‰)으로 보고되었다(Hall et al., 1991; Gonfiantini et al., 1995; Böttcher et al., 1999; Palmer et al., 2004). 아직까지 IAEA에서 공식적으로 HT-EA-IRMS를 이용하여 분석한 산소 동위원소 값을 제공하고 있지 않다. 최근 HT-EA-IRMS의 개발과 함께 NBS-127에 대한 산소 동위원소 값이 많은 이전 연구에서 8.5‰~8.7‰의 범위로 새롭게 보고되었다(Kornexl et al., 1999; Böhlke et al., 2003; Boschetti and Iacumin, 2005). 오프라인 방식으로부터 분석한 NBS-127의 산소 동위원소 비와 온라인 방식으로부터 획득한 분석 값에 대한 차이는 (1) 오프라인 방식에서 CO가 CO2로 산화될 때 발생하는 kinetic isotope effect, (2) 오프라인 방식에서 quartz/ pyrex와 CO 사이의 산소 동위원소 교환 (3) 시료의 오염 등과 같은 원인이 제시되었다(Boschetti and Iacumin, 2005).
3. 결과
3.1 황 동위원소 비
국제 공인 표준시료 3종 NBS-127, IAEA-SO5와 IAEA-SO6은 각각 20.2‰~20.4‰ (20.3±0.10‰, n=9), 0.1‰~0.9‰ (0.5±0.2‰, n=10)와 –34.1‰~–33.8‰ (–34.1±0.2‰, n=9)의 황 동위원소 값으로 분석되었다(그림 2a). 분석된 표준시료의 신뢰도를 검증하기 위하여 분석 값과 공인 값에 대하여 z-test를 실시하였으며, 통계적으로 두 값이 차이가 없는 것으로 나타났다(p-value >0.05). 서로 다른 황 동위원소 값을 갖는 국제 공인 표준시료 3종에 대해 공인된 값과 측정된 값은 일정한 선형 함수 관계를 보이고 있다(R2=0.999, 그림 2b). 이는 다양한 황 동위원소 값의 범위를 갖는 분석시료에 대한 온라인 분석법 적용이 성공적임을 나타낸다.
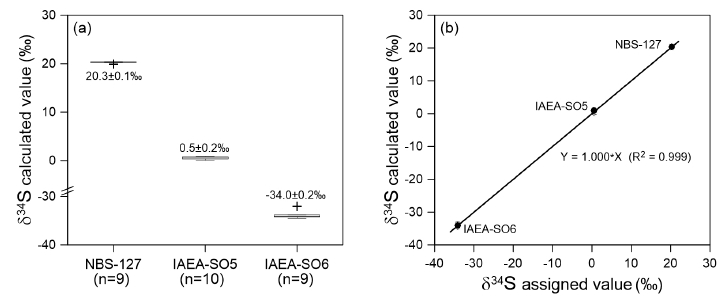
A range of calculated δ34S values for three international standard materials (NBS-127, IAEA-SO5 and IAEA-SO6) (a) and relationship between their assigned values and calculated values (b).
동일한 시료에 대하여 분석을 수행한 이전 연구들에서 제시한 분석 값과 이 실험에서 측정한 분석 값을 표 1에 제시하였다. 이 실험에서 측정된 NBS-127, IAEA-SO5와 IAEA-SO6의 분석 값은 대부분 이전 연구에서 보고된 분석 값과 95% 신뢰수준 내에서 일치한다. 즉, NBS-127과 IAEA-SO5에 대한 분석 값은 Christensen et al. (2007) (각각 20.3±0.2‰과 0.48± 0.2‰)과 Fourel et al. (2014)에 의해 보고된 분석 값(각각 20.6±0.1‰과 0.5±0.2‰)에 대해 95% 신뢰수준 내에서 일치하였다. 또한 IAEA-SO6에 대한 분석 값의 경우 이전 연구의 결과들과 비슷하게 측정되었다(–34.12±0.11‰, Halas and Szaran, 2001; –34.2±0.2‰, Christensen et al., 2007; –33.9±0.3‰, Fourel et al., 2014). 한편, Halas and Szaran (2001)에 의해 수행된 NBS-127과 IAEA-SO5의 분석은 오프라인 방식에 기반을 두고 있으며, 각 표준시료에 대한 분석 값(21.17±0.12‰와 0.15±0.06‰)은 다른 연구결과와 비교하여 95% 신뢰수준에서 벗어나 있다; 진공 전처리관에서 시료와 촉매제의 연소에 의해 발생한 SO2 기체가 동위원소질량분석기로 주입되는 방식을 이용하였다. 온라인 분석법과 오프라인 분석법이 시료에 따라 다른 분석 결과를 나타내는 것을 밝히기 위해서는 보다 많은 시료에 대한 분석이 선행된 비교연구가 요구된다.
3.2 산소 동위원소 비
NBS-127의 산소 동위원소 비는 8.4‰~8.9‰의 범위(8.7±0.2‰, n=6)를 보였으며, IAEA-SO5와 IAEA-SO6은 각각 11.6‰~12.8‰ (12.0±0.2‰, n=14)와 –11.6‰~–11.0‰의 범위(–11.3±0.2‰, n=14)를 나타내었다(그림 3a). 신뢰도를 검증하기 위하여 분석된 표준시료의 분석 값과 공인 값에 대하여 z-test를 실시하였으며, 통계적으로 두 값이 차이가 없는 것으로 나타났다(p-value >0.05). 국제 공인 표준 시료 3종에 대해 공인된 산소 동위원소 값과 이 실험에서 측정한 동위원소 값은 일정한 선형 함수 관계로 표현 되었다(R2=0.999, 그림 3b). 황 동위원소에서 제안된 것처럼 이러한 결과는 다양한 산소 동위원소 값의 범위를 갖는 분석시료에 대한 온라인 분석법 적용이 성공적임을 나타낸다.
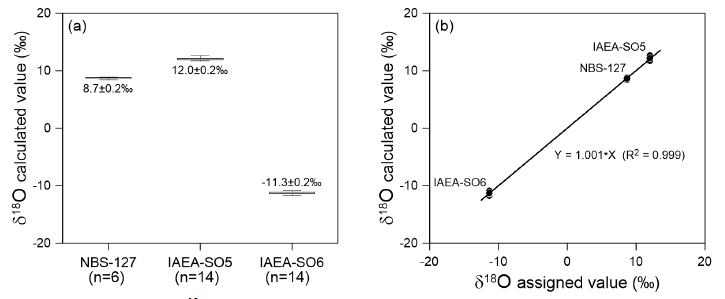
A range of calculated δ18O values for three international standard materials (NBS-127, IAEA-SO5 and IAEA-SO6) (a) and relationship between their assigned values and calculated values (b).
산소 동위원소 비 또한 동일한 시료에 대하여 분석을 수행한 이전 연구들의 결과와 함께 표 1에 제시하였다. 이 연구에서 분석한 NBS-127과 IAEA-SO5의 산소 동위원소 비는 Brand et al. (2009) (각각 8.59±0.26‰, 12.13±0.33‰), Halas et al. (2007) (각각 8.73±0.05‰, 12.20±0.07‰) 그리고 Kornexl et al. (1999) (각각 8.7±0.2‰, 12.0±0.2‰)에서 보고한 값에 대해 95% 신뢰수준 내에서 일치하는 것을 확인하였다. 또한 IAEA-SO6의 경우 Brand et al. (2009)와 Kornexl et al. (1999)의 분석 값과 95% 신뢰수준 내에서 일치하였다(각각 –11.35±0.31‰과 –11.0±0.2‰).
4. 결 론
이 연구에서는 한국기초과학지원연구원에서 운영 중인 EA-IRMS와 HT-EA-IRMS를 사용하여 황산염 성분인 국제표준시료 3종(NBS-127, IAEA-SO5, IAEA- SO6)의 황과 산소 동위원소 비에 대한 분석 결과를 제시하였다. NBS-127 (δ34S=20.3±0.1‰, δ18O=8.7±0.2‰), IAEA-SO5 (δ34S=0.5±0.2‰, δ18O=12.0±0.2‰)와 IAEA-SO6 (δ34S=–34.1±0.2‰, δ18O=–11.3±0.2‰)의 분석 결과는 보고된 표준시료 동위원소 비와 오차 범위 내에서 일치하였으며, 95% (p-value >0.05) 신뢰 수준에서 유의미한 차이가 없음을 보였다. 또한 국외에서 수행된 이전 연구들에서 동일 시료에 대해 제시된 동위원소 비와 비교하여 오차범위 내에서 대체로 다르지 않음을 확인하였다. 이러한 연구 결과로부터 한국기초과학지원연구원에서 수행되고 있는 황산염의 황과 산소 동위원소의 온라인 분석법에 대한 분석 신뢰도가 확인되었다. 황 및 산소 동위원소 온라인 분석이 가능하게 됨으로써 수권 및 토양권에서 발생하는 황산염의 기원을 추적할 수 있을 뿐만 아니라 생물권에서 일어나는 황 화합물의 변형 현상을 이해할 수 있을 것으로 판단된다. 또한 대기 오염문제를 유발시키는 주요 근원물질을 밝히는데 효과적인 도구로 사용될 수 있을 것으로 기대된다.
Acknowledgments
이 논문은 2016년 한국광해관리공단으로부터 기술개발사업비를 지원받아 수행된 사업임.
References
- Avak, H., and Fry, B., (1999), EA-IRMS: Precise and accurate measurements of δ15N on <10 μg N. Finnigan MAT Application Flash Report G, p29.
-
Bailey, S.A., and Smith, J.W., (1972), Improved method for the preparation of sulfur dioxide from barium sulfate for isotope ratio studies, Analytical Chemistry, 44(8), p1542-1543.
[https://doi.org/10.1021/ac60316a034]
- Böhlke, J.K., Mroczkowski, S.J., and Coplen, T.B., (2003), Oxygen isotopes in nitrate: New reference materials for 18O: 17O: 16O measurements and observations on nitrate-water equilibration, Rapid Communications in Mass Spectrometry, 17(16), p1835-1846.
-
Boschetti, T., and Iacumin, P., (2005), Continuous-flow δ18O measurements: New approach to standardization, high-temperature thermodynamic and sulfate analysis, Rapid Communications in Mass Spectrometry, 19(21), p3007-3014.
[https://doi.org/10.1002/rcm.2161]
-
Böttcher, M.E., Bernasconi, S.M., and Brumsack, H.J., (1999), Carbon, sulfur and oxygen isotope geochemistry of interstitial waters from the Western Mediterranean, In Zahn, R., Comas, M.C., and Klaus, A. (eds.), Proceedings Ocean Drilling Program, Scientific Results, 161, p413-421.
[https://doi.org/10.2973/odp.proc.sr.161.229.1999]
-
Brand, W.A., Coplen, T.B., Aerts-Bijma, A.T., Böhlke, J.K., Gehre, M., Geilmann, H., Gröning, M., Jansen, H.G., Meijer, H.A.J., Mroczkowski, S.J., Qi, H., Soergel, K., Stuart-Williams, H., Weise, S.M., and Werner, R.A., (2009), Comprehensive inter-laboratory calibration of reference materials for δ18O versus VSMOW using various on-line high-temperature conversion techniques, Rapid Communications in Mass Spectrometry, 23(7), p999-1019.
[https://doi.org/10.1002/rcm.3958]
-
Brunner, B., Bernasconi, S.M., Kleikemper, J., and Schroth, M.H., (2005), A model for oxygen and sulfur isotope fractionation in sulfate during bacterial sulfate reduction processes, Geochimica et Cosmochimica Acta, 69(20), p4773-4785.
[https://doi.org/10.1016/j.gca.2005.04.017]
-
Christensen, L.E., Brunner, B., Truong, K.N., Mielke, R.E., Webster, C.R., and Coleman, M., (2007), Measurement of sulfur isotope compositions by tunable laser spectroscopy of SO2, Analytical Chemistry, 79(24), p9261-9268.
[https://doi.org/10.1021/ac071040p]
- De Groot, P.A. (ed.), (2004), Handbook of stable isotope analytical techniques (Vol. 2), Elsevier.
-
Edraki, M., Golding, S.D., Baublys, K.A., and Lawrence, M.G., (2005), Hydrochemistry, mineralogy and sulfur isotope geochemistry of acid mine drainage at the Mt. Morgan mine environment, Queensland, Australia, Applied Geochemistry, 20(4), p789-805.
[https://doi.org/10.1016/j.apgeochem.2004.11.004]
-
Fourel, F., Martineau, F., Seris, M., and Lécuyer, C., (2014), Simultaneous N, C, S stable isotope analyses using a new purge and trap elemental analyzer and an isotope ratio mass spectrometer, Rapid Communications in Mass Spectrometry, 28(23), p2587-2594.
[https://doi.org/10.1002/rcm.7048]
-
Fry, B., (2007), Coupled N, C and S stable isotope measurements using a dual‐column gas chromatography system, Rapid Communications in Mass Spectrometry, 21(5), p750-756.
[https://doi.org/10.1002/rcm.2892]
-
Giesemann, A., Jäger, H.J., Norman, A.L., Krouse, H.R., and Brand, W.A., (1994), On-line sulfur-isotope determination using an elemental analyzer coupled to a mass spectrometer, Analytical Chemistry, 66, p2816-2819.
[https://doi.org/10.1021/ac00090a005]
- Gonfiantini, R., Stichler, W., and Rozanski, K., (1995), Standards and intercomparison materials distributed by the International Atomic Energy Agency for stable isotope measurements, In Staff, I. (ed.), Reference and Intercomparison Materials for Stable Isotopes of Light Elements, p13.
-
Halas, S., (1979), An automatic inlet system with pneumatic changeover valves for isotope ratio mass spectrometer, Journal of Physics E: Scientific Instruments, 12(5), p418.
[https://doi.org/10.1088/0022-3735/12/5/020]
-
Halas, S., and Szaran, J., (2001), Improved thermal decomposition of sulfates to SO2 and mass spectrometric determination of δ34S of IAEA-SO5, IAEA-SO6 and NBS-127 sulfate standards, Rapid Communications in Mass Spectrometry, 15(17), p1618-1620.
[https://doi.org/10.1002/rcm.416]
- Halas, S., Szaran, J., Czarnacki, M., and Tanweer, A., (2007), Refinements in BaSO4 to CO2 preparation and δ18O calibration of the sulfate reference materials NBS-127, IAEA-SO5 and IAEA-SO6, Geostandards and Geoanalytical Research, 31(1), p61-68.
-
Hall, A.J., Boyce, A.J., Fallick, A.E., and Hamilton, P.J., (1991), Isotopic evidence of the depositional environment of Late Proterozoic stratiform barite mineralisation, Aberfeldy, Scotland, Chemical Geology: Isotope Geoscience Section, 87(2), p99-114.
[https://doi.org/10.1016/0168-9622(91)90044-w]
-
Hiscock, K.M., Lloyd, J.W., and Lerner, D.N., (1991), Review of natural and artificial denitrification of groundwater, Water Research, 25(9), p1099-1111.
[https://doi.org/10.1016/0043-1354(91)90203-3]
-
Holt, B.D., and Engelkemeir, A.G., (1970), Thermal decomposition of barium sulfate to sulfur dioxide for mass spectrometric analysis, Analytical Chemistry, 42(12), p1451-1453.
[https://doi.org/10.1021/ac60294a032]
- Holt, B.D., and Kumar, R., (1991), Oxygen isotope fractionation for understanding the sulphur cycle, In Krouse, H.R., and Grinenko, V.A. (eds.), Stable Isotopes: Natural and Anthropogenic Sulphur in the Environment: SCOPE 43, John Wiley and Sons, Chichester, p27-41.
-
Hosono, T., Nakano, T., Igeta, A., Tayasu, I., Tanaka, T., and Yachi, S., (2007), Impact of fertilizer on a small watershed of Lake Biwa: Use of sulfur and strontium isotopes in environmental diagnosis, Science of the Total Environment, 384(1), p342-354.
[https://doi.org/10.1016/j.scitotenv.2007.05.033]
-
Kiba, T., Takagi, T., Yoshimura, Y., and Kishi, I., (1955), Tin (II)-strong phosphoric acid. A new reagent for the determination of sulfate by reduction to hydrogen sulfide, Bulletin of the Chemical Society of Japan, 28(9), p641-644.
[https://doi.org/10.1246/bcsj.28.641]
-
Kornexl, B.E., Gehre, M., Höfling, R., and Werner, R.A., (1999), On-line δ18O measurement of organic and inorganic substances, Rapid Communications in Mass Spectrometry, 13(16), p1685-1693.
[https://doi.org/10.1002/(sici)1097-0231(19990830)13:16<1685::aid-rcm699>3.3.co;2-0]
-
Krouse, H.R., and Mayer, B., (2000), Sulphur and oxygen isotopes in sulphate, In Cook, P.G., and Herczeg, A.L. (eds.), Environmental Tracers in Subsurface Hydrology, Springer Science & Business Media, p195-231.
[https://doi.org/10.1007/978-1-4615-4557-6_7]
- Lloyd, R.M., (1967), Oxygen-18 composition of oceanic sulfate, Science, 156, p1228-1231.
-
Longinelli, A., and Craig, H., (1967), Oxygen-18 variations in sulfate ions in sea water and saline lakes, Science, 156(3771), p56-59.
[https://doi.org/10.1126/science.156.3771.56]
- Mayer, B., (2005), Assessing sources and transformations of sulphate and nitrate in the hydrosphere using isotope techniques, In Aggarwal, P.K., Froehlich, K.F., and Gat, J.R. (eds.), Isotopes in the Water Cycle, Springer Netherlands, p67-89.
-
Mayer, B., and Krouse, H.R., (2004), Procedures for sulfur isotope abundance studies, In De Groot, P.A. (ed.), Handbook of Stable Isotope Analytical Techniques, Elsevier, 1, p538-596.
[https://doi.org/10.1016/b978-044451114-0/50028-4]
-
Meijer, H.A.J., Neubert, R.E.M., and Visser, G.H., (2000), Cross contamination in dual inlet isotope ratio mass spectrometers, International Journal of Mass Spectrometry, 198(1), p45-61.
[https://doi.org/10.1016/s1387-3806(99)00266-3]
-
Migaszewski, Z.M., Gałuszka, A., Michalik, A., Dołęgowska, S., Migaszewski, A., Hałas, S., and Trembaczowski, A., (2013), The use of stable sulfur, oxygen and hydrogen isotope ratios as geochemical tracers of sulfates in the Podwiśniówka acid drainage area (south-central Poland), Aquatic Geochemistry, 19(4), p261-280.
[https://doi.org/10.1007/s10498-013-9194-7]
-
Mizutani, Y., (1971), An improvement in the carbon-reduction method for the oxygen isotopic analysis of sulphates, Geochemical Journal, 5(2), p69-77.
[https://doi.org/10.2343/geochemj.5.69]
- Morrison, J., (1999), A new high temperature EA-pyrolysis-IRMS solution to δ18O analyses of inorganic samples, Application Brief, AB10, p2.
-
Palmer, M.R., Helvací, C., and Fallick, A.E., (2004), Sulphur, sulphate oxygen and strontium isotope composition of Cenozoic Turkish evaporites, Chemical Geology, 209(3), p341-356.
[https://doi.org/10.1016/j.chemgeo.2004.06.027]
- Pichlmayer, F., and Blochberger, K., (1988), Isotopic abundance analysis of carbon, nitrogen and sulfur with a combined elemental analyzer-mass spectrometer system, Fresenius' Zeitschrift fuer Analytische Chemie, 331(2), p196-201.
- Prot, D., and Monty, C., (1996), Self-diffusion in α [sbnd] Al2O3. II. Oxygen diffusion in ‘undoped’ single crystals, Philosophical Magazine A, 73(4), p899-917.
- Rafter, T.A., (1967), Oxygen isotopic composition of sulfates. Part 1. A method for the extraction of oxygen and its quantitative conversion to carbon dioxide for isotope radiation measurements, New Zealand Journal of Science, 10(2), p493-510.
-
Sakai, H., and Krouse, H.R., (1971), Elimination of memory effects in 18O/16O determinations in sulphates, Earth and Planetary Science Letters, 11(1-5), p369-373.
[https://doi.org/10.1016/0012-821x(71)90196-8]
-
Shin, W.J., Ryu, J.S., Lee, K.S., and Park, Y., (2015), Identification of anthropogenic contaminant sources in urbanized streams using multiple isotopes, Environmental Earth Sciences, 73(12), p8311-8324.
[https://doi.org/10.1007/s12665-014-3992-0]
- Shin, W.J., Ryu, J.S., Mayer, B., Lee, K.S., and Lee, S.W., (2014), Natural and anthropogenic sources and processes affecting water chemistry in two South Korean streams, Science of the Total Environment, 485, p270-280.
-
Taylor, B.E., Wheeler, M.C., and Nordstrom, D.K., (1984), Isotope composition of sulphate in acid mine drainage as measure of bacterial oxidation, Nature, 308, p538-541.
[https://doi.org/10.1038/308538a0]
- Thode, H.G., (1991), Sulphur isotopes in nature and the environment: An overview, In Krouse, H.R., and Grinenko, V.A. (eds.), Stable Isotopes: Natural and Anthropogenic Sulphur in the Environment: SCOPE 43, John Wiley and Sons, Chichester, p1-26.
-
Ueda, A., and Krouse, H.R., (1986), Direct conversion of sulphide and sulphate minerals to SO2 for isotope analyses, Geochemical Journal, 20(4), p209-212.
[https://doi.org/10.2343/geochemj.20.209]
-
Ueda, A., and Sakai, H., (1983), Simultaneous determinations of the concentration and isotope ratio of sulfate-and sulfide-sulfur and carbonate-carbon in geological samples, Geochemical Journal, 17(4), p185-196.
[https://doi.org/10.2343/geochemj.17.185]
- Vitòria, L., Otero, N., Soler, A., and Canals, À., (2004), Fertilizer characterization: Isotopic data (N, S, O, C, and Sr), Environmental Science & Technology, 38(12), p3254-3262.
- Yanagisawa, F., and Sakai, H., (1983), Precipitation of SO2 for sulphur isotope ratio measurements by the thermal decomposition of BaSO4-V2O5-SiO2 mixtures, Analytical Chemistry, 55, p985-987.