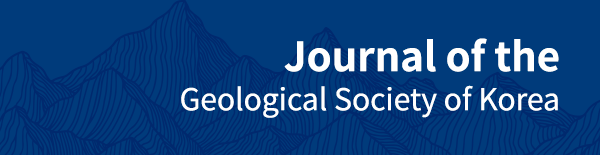
경북 포항 냉수리 제4기 단층물질과 미구조에 기록된 동지진성 미끌림 흔적
초록
활성단층의 단층물질과 미구조는 과거 단층의 미끌림 특성을 이해하는 고지진 연구와 지질재해 평가를 위한 필수적인 정보를 제공한다. 본 연구는 경북 포항시 냉수리의 굴착조사에서 관찰되는 제4기 단층대의 내부구조와 단층물질, 미구조분석을 통해 제4기 동안 발생한 단층의 미끌림 특성을 이해하는 것을 목표로 수행되었다. 동서방향의 굴착단면은 불국사 화강암류와 경상분지 하양층군을 경계하는 북북동 방향의 양산단층선을 가로지르며, >6 m 폭의 엽리성단층비지 및 각력암으로 이루어진 성숙한 단층핵대가 제4기 미고결 사질 및 자갈층을 N15°E/42°SE 자세로 날카로운 경계를 가지며 충상하는 기하를 보여준다. 제4기 단층대는 주로 결정도가 낮은 스멕타이트와 팔리고스카이트로 이루어진 점토질 단층비지들과 변형된 미고결 퇴적물로 정의되며, 미구조 분석결과, 날카로운 경계와 강한 엽리를 가지는 <1 cm 폭의 주미끌림대(PSZ)와 미세규모에서 관찰되는 <400 μm 폭의 미세-PSZ가 관찰되었다. 또한 변형된 제4기 사질층의 미구조는 입자들 간의 맞물림과 부분적인 파쇄조직을 보여준다. 이러한 미구조와 광물학적 특성들은 양산단층을 따라 지표파열을 발생시킨 지진성 제4기 단층운동이 발생하여 지표 근처의 기존 단층핵과 미고결 퇴적층 내에 빠른 미끌림이 발생하였음을 알려준다. 한편 제4기 단층물질과 미구조연구는 단층의 재활 시 나타날 수 있는 미끌림 특성을 예측하고, 보다 정확한 단층의 운동시기를 알아내는데도 기여할 것으로 기대된다.
Abstract
The materials and microstructures of active faults provide essential information to better understand the slip properties of past faults through geological hazard assessments and paleoseismology studies. This study aimed to elucidate the slip properties of the Quaternary fault during Quaternary slip. This was done through analyses of the internal structure, fault materials, and microstructure of the Quaternary fault zone observed in the trench section of the Pohang area. An E-W trending trench section excavated perpendicular to the NNE-SSW trending Yangsan fault line, which divides Hayang Supergroup and Bulkuksa Granite, reveals that a >6 m thick mature fault core zone composed of foliated gouges and breccia thrusts poorly lithified Quaternary sand and gravel deposits. Its shape boundary strikes N15°E and dips 42°SE. The Quaternary fault zone is defined by clay-rich gouges, mainly composed of poorly crystallized smectite and palygorskite, and deformed Quaternary sediments. The microstructure of the Quaternary fault zone shows a <1 cm thick principal slip zone (PSZ) and <400 μm thick micro-PSZ with sharp boundaries and strong foliations between paleo fault rocks and deformed Quaternary sediments. In addition, the microstructure of deformed Quaternary sand exhibits interconnected and partially fractured structures between the grains. These microstructural and mineralogical characteristics of the Quaternary fault suggest that the coseismic Quaternary faulting, which caused surface rupture along the Yangsan fault, was activated, resulting in rapid slips between the pre-existing fault core zone and unconsolidated sediments near the surface. Quaternary fault material and microstructural studies expect to contribute to anticipating the slip properties of the fault after reactivation and to determine a more accurate timing of faulting.
Keywords:
fault internal structure, fault materials, microstructure, Quaternary fault, Yangsan fault키워드:
단층내부구조, 단층물질, 미구조, 제4기 단층, 양산단층1. 서 언
과거에 발생되었던 지진의 위치와 규모, 재발주기, 원인을 이해하기 위해 수행하는 고지진학(paleoseismology) 연구들은 중규모 이상의 지진들의 대부분이 기존에 존재하였던 활성단층대를 따라 발생함을 보고하였다(e.g., Sibson, 1990; Meyer et al., 1994; Rajendran et al., 1996; Lin, 2001; Collettini and Holdsworth, 2004). 고지진학의 연구방법으로는 크게 지진학(e.g., Rockwell et al., 2001; Michetti et al., 2005; Ota et al., 2009), 지형 및 측지학(e.g., Sibson, 1989; Arrowsmith et al., 1997; Klinger et al., 2005; Choi et al., 2018), 그리고 야외 및 실험단층대연구(e.g., Chester and Logan, 1987; Chester and Chester, 1998; Faulkner et al., 2003; Smith et al., 2013) 등으로 나눌 수 있으며, 최근에는 이들을 통합하여 지진에 대한 근본적인 질문들에 대한 답을 찾으려는 노력들이 진행되고 있다(Sibson, 2003; Collettini et al., 2005; Valoroso et al., 2014). 이들 중, 굴착조사(trench)를 포함한 야외 단층대연구는 단층대의 직접적인 관찰을 통해 지진을 발생시킨 단층대 내부구조, 변위, 재발주기, 과거의 최대지진규모 등의 지진재해예방의 측면에서 중요하고 필수적인 정보들을 얻을 수 있으며(McCalpin, 1996; Kim and Jin, 2006), 최근에는 실내전단실험과 통합수행으로 지진에 대한 단층의 안정성을 정량적으로 평가하기 위한 연구들이 활발히 진행되고 있다(e.g., Di Toro et al., 2006; Brantut et al., 2008; Yao et al., 2013)
고지진학적 관점에서 활성단층의 과거 미끌림특성을 아는 것은 매우 중요하다. 지진 발생시 방출되는 에너지의 크기는 단층의 최종 변위나 연장보다 미끌림이 개시된 이후의 미끌림속도(slip rate)의 영향을 크게 받는 것으로 알려져 있다(e.g., Cowan, 1999). 만약 포행(creep)형 단층대와 같이 비지진성(aseismic) 미끌림특성을 가지는 단층이라면 최대지진규모 산정 시 오류를 범할 수 있으며, 주변 환경의 심각한 변화가 없는 한 재활하더라도 안정된 미끌림거동을 가질 가능성이 높다. 이러한 단층의 미끌림특성은 단층의 내부구조(internal structure)와 단층핵대(fault core zone)를 이루는 단층물질(fault material)의 종류 및 미구조에 큰 영향을 받는 것으로 알려져 있다(Bos and Spiers, 2001; Ben-Zion and Samis, 2003; Faulkner et al., 2003; Collettini et al., 2009). 특히, 단층의 미끌림이 진행될수록 미끌림의 속도가 증가하는 동적약화현상(dynamic weakening)의 원인으로 단층물질의 물리화학적(e.g., Han et al., 2007a, 2007b, 2010; Hirose and Bystricky, 2007; Di Toro et al., 2011) 및 결정구조(e.g., Brantut et al., 2016; Hayward et al., 2016)의 변화, 좁은 폭으로 발달된 주미끌림대(principal slip zone; 이하 PSZ)의 존재(e.g., Ikari, 2015) 등이 보고되었다. 따라서, 활성단층대의 미끌림특성을 이해하기 위해서는 굴착과 시추조사를 통해 실제 지진을 발생시킨 활성단층대를 구성하는 단층물질의 특성과 정밀한 미구조 분석이 필수적이다(e.g., Lin, 2001; Holdsworth, 2004; Tanikawa and Shimamoto, 2009; Janssen et al., 2010; Carpenter et al., 2012).
한반도는 다른 동아시아 국가들에 비해 지진에 비교적 안전하다고 알려져 왔지만, 최근 경주와 포항에서 발생된 두 차례 이상의 중규모 이상의 지진으로 인해 국내에서도 활성단층지도제작과 같은 장기적인 연구과제가 시작되었다. 이 과정에서 제4기 퇴적층을 절단하는 다수의 제4기 단층대들이 새롭게 발견되는 큰 성과를 거두고 있다. 그러나 단층대를 직접 관찰할 수 있는 굴착단면들은 야외 기재 및 연대측정에 필요한 시료채취 이후 지주의 요청 또는 행정적인 문제로 인해 대부분 복구되어 제4기 단층의 미끌림특성을 평가할 수 있는 단층물질들을 더 이상 체계적으로 채집할 수 없는 상황이다. 본 연구는 경북 포항시 냉수리 지역에서 양산단층대를 따라 수행된 굴착조사를 통해 새롭게 발견된 제4기 단층의 내부구조를 상세히 기재하고 단층물질과 미구조 분석을 실시하여 과거 지진성 단층운동의 흔적과 미끌림 특성을 이해하는 것을 목표로 진행되었다.
2. 연구 방법
경북 경주 북부 및 포항 남부지역의 위성영상분석결과로 인지된 양산단층의 선구조를 따라 상세한 야외조사가 실시되었으며, 제4기 퇴적층 분포 및 지질구조 분석을 통해 제4기 단층의 유력한 후보지를 선정하여 폭 1.5 m, 길이 20 m, 깊이 5 m의 동서방향의 굴착조사를 실시하였다. 굴착단면에 드러난 단층대의 내부구조를 파악하기 위해 자세한 사진촬영 및 스케치를 통해 야외특성에 따라 단층암을 분대하였다. 분대된 단층암들의 덩어리시료를 정향채취, 실내에서 평판(slab)형태의 관찰면을 연마 후 스캔작업을 실시하여 고해상도 단층암 이미지를 획득하였다. 평판에서 확인되는 미끌림대(slip zone) 및 주변부를 중심으로 박편을 제작하였으며, 제4기 단층암의 경우 동서방향의 수직단면, 일부 단층암들은 수평면을 관찰면으로 설정하였다. 모든 시료는 점토 및 미고결 퇴적물로 이루어진 시료의 특성상 수분에 취약함으로 연마 간 금속가공용 냉각유와 연마제를 섞은 현탁액을 이용하여 관찰면을 연마하였다.
단층암의 미구조와 화학조성분석을 위해 광학현미경 및 경상대학교 공동실험실습관에 위치한 에너지분산형 분광분석기(energy dispersive X-ray spectroscopy; EDS; Oxford X-Max 50)가 장착된 전계방사 전자현미분석기(field emission electron probe micro-analyzer; FE-EPMA; JEOL JXA-8530F PLUS)를 활용하였으며, 가속전압 20 kv, 빔 전류 5-10 nA의 조건으로 분석되었다. 또한, 한국기초과학지원연구원 본원에 위치한 집속이온빔(focused ion beam; FIB; FEI Quanta 3D FEG)을 이용한 박막시료제작 후 한국과학기술연구원의 투과전자현미경(transmission electron microscope; TEM; Tecnai F20 G2)을 이용하여 미끌림대를 구성하는 단층물질의 초미세구조와 결정구조를 관찰하였다.
단층암의 광물학적 조성을 이해하기 위해 분말 X-선회절(X-ray diffraction; XRD) 정성 및 정량분석을 실시하였다. 야외에서 채취된 단층물질들을 40℃의 건조오븐에서 약 24시간 동안 건조 시켰으며, 건조된 시료를 10분 동안 마이크로나이징 밀(micronizing mill)을 사용하여 <5 μm 크기의 입자로 분쇄시켰다. 분석조건은 40 kv/40 mA, 2θ 4-70° 구간에서 주사간격 0.02°, 주사시간은 0.5초로 설정하였다. 광물의 정량분석은 시로퀀트(siroquant) ver 3.0 프로그램을 활용하였으며, 2θ 9-70° 구간에서 결정학적 특성 및 리트벨트 보정을 반복적으로 실시하여 각 광물들의 카이-자승값(chi-squared value)이 3을 넘지 않는 상태에서 계산되었다.
3. 냉수리 굴착단면
한반도 동남부의 주요 단층으로 북북동방향의 양산단층계와 북북서 방향의 울산단층이 있다(e.g., Lee and Jin, 1991; Chwae et al., 1998). 1990년대 이후 한반도 동남부에 집중된 원자력 시설물의 안정성 평가를 위해 양산단층계를 대상으로 제4기 단층에 대한 연구가 활발히 진행되었으며, 그 결과로 양산단층과 울산단층 주변부에서 약 60여개의 활성단층의 위치가 보고되었다(e.g., Kim, Y.-S. et al., 2011; Kim, M.-C. et al., 2016 and references therein; 그림 1a, 1b). 최근 2017년부터 시작된 국가활성단층지도제작 과제가 진행됨에 따라 양산단층의 남부지역을 포함하여 포항-영덕 구간인 중부 및 북부에서도 새롭게 그 위치가 추가되고 있다. 이번 연구의 굴착조사 또한 국가활성단층지도제작의 일환으로 수행되었으며, 조사지점은 양산단층의 중부에 해당하는 경북 포항시 북구 신광면 냉수리에 위치한다. 단층대는 백악기 경상분지 하양층군 대구층과 불국사 화강암류에 속하는 흑운모 화강암의 경계를 이루며(그림 1c, 1d) 굴착단면에서 제4기 퇴적층을 절단한다(그림 2). 연구지점에서 약 2.5 km 남부에는 제4기 층을 절단하는 벽계 및 단구단층(Kee et al., 2009; Lee et al., 2015)이 인접한다(그림 1c).
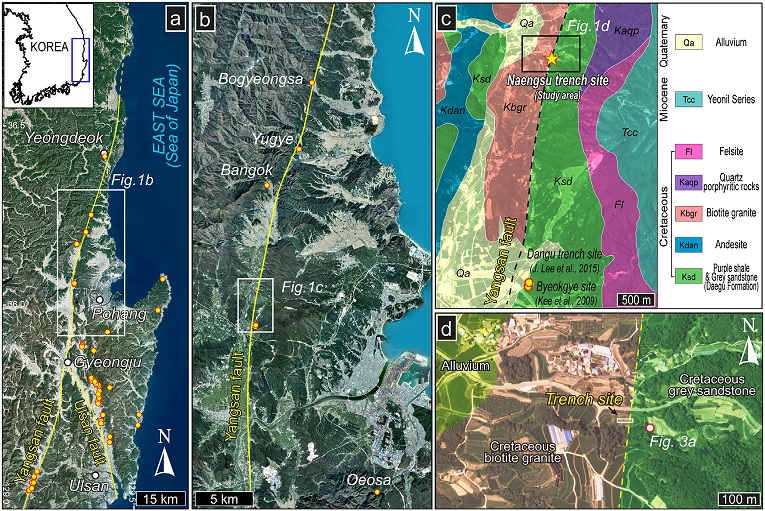
Satellite images show the locations of the Quaternary faults discovered within the Yangsan fault system (a) and the Pohang area (b) (modified from Kim, Y.-S. et al., 2011; Kim, M.-C. et al., 2016 and references therein). (c) Geological map of the study area (yellow star: trench site). (d) Aerial photograph shows the location of the trench section excavated on the Yangsan fault line (yellow dashed line).
4. 단층대 내부구조
굴착단면에서 관찰되는 단층대는 동측의 엽리상 단층비지 및 단층각력암대로 이루어진 단층핵(fault core) 전체가 서측의 제4기 사질 및 역질 퇴적층을 N15°E/42°SE 자세로 충상하는 기하를 가지며, 날카로운 경계면이 인지된다(그림 2). 여러 종류의 단층암들로 구성된 동측의 넓은 단층핵대는 입자크기감소(grain size reduction)와 강한 점토질 엽리가 두껍게 발달되어 있어 제4기 시기 이전 지하에서부터 발달되어 온 성숙한(mature) 단층대의 특성을 보여준다. 따라서 제4기 단층대의 특성을 이해하기 위해서는 기존 단층대와 제4기 단층대의 변형구조들을 명확히 구분하여 분석해야 한다.
4.1 기존 단층대
단층암대는 크게 두 그룹의 엽리상단층비지대(foliated gouge zone)와 그 사이에 위치하는 갈색각력암(brown breccia) 등으로 구성된다(그림 2). 굴착단면에서 확인되는 단층핵의 폭은 약 6 m 이며, 동쪽 끝 지점을 기준으로 동측으로 약 50 m 떨어진 지점(그림 1d의 흰색 점)에서 채집된 백악기 퇴적암은 단열의 밀도가 높고, 망상의 세맥들이 단열들을 채우고 있는 전형적인 단층손상대(fault damage zone)의 조직을 보여준다(그림 3a). 이러한 야외특성들은 연구지역 단층대의 폭이 약 50 m 이상일 가능성을 지시하며, 연구지역 일대의 지질구조와 위성이미지, 그리고 양산단층 내부구조에 대한 기존 연구결과들(Choi et al., 2009; Kim, C.M. et al., 2016; Cheon et al., 2019)을 고려하였을 때 본 단층대는 양산단층의 주단층대(main fault zone)일 가능성이 존재한다.
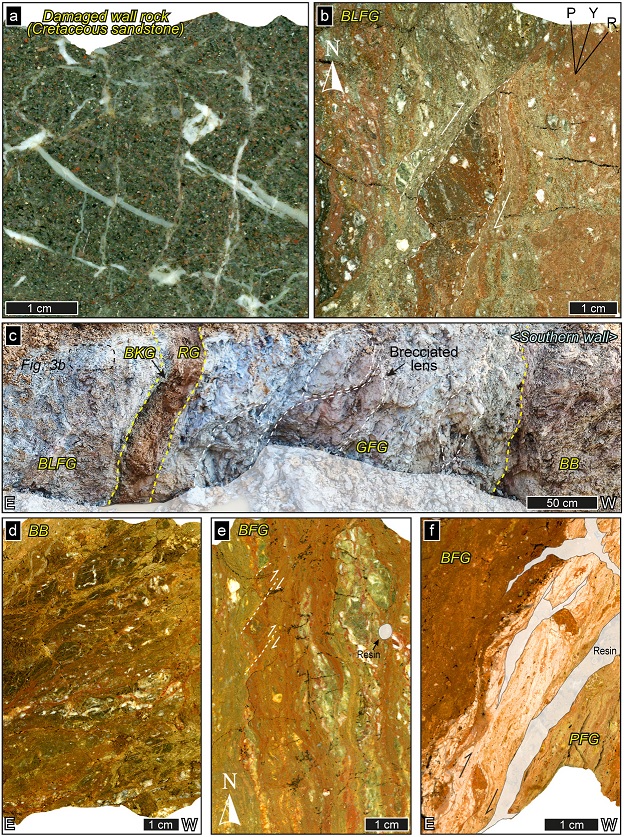
High-resolution scanned slab images of damaged wall rock (a), and blue-gray foliated gouge (BLFG; b). (c) Outcrop photograph of gray foliated gouge (GFG). High-resolution scanned slab images of brown breccia (BB; d), brown foliated gouge (BFG; e), and the boundary between BFG and pink foliated gouge (PFG; f). BKG: black gouge, RG: red gouge.
굴착단면의 가장 동측에 위치한 청회색엽리상단층비지(blue-gray foliated gouge: BLFG; 그림 3b)는 가장자리에 위치하여 그 폭을 정확히 알 수 없지만 최소 1 m 이상으로 추정되며, 비대칭(asymmetric)구조의 청회색, 담갈색과 암회색의 점토질 엽리대, 그리고 수 mm 이하로 미세하게 파쇄된 암편들이 유동하며 생성된 파쇄엽리(cataclastic foliation)들로 구성된다. 수평방향의 관찰면에서 관찰되는 비대칭 암편들의 기하들은 우수향의 운동감각을 지시하며, 그에 따른 R- 또는 P-전단방향으로의 엽리들이 연속적으로 발달한다. 회색엽리상단층비지(gray foliated gouge: GFG; 그림 3c)는 약 1.6 m 폭으로 관찰되며, 암편의 함량이 매우 낮은 회색 내지 청회색 점토질 엽리성단층비지대와 최대 50 cm 크기의 각력화(brecciated)된 렌즈상의 암편들로 구성된 성숙한 단층암의 특성을 잘 나타낸다. BLFG와 GFG 의 경계부에는 흑색(black gouge: BKG) 및 적색(red gouge: RG) 단층비지들이 각각 약 20 cm 와 10 cm 폭으로 발달한다. 갈색단층각력암(brown fault breccia: BB; 그림 3d)의 폭은 약 1.2-2.0 m 이며, 내부에 약 10 cm 폭의 회색단층비지대(gray gouge zone: GG; 그림 2)를 포함한다. 동서 방향의 단면으로 제작된 평판은 수 mm 폭의 점토와 석영으로 이루어진 세맥(vein)들이 주입되고 열수변질 된 모암이 각력화 된 조직을 보여주며, 수 많은 미세단열들은 대부분 점토광물로 추정되는 미세크기의 물질들로 채워진다.
제4기 퇴적층과 인접한 약 60 cm 폭의 갈색엽리상단층비지(brown foliated gouge: BFG; 그림 3e)는 갈색 및 올리브색 점토광물들이 교호하는 점토질 엽리들과 최대 수 cm 크기의 렌즈상 암편들로 구성되어 있으며, 암편의 일부는 파쇄된 작은 암편조각들이 이동하여 주변 엽리와 조화적으로 배치되는 파쇄유동구조(cataclastic flow structure)를 보여준다. 수평방향으로 절단된 평판에는 우수향 전단감각지시자들이 관찰되며, 렌즈상의 암편들은 소규모 R-전단단열에 의해 절단된 모습을 관찰할 수 있다. 제4기 퇴적층과 직접적으로 접하는 담홍색엽리상단층비지(pink foliated gouge: PFG; 그림 3f)는 제4기 퇴적층과 경계부를 따라 약 10-20 cm 폭으로 발달하며, 이번 굴착단면에서 관찰되는 단층암들 중 암편의 함량이 가장 낮고 점토광물로 이루어진 기질부가 대부분을 이룬다. 기질부는 담홍색 및 담갈색의 점토들이 매우 얇은 폭의 엽리들을 정의하며, 갈색의 암편들은 대부분 심각하게 파쇄되었다. 동서방향의 수직단면으로 제작된 PFG와 BFG의 경계부 평판은 PFG 내부의 암편들의 우수향의 전단감각을 지시하므로 그 경계부를 따라 과거에 역단층성 미끌림이 작동되었음을 알 수 있다.
4.2 제4기 단층대
제4기 퇴적층은 하부의 담황색사질층(Quaternary sand; QS)과 상부의 갈색자갈층(Quaternary gravel; QG)으로 구성되며, 제4기 퇴적층과 기존 단층암이 접촉하는 경계면은 서로 다른 색과 조직을 가지는 단층암 내지 퇴적물들로 인해 비교적 명확히 경계가 인지된다(그림 4c, 4d). PFG와 제4기 퇴적물 사이에는 엽리가 거의 발달하지 않는 갈색단층비지(brown gouge: BG)가 날카로운 경계면을 따라 ~3 cm 폭으로 발달한다. BG와 퇴적층의 경계부 상부에는 자갈층 보다 아래에 위치한 사질 물질들이 쐐기형태로 위치하며, 하부로 갈수록 두께가 얇아지며 첨멸한다(그림 2, 4a). 그 하부에는 PFG와 얇은 폭의 BG, QG가 직접 접촉하는 부분이 관찰되는데, 마찬가지로 PFG와 BG의 경계는 매우 날카롭다(그림 4b).
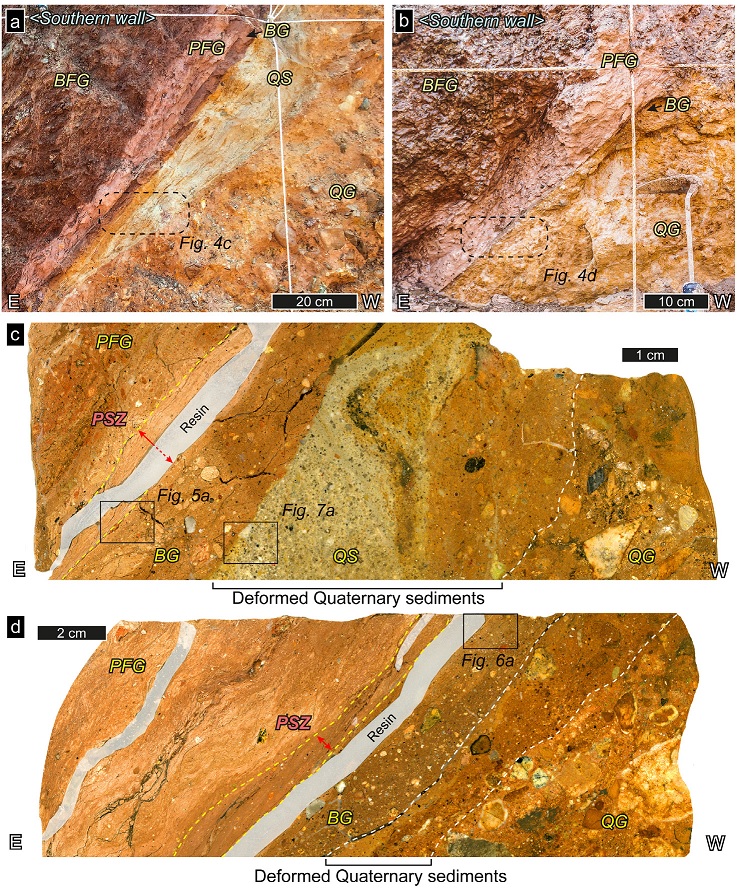
Outcrop photographs and slap images of the Quaternary fault zone. (a) The Upper part of the Quaternary fault zone. (b) The lower part of the Quaternary fault zone. Scanned images of slabs taken from black dashed boxes in Fig. 4a (c) and 4b (d) exhibit the occurrence of the paleo fault rocks and deformed Quaternary sediments in detail. Note the <1 cm thick principal slip zone (PSZ) developed along the boundary between PFG and BG. BFG: brown foliated gouge, PFG: pink foliated gouge, BG: brown gouge, QS: Quaternary sand, QG: Quaternary gravel, PSZ: principal slip zone.
이러한 특성들은 동서방향의 수직단면으로 제작된 평판에서도 잘 관찰된다. 쐐기형태의 사질물질이 나타나는 상부 경계의 평판은(그림 4c) PFG와 제4기 퇴적물, 그리고 둘 사이에 위치한 BG와의 접촉관계를 잘 보여준다. 특히, PFG와 BG 사이에는 암편들의 극적인 입자크기감소현상과 미끌림방향과 거의 나란한 엽리들이 강하게 발달하는 1 cm 미만의 폭의 주미끌림대(PSZ)를 관찰할 수 있었다. 또한, BG와 QG 사이에 존재하는 쐐기형태의 사질층 및 그와 인접한 자갈층 내부에는 주변부에 비해 입자크기가 감소된 영역과 일부 입자유동(granular flow) 및 파쇄유동구조를 보이는데, 이들은 제4기 이후 단층활동에 의한 변형의 결과로 판단된다. 하부 경계에서 채집된 평판은(그림 4d) 암편이 거의 관찰되지 않고 점토광물들이 이루는 파상(wavy)의 엽리들이 발달하는 PFG의 특성을 잘 보여주며, BG와 경계부에는 PSZ, 그리고 BG와 자갈층 사이에 약 2-3 cm 폭의 변형대가 일관성 있게 관찰된다. BG 내부의 암편 및 기질부는 성숙한 단층대로부터 유래된 물질들과 제4기 퇴적층의 물질들이 모두 관찰되므로 제4기 단층활동과 함께 생성된 단층암으로 추정된다. 이상의 관찰결과를 종합하였을 때, 제4기 시기의 운동으로 변형된 영역은 PFG 내부에서 발달하는 PSZ와 BG, 그리고 변형된 제4기 퇴적층 영역(그림 2의 하늘색 점선 영역)이 기존 성숙된 단층대를 따라 제4기 단층운동으로 새로이 만들어진 단층대로 정의된다.
5. 제4기 단층대의 미구조
제4기 단층대의 평판에서 인지된 PSZ와 BG, 그리고 변형된 제4기 퇴적물의 미구조적 특성을 파악하기 위해 그들의 주변부를 포함한 박편을 제작하여 미구조 관찰을 실시하였다. BG는 갈색의 미립의 불투명 및 점토광물들로 구성된 기질부와 최대 2 mm 크기의 석영, 장석 및 모암의 암편들로 이루어져 있으며, 엽리가 미약하게 발달되어 있다. 반면, PSZ는 BG에서 유래된 단층암 파편 및 수 십 μm 크기의 미세암편들을 포함하지만 함량이 매우 낮고 대부분 세립의 점토광물들로 이루어져 있으며, 광학현미경의 보조검판(accessory plate) 삽입 시 구성광물들의 강한 선호방위(preferred orientation)을 관찰할 수 있다(그림 5a, 5b). 전자현미경에서도 암편의 함량이 낮고 엽리가 잘 발달된 PSZ와 상대적으로 암편의 함량이 높고 미약한 엽리의 BG의 미구조적 특성이 잘 관찰되며(그림 5c), 그들 간의 날카로운 경계부에는 수 μm 크기의 암편들과 초미립(ultra-fine)의 기질부로 이루어진 미끌림대를 관찰 할 수 있다(그림 5d). 투과전자현미경(transmission electron microscope; TEM)을 사용하여 초미립 단층물질의 미세구조와 결정구조를 분석한 결과, 소량의 0.1-1.0 μm 크기의 석영 암편들과 침상의 팔리고스카이트(palygorskite) 및 판상의 스멕타이트로 이루어진 기질부로 구성되며, 기질부의 선택영역전자회절(selected area electron diffraction; SAED)패턴은 산란되는 영역과 동심원상의 고리(ring)형태의 패턴이 함께 인지되는데, 이는 결정축의 선호방위가 없고 결정도가 낮은 단층물질의 패턴을 지시한다(Viti, 2011; 그림 5e, 5f).
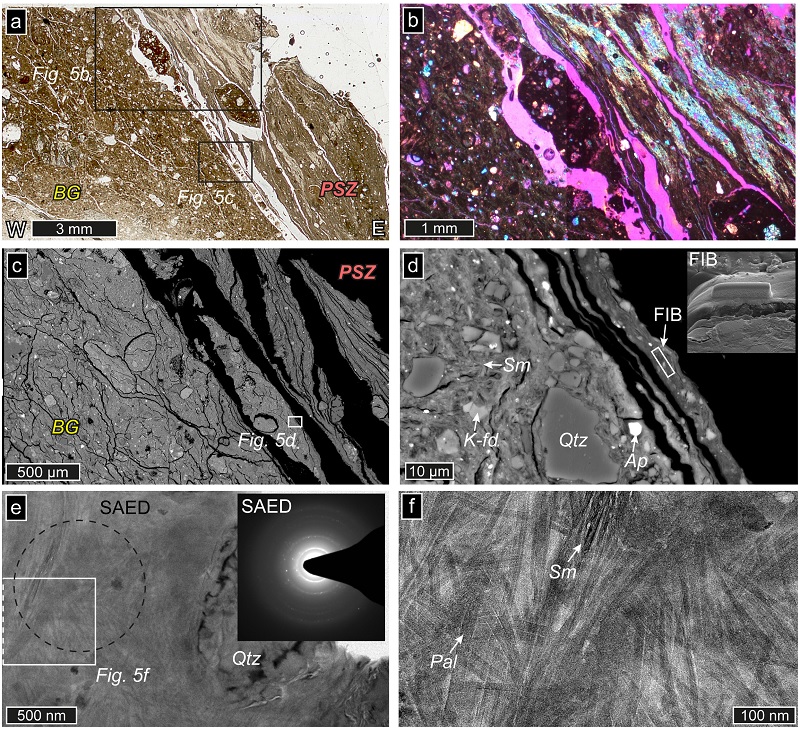
(a) Scanned thin-section image showing the shape boundary between BG and PSZ (plane polarized light). (b) Photomicrograph showing a strong preferred orientation of PSZ materials (crossed polarized light; gypsum plate insert). (c) SEM-BSE image of the boundary between BG (light and weak foliated materials) and PSZ (dark and strong foliated materials). (d) High magnification SEM-BSE image showing the ultra-fine materials along the boundary of BG and location of FIB sampling (white box and inset). (e) Bright-field TEM image showing the various size of quartz clasts and the networks of platy and acicular clay minerals. Selected area electron diffraction (SAED) pattern of the inset image represents randomly oriented, poorly crystalline grains (e.g., Viti, 2011). (f) High magnification bright-field TEM image of palygorskite and smectite in PSZ. Sm: smectite, K-fd: K-feldspar, Qtz: quartz, Ap: apatite, Pal: palygorskite.
PSZ와의 경계면에 인접한 BG 내부 영역에는 매우 강한 선호방위를 가지는 점토질의 미세-PSZ(micro-PSZ)가 최대 400 μm 폭으로 관찰된다(그림 6a, 6b). 전자현미경에서 관찰되는 미세-PSZ의 특징은 미끌림 방향으로 평행 또는 아평행한 방향의 엽리들이 잘 발달하며, 최대 수 십 μm 크기의 암편 및 BG 물질로 이루어진 암편들과 초미립의 기질부로 구성된다(그림 6c, 6d). 기질부의 투과현미경분석결과, 대부분의 영역은 침상의 팔리고스카이트로 구성되었으며, 본 영역에서도 산란 및 고리형태의 패턴이 함께 인지되는 SAED 패턴을 가진다(그림 6e, 6f). 또한 EDS 결과는 팔리고스카이트의 전형적인 화학조성((Mg,Al) 2Si4O10(OH)·4(H2O)) 이외에 소량의 Fe가 검출되는데(그림 6g), 이는 Mg의 일부가 Fe로 치환된 결과로 추측된다. 일부 영역에서는 침상의 팔리고스카이트가 유사한 방향으로 잘 배열되어 있다(그림 6h).
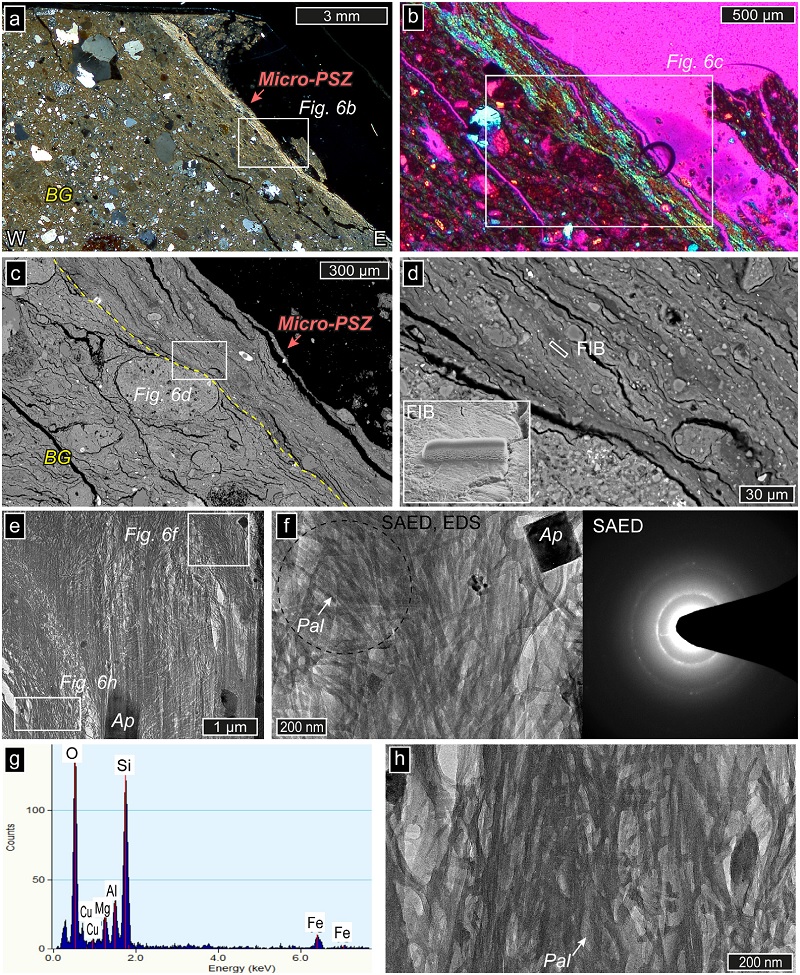
(a) Scanned thin-section image showing micro-PSZ in BG (crossed polarized light). (b) Photomicrograph showing a narrow (<400 μm) micro-PSZ with strong foliations (crossed polarized light; gypsum plate insert). (c) SEM-BSE image of the boundary between BG and micro-PSZ. (d) High magnification SEM-BSE image showing the ultra-fine matrix materials in micro-PSZ and the location of FIB sampling (white box and inset). (e) Low magnification bright-field TEM image of the matrix of micro-PSZ mainly composed of acicular palygorskite. (f) Selected area electron diffraction (SAED) pattern of the inset image represents randomly oriented and poorly crystallized palygorskite. (g) EDS pattern of the dashed black circle in Fig. 6f. (h) High magnification bright-field TEM image showing the nano-foliation of palygorskite. Pal: palygorskite, Ap: apatite.
BG와 인접한 제4기 사질층(그림 4c)의 미구조 분석결과, 기질부는 점토광물로 구성되며, 암편들은 약 1-2 mm 크기로 분급이 양호하며 BG와의 경계부로 가까워질수록 입자들의 크기가 다소 감소하는 경향을 보인다(그림 7a). 암편들은 주로 석영 및 장석류(사장석 및 K-장석)로 이루어 졌으며, 미세단열(microfracture)들이 발달하는 수 백 μm 이상의 비교적 큰 크기의 암편들은 맞물린 채로 서로 접촉한다(그림 7b). 또한, 전자현미경 상에서는 석영의 가장자리가 패각상(conchoidal)으로 깨져 만들어진 단열(spalling fracture)와 열림(open)형의 입자를 가로지르며 생성되는 단열(transgranular fracture)들이 관찰되며, 일부 석영 입자에서는 과거의 미세단열들이 생성 된 후 모입자와 같은 성분으로 다시 매워진 회복단열(healed fracture)들이 관찰된다(그림 7c, 7d).
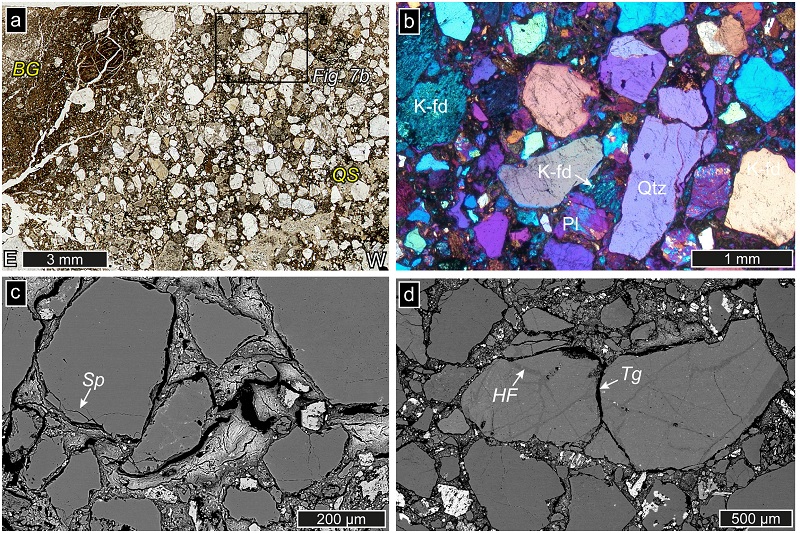
(a) Scanned thin-section image of Quaternary sand (QS) in the deformed Quaternary sediments (plane polarized light). (b) Photomicrograph showing the microfractured and interconnected quartz and feldspar grains (crossed polarized light; gypsum plate insert). (c) SEM-BSE image showing the fresh spalling fractures which developed at the margin of quartz grains. (d) SEM-BSE image showing healed and transgranular fractures in quartz grains. Qtz: quartz, Pl: plagioclase, K-fd: K-feldspar, Sp: spalling fracture, Tg: transgranular fracture, HF: healed fracture.
6. 분말 XRD 분석
제4기 단층대를 이루는 사질 및 역질 퇴적물 및 갈색단층비지(BG)와 기존 단층대의 갈색(BFG)및 담홍색엽리상단층비지(PFG), 그리고 평판에서 인지된 PSZ를 이루는 단층물질들의 광물학적 특성을 이해하기 위해 정성 및 정량 분말 XRD분석을 실시하였다(표 1; 그림 8). 제4기 역층의 기질부(QM)와 사질층(QS)에서 채취된 시료들의 석영 및 장석류 함량은 각각 73.4% 및 78.0%로 매우 높은 비율을 차지하며, 주로 스멕타이트로 구성되며 소량의 일라이트/백운모 및 고령석(kaolinite)를 포함하는 점토광물들의 총량은 각각 26.6%, 22.0%의 함량비를 나타낸다. BG는 석영 및 장석류가 34.6%를 차지하며, 로몬타이트(laumontite) 및 클리놉틸로라이트(clinoptilolite) 등의 불석류(zeolite)가 7.0%, 스멕타이트류가 58.4%를 차지한다. PSZ와 PFG 시료들은 서로 유사한 광물구성을 가진다. 석영 및 장석류가 각각 28.2% 및 24.1%, 점토광물들이 각각 71.7% 및 76.0%를 차지하는데, 이 중 PSZ는 42.3% 및 25.0%, PFG는 61.1%및 14.9%의 스멕타이트 및 팔리고스카이트 함량을 보인다. 평판에서 비교적 암편의 함량이 높았던 BFG의 경우, 석영 및 장석류가 40.0%의 비율을 차지하며, 불석류 광물인 로몬타이트(17.6%) 및 스멕타이트(37.4%), 그리고 소량의 고령석(5.1%)으로 구성된 점토광물들 역시 분석되었다. 또한, 비정질(amorphous)물질의 XRD 패턴에서 흔히 관찰되는 2θ 20°-40° 사이의 높은 배경값(background; e.g., Lin, 1994)은 명확히 확인할 수 없었다.
7. 토 의
7.1 제4기 단층대의 미구조에 기록된 동지진성 미끌림의 흔적들
냉수리의 제4기 단층대는 기존에 발달되었던 점토 및 각력질의 성숙한 단층핵대 전체가 제4기 이후 지표에서 퇴적된 미고결 퇴적층을 직접 충상하고 있어, 제4기 단층활동이 개시되었던 시기에는 단층대를 둘러싼 물질들의 강도(strength)와 봉압(confining pressure)이 상대적으로 매우 낮은 상황이었음을 짐작할 수 있다. 이러한 역학적 환경에서 미끌림을 경험한다면 미끌림이 집중되지 못하고 분산되어 점토질 단층암에서는 두꺼운 엽리대의 발달, 미고결 퇴적물에서는 입자의 구름(rolling)에 의한 변형대가 쉽게 발달하는 것으로 알려져 있다(Cowan, 1999; Baldwin et al., 2002; Kee et al., 2007). 그러나 연구지역의 제4기 단층대는 이러한 불리한 조건들에도 불구하고 미끌림이 극도로 좁은 폭에 집중되어 극적인 입자크기감소와 구성광물들의 강한 선호방위를 가지는 <1 cm 폭의 PSZ 및 <400 μm 폭의 미세-PSZ, 그리고 변형된 사질층 입자들의 파쇄작용(cataclasis)이 관찰되는 것이 특징이다.
최근 활성단층대의 단층핵을 대상으로 수행된 미구조연구들과 고속마찰실험 결과들은 단층의 동지진성 미끌림과 좁은 PSZ의 생성이 서로 연관되어 있음을 보여준다. 지진성 단층운동 또는 지표파열(surface rupture)을 경험한 것으로 판단되는 자연의 활성단층대를 대상으로 수행된 다수의 미구조연구들은 단층핵 내부에서 초파쇄암(ultracataclasite; e.g., De Paola et al., 2008; Smith et al., 2011), 나노입자(e.g., Siman-Tov et al., 2013), 비정질물질(e.g., Collettini et al., 2013; Tarilng et al., 2018), 판상규산염광물(e.g., Balsamo et al., 2014; Smeraglia et al., 2017)등의 다양한 단층물질들로 이루어진 1 mm 이하의 미세-PSZ들과 이와 동반되는 온도상승의 흔적들을 보고하였다. 이러한 미끌림대의 온도상승은 좁은 미끌림대를 따라 빠른 미끌림이 국지화(localization) 되는 경우 쉽게 발생한다(e.g., Sibson, 2003; Rice, 2006). 또한, Smith et al. (2017a)는 방해석(calcite) 및 백운석(dolomite)으로 이루어진 단층비지를 빠른(<1.13 m/s) 미끌림속도로 0.03-1 m 범위의 변위를 단계적으로 적용시킨 전단실험결과, 동적약화현상이 시작되는 시점에서 회수된 시료의 미구조는 날카로운 주미끌림면(principal slip surface)과 함께 약 40 μm 폭의 강한 엽리를 가지는 미세-PSZ가 발달됨을 보여줬으며, 이를 동지진성엽리(coseismic foliation)로 해석하였다. 이번 연구의 미구조 관찰에서 PSZ와 동반된 온도상승의 확실한 증거는 찾지 못하였지만, 제4기 단층의 미끌림이 분산되지 않고 국지화되었음을 보여주는 매우 좁은 폭의 직선형 PSZ와 미세-PSZ의 존재를 확인하였다. 한편 단층암 XRD 분석결과는 주변 단층암에 비해 미끌림이 집중된 PSZ의 팔리고스카이트의 함량이 증가하는 것을 보여주는데(표 1), 이러한 광물조성의 변화가 마찰열에 의한 온도상승과 관련되는지를 밝히기 위해서는 PSZ물질의 고속마찰실험 후 회수된 시료를 대상으로 보다 정밀한 광물학적 비교연구가 필요하다.
변형된 미고결 사질층의 미구조는 서로 접촉면을 공유하는 미세단열이 발달한 입자들과 가장자리가 패각상으로 깨지거나 입자 중심을 가로지르는 열림형 단열이 발달된 석영입자들을 보여준다(그림 7). 이러한 미구조적 특성들은 활성단층대 내부의 미고결 사질층에서 발달하는 변형띠(deformation band)들을 대상으로 연구되어 왔다. Cashman et al. (2007)은 산안드레아스(San Andreas)단층의 분절들 중 비지진성의 포행형 분절지역과 지표파열을 경험한 분절지역의 미고결 퇴적층에서 관찰되는 변형띠들의 미구조를 비교한 결과, 전자의 입자들은 전단 및 구름에 의한 입자배열을 가지고 있었지만, 후자의 입자들은 미세단열들이 발달하며, 원마도가 불량하고, 입자들이 서로 접촉한 상태로 파쇄되는 교량구조(bridge structure)가 관찰되었다. 또한, 알프스와 인접한 활성단층대에서 수행된 미고결 퇴적층의 변형띠 미구조연구(Shipton et al., 2017)는 입자 가장자리의 신선한 패각상의 깨짐단열(spalling fracture)과 입자의 중심부를 가로지는 단열(transgranular fracture)들이 발달함을 보고 하였는데, 이러한 단열들은 봉압이 매우 낮은 천부에서 생성될 수 있으며(e.g., Rawling and Goodwin, 2003), 봉압이 낮은 상황에서 파쇄작용이 발생될 수 있는 조건을 지하로부터 개시된 동지진성의 빠른 미끌림의 전파에 의한 것으로 해석하였다(e.g., Balsamo and Storti, 2011). 따라서 냉수리 제4기 단층대의 미고결 퇴적층은 퇴적된 이후 지표변형을 일으킬 정도의 규모를 가지는 지진에 기인한 빠른 미끌림을 겪은 것으로 판단된다.
7.2 양산단층의 신기 운동
냉수리 굴착단면에서 관찰된 단층대는 양산단층의 주단층핵으로 추정되는 두꺼운 단층핵대가 제4기 퇴적층을 충상하는 기하를 보여주고 있어, 제4기 동안 양산단층의 주단층대가 재활되었을 가능성을 보여준다. 현재까지 보고된 양산단층계 내부의 제4기 단층들은 주로 양산단층 및 울산단층의 주변부에 위치하여 단층손상대 내부 부차단층(subsidiary fault)들의 재활로 해석되어 왔다(e.g., Ree et al., 2003). 일반적으로 양산단층의 주단층대는 백악기 경상분지의 쇄설성 퇴적층 및 화산암류, 그리고 이를 관입한 심성암체들을 절단하는 것으로 알려져 있지만, 양산단층 주단층핵대(main fault core zone)의 신기 운동에 관해서는 현재까지도 논란 중이다. 구조지질학 및 연대측정연구들은 53.9±0.3 Ma (U/Pb)의 경북 포항시 기계면 화강암체(Hwang et al., 2012), 44.7±1.1 Ma (K-Ar)의 경북 포항시 흥해읍 유문암질 응회암 등이 양산단층에 의해 절단됨을 보고하였다(Shibata et al., 1979). 또한, 최근 경북 영덕군 일원에서 수행된 연구결과들은 23.1±0.2 Ma ~ 22.97±0.13 Ma (U/Pb)의 연대를 가지는 유문암질 화산암이 양산단층에 의해 절단되었으나 단층대를 피복하는 제4기 퇴적층은 절단되지 않음을 보고하였다(Kang et al., 2018; Cheon et al., 2019). 그러나, 연구지역으로부터 약 10 km 북부에서 조사된 경북 포항시 청하면 유계리의 유계단층의 경우, 양산단층의 주단층핵대로 보이는 최소 6 m 폭의 성숙한 각력 및 점토질 단층핵대가 1314 B.P. 이후에 퇴적된 제4기 퇴적층을 직접 충상하는 사실을 보고하였으며(e.g., Kyung, 2003; Kim and Jin, 2006), 이는 연구지역 단층대의 내부구조와 매우 유사하다. 또한 연구지역에서 약 2.5 km 남부에 위치하며 경주시 단구리의 양산단층 연장부에서 굴착조사로 확인된 단층(그림 1c)은 조선시대 중기(AD 1,550-1,700)의 도자기를 포함한 제4기 퇴적층(7.4±0.4 ka, OSL)을 절단함을 보고하였다(Lee et al., 2015). 이러한 연구결과들은 경주-포항 일원의 중부 양산단층의 주단층대를 따라 아주 최근까지 지진을 동반한 단층운동이 발생하였음을 지시한다. 한편 양산단층은 하나의 단일 단층대가 아닌 여러 개의 분절로 나누어지며 (e.g., Lee and Jin, 1991; Chang and Chang, 2009; Choi et al., 2017), 분절에 따라 운동학적 특성과, 운동시기, 미끌림거동을 달리 할 가능성이 높으므로 각 분절에 따른 자세한 사례연구들이 추가되어야 전체 양산단층의 운동사를 보다 심도 깊게 논할 수 있을 것이다.
7.3 제4기 단층연구에서 단층물질과 미구조분석의 중요성
Sibson (1977)에 의해 단층암이 체계적으로 분류된 이래로 분석기술의 발달과 함께 단층의 내부구조와 단층핵을 이루는 물질들과 미구조에 관한 이해가 비약적으로 발전하면서, 최근에는 활성단층의 안정성 연구에도 활발히 적용되고 있다. 여기서 단층물질 및 미구조분석의 중요성은 다음과 같다.
첫째, 단층의 과거 미끌림특성을 추정할 수 있다. 미끌림대는 미끌림과정에서 여러가지 물리화학적 과정들을 겪게 되며, 이것은 단층암의 물질특성과 미구조 형태로 기록되므로 지진성 및 비지진성 단층대의 미구조 및 물질들의 비교분석을 통해 지진성 단층과정의 특성을 이해할 수 있다(Cowan, 1999; Cashman et al., 2007; Han et al., 2007a; Rowe and Griffith, 2015). 또한, 천부의 미고결 퇴적층 내부에 발달하는 좁은 PSZ를 따라 지진성 미끌림이 작동된 후 마찰열의 크기에 따라 단층물질의 특성이 변화하며(e.g., Balsamo et al., 2014), 과거 미끌림 당시의 최대마찰온도에 따른 미끌림속도를 정량적으로 산정하는 연구가 진행중이다(Tarling et al., 2018).
둘째, 향후 재활 시 미끌림특성에 영향을 주는 필수 정보들을 제공한다. 지진성 단층운동의 역학적 특성을 정량적으로 이해하기 위해 많은 실내전단실험연구들이 수행되어왔으며, 그로부터 알 수 있었던 사실은 미끌림대의 물질의 종류(e.g., Tembe et al., 2010; Ikari et al., 2011; Woo et al., 2016)와 입도(e.g., Han et al., 2010, 2011) 및 결정구조(e.g., Hayward et al., 2016; Rowe et al., 2019), 미끌림 이전에 발달된 PSZ의 존재(e.g., Ikari, 2015) 등이 재활 이후 미끌림특성에 큰 영향을 끼친다는 점이다. 이러한 실내전단실험들은 실험기의 구조 및 기술적 제약으로 인해 인공적인, 또는 가공된 단층물질을 사용한 실험들이 주류를 이루어 자연상태의 복잡한 단층물질의 특성을 온전히 적용하는데 한계점을 가진다. 그러나 최근 자연단층대의 단층물질을 가공없이 채취하여 자연 단층암의 미구조가 보존된 웨이퍼(wafer)를 활용한 전단시험들이 시도되고 있으며(e.g., Collettini et al., 2009; Smith et al., 2017b; Woo and Han, 2019), 그 결과, 가공된 것과 그렇지 않은 단층물질들 간의 역학적특성이 서로 다름을 보고하였다. 따라서 현재 단층의 미구조와 물질의 상태를 정확히 분석하는 것은 향후 미끌림특성을 이해하기 위한 중요한 첫 걸음이다.
셋째, 단층의 운동시기를 결정하는데 기여한다. 단층의 활동시기를 알아내기 위한 단층암 연대측정법들은 제4기 단층조사에서 중요한 부분을 차지한다. 단층암의 연대를 측정할 수 있는 대표적인 방법으로는 전자스핀공명(electron spin resonance: ESR; e.g., Fukuchi, 1988; Lee and Schwarcz, 2001), K-Ar (e.g., Zwingmann et al., 2004), 피션트랙 연대법(e.g., Tagami and Murakami, 2007) 등이 널리 사용되고 있다. 위의 방법들은 다소 차이점들을 가지지만 미끌림으로 인해 발생되는 마찰열에 의해 연대측정인자들이 초기화되는 성질을 이용하는 연대측정법이기 때문에 단층의 미끌림과정에서 미끌림이 집중되어 가장 높은 마찰열을 경험한 PSZ 영역과 미구조적 선후관계들을 고려한 시료수집과정이 필수적이다. 또한, 최근 수행된 고속마찰실험결과들은 약 300 μm 폭의 미세-PSZ을 따라 발생된 마찰열에 의해 석영의 OSL 신호가 초기화 됨을 보고하여(Kim, J.H. et al., 2019; Yang et al., 2019) 미세-PSZ를 고려한 시료채취도 중요하다. 게다가 7870±14 B.P. (14C) 이후에 퇴적된 단구층을 절단하는 활성단층의 PSZ의 미구조연구(Hirono et al., 2016)는 초미세크기의 비정질물질의 양이 활성단층의 운동시기를 결정하는 중요한 역할을 하는 것으로 보고되었다.
Acknowledgments
이 연구는 기상청지진기술개발사업(KMI2018-01710)의 지원으로 수행되었다. 이번 연구에 사용된 모든 자료의 검토와 연구방향을 지도해주신 한래희 지도교수님과 굴착조사에 도움을 준 경상대학교 및 부산대학교 구조지질학 연구실 동료들에게 깊은 감사의 마음을 전한다. 또한 점토광물정량법에 도움을 주신 안동대학교 정기영 교수님과 심사 간 유익한 조언을 주신 두 분의 익명의 심사위원들께도 감사드린다.
References
-
Arrowsmith, R., McNally, K. and Davis, J., 1997, Potential for Earthquake Rupture and M 7 Earthquakes Along the Parkfield, Cholame, and Carrizo Segments of the San Andreas Fault. Seismological Research Letters, 68, 902-916.
[https://doi.org/10.1785/gssrl.68.6.902]
-
Baldwin, J.N., Barron, A.D., Kelson, K.I., Harris, J.B. and Cashman, S., 2002, Preliminary Paleoseismic and Geophysical Investigation of the North Farrenburg Lineament: Primary Tectonic Deformation Associated with the New Madrid North Fault?. Seismological Research Letters, 73, 393-413.
[https://doi.org/10.1785/gssrl.73.3.393]
-
Balsamo, F., Aldega, L., De Paola, N., Faoro, I. and Storti, F., 2014, The signature and mechanics of earthquake ruptures along shallow creeping faults in poorly lithified sediments. Geology, 42, 435-438.
[https://doi.org/10.1130/G35272.1]
-
Balsamo, F. and Storti, F., 2011, Size-dependent comminution, tectonic mixing, and sealing behavior of a “structurally oversimplified” fault zone in poorly lithified sands: Evidence for a coseismic rupture?. Geological Society of America Bulletin, 123, 601-619.
[https://doi.org/10.1130/B30099.1]
-
Ben-Zion, Y. and Sammis, C.G., 2003, Characterization of Fault Zones. Pure and Applied Geophysics, 160, 677-715.
[https://doi.org/10.1007/PL00012554]
-
Bos, B. and Spiers, C.J., 2001, Experimental investigation into the microstructural and mechanical evolution of phyllosilicate-bearing fault rock under conditions favouring pressure solution. Journal of Structural Geology, 23, 1187-1202.
[https://doi.org/10.1016/S0191-8141(00)00184-X]
-
Brantut, N., Passelègue, F.X., Deldicque, D., Rouzaud, J.-N. and Schubnel, A., 2016, Dynamic weakening and amorphization in serpentinite during laboratory earthquakes. Geology, 44, 607-610.
[https://doi.org/10.1130/G37932.1]
-
Brantut, N., Schubnel, A., Rouzaud, J.N., Brunet, F. and Shimamoto, T., 2008, High-velocity frictional properties of a clay-bearing fault gouge and implications for earthquake mechanics. Journal of Geophysical Research: Solid Earth, 113, B10401.
[https://doi.org/10.1029/2007JB005551]
-
Carpenter, B.M., Saffer, D.M. and Marone, C., 2012, Frictional properties and sliding stability of the San Andreas fault from deep drill core. Geology, 40, 759-762.
[https://doi.org/10.1130/G33007.1]
-
Cashman, S.M., Baldwin, J.N., Cashman, K.V., Swanson, K. and Crawford, R., 2007, Microstructures developed by coseismic and aseismic faulting in near-surface sediments, San Andreas fault, California. Geology, 35, 611-614.
[https://doi.org/10.1130/G23545A.1]
- Chang, C.J. and Chang, T.W., 2009, Behavioral characteristics of the Yangsan Fault based on geometric analysis of fault slip. The Journal of Engineering Geology, 19, 277-285 (in Korean with English abstract).
-
Cheon, Y., Cho, H., Ha, S., Kang, H.-C., Kim, J.-S. and Son, M., 2019, Tectonically controlled multiple stages of deformation along the Yangsan Fault Zone, SE Korea, since Late Cretaceous. Journal of Asian Earth Sciences, 170, 188-207.
[https://doi.org/10.1016/j.jseaes.2018.11.003]
-
Chester, F.M. and Chester, J.S., 1998, Ultracataclasite structure and friction processes of the Punchbowl fault, San Andreas system, California. Tectonophysics, 295, 199-221.
[https://doi.org/10.1016/S0040-1951(98)00121-8]
-
Chester, F.M. and Logan, J.M., 1987, Composite planar fabric of gouge from the Punchbowi Fault, California. Journal of Structural Geology, 9, 621-634.
[https://doi.org/10.1016/0191-8141(87)90147-7]
-
Choi, J.-H., Kim, Y.-S. and Klinger, Y., 2017, Recent progress in studies on the characteristics of surface rupture associated with large earthquakes. Journal of the Geological Society of Korea, 53, 129-157 (in Korean with English abstract).
[https://doi.org/10.14770/jgsk.2017.53.1.129]
-
Choi, J.-H., Klinger, Y., Ferry, M., Ritz, J.-F., Kurtz, R., Rizza, M., Bollinger, L., Davaasambuu, B., Tsend-Ayush, N. and Demberel, S., 2018, Geologic Inheritance and Earthquake Rupture Processes: The 1905 M ≥ 8 Tsetserleg-Bulnay Strike-Slip Earthquake Sequence, Mongolia. Journal of Geophysical Research: Solid Earth, 123, 1925-1953.
[https://doi.org/10.1002/2017JB013962]
- Choi, J.-H., Yang, S.-J. and Kim, Y.-S., 2009, Fault zone classification and structural characteristics of the southern Yangsan fault in the Sangcheon-ri area, SE Korea. Journal of the Geological Society of Korea, 45, 9-28. (in Korean with English abstract)
- Chwae, U., Ryoo, C.-R., Kee, W.-S., Lee, B.J., Lee, B.J., Hwang, J.H., Park, K.H., Choi, Y.S., Choi, S.-J., Choi, P., Cho, D.-L., Kim, B.-C., Song, K.-Y., Chae, B.-G., Kim, W.-Y., Kim, J.-Y., Lee, S.-K., Cho, S.-J., Hwang, S., Hwang, H.S., Kim, Y.-S., Hyun, H.-J., Park, I.-H., Lee, H.-I., Lee, D.Y., Lee, C.-B., Kim, J.-Y., Yang, D.Y., Park, D.-W., Shin, S.-C., Kim, Y.S., Kim, I.-J., Yoo, J.-H., Jin, M.-S., Jun, M.-S., Chi, H.-C., Jeon, J.-S., Shin, I.-C., Kang, I.-B., Shin, H.M., Kwon, M.C., Oh, S.J., Kim, S.G., Yim, M.S., Kim, S.G. and Jeong, H.Y., 1998, Final report of the re-evaluation to the design base earthquake considering the Yangsan fault(1). Report KR-B-255-1998, Korea Institute of Geoscience and Mineral Resources, Daejeon, 1694 p (in Korean).
-
Collettini, C., Chiaraluce, L., Pucci, S., Barchi, M.R. and Cocco, M., 2005, Looking at fault reactivation matching structural geology and seismological data. Journal of Structural Geology, 27, 937-942.
[https://doi.org/10.1016/j.jsg.2004.10.016]
-
Collettini, C. and Holdsworth, R.E., 2004, Fault zone weakening and character of slip along low-angle normal faults: insights from the Zuccale fault, Elba, Italy. Journal of the Geological Society, 161, 1039-1051.
[https://doi.org/10.1144/0016-764903-179]
-
Collettini, C., Niemeijer, A., Viti, C. and Marone, C., 2009, Fault zone fabric and fault weakness. Nature, 462, 907-910.
[https://doi.org/10.1038/nature08585]
-
Collettini, C., Viti, C., Tesei, T. and Mollo, S., 2013, Thermal decomposition along natural carbonate faults during earthquakes. Geology, 41, 927-930.
[https://doi.org/10.1130/G34421.1]
-
Cowan, D.S., 1999, Do faults preserve a record of seismic slip? A field geologist's opinion. Journal of Structural Geology, 21, 995-1001.
[https://doi.org/10.1016/S0191-8141(99)00046-2]
-
De Paola, N., Collettini, C., Faulkner, D.R. and Trippetta, F., 2008, Fault zone architecture and deformation processes within evaporitic rocks in the upper crust. Tectonics, 27, TC4017.
[https://doi.org/10.1029/2007TC002230]
-
Di Toro, G., Han, R., Hirose, T., De Paola, N., Nielsen, S., Mizoguchi, K., Ferri, F., Cocco, M. and Shimamoto, T., 2011, Fault lubrication during earthquakes. Nature, 471, 494-498.
[https://doi.org/10.1038/nature09838]
-
Di Toro, G., Hirose, T., Nielsen, S., Pennacchioni, G. and Shimamoto, T., 2006, Natural and Experimental Evidence of Melt Lubrication of Faults During Earthquakes. Science, 311, 647-649.
[https://doi.org/10.1126/science.1121012]
-
Faulkner, D.R., Lewis, A.C. and Rutter, E.H., 2003, On the internal structure and mechanics of large strike-slip fault zones: field observations of the Carboneras fault in southeastern Spain. Tectonophysics, 367, 235-251.
[https://doi.org/10.1016/S0040-1951(03)00134-3]
-
Fukuchi, T., 1988, Applicability of ESR dating using multiple centres to fault movement: The case of the Itoigawa-Shizuoka tectonic line, a major fault in Japan. Quaternary Science Reviews, 7, 509-514.
[https://doi.org/10.1016/0277-3791(88)90054-6]
-
Han, R., Hirose, T. and Shimamoto, T., 2010, Strong velocity weakening and powder lubrication of simulated carbonate faults at seismic slip rates. Journal of Geophysical Research: Solid Earth, 115, B03412.
[https://doi.org/10.1029/2008JB006136]
-
Han, R., Hirose, T., Shimamoto, T., Lee, Y. and Ando, J.-i., 2011, Granular nanoparticles lubricate faults during seismic slip. Geology, 39, 599-602.
[https://doi.org/10.1130/G31842.1]
-
Han, R., Shimamoto, T., Ando, J.-I. and Ree, J.-H., 2007b, Seismic slip record in carbonate-bearing fault zones: An insight from high-velocity friction experiments on siderite gouge. Geology, 35, 1131-1134.
[https://doi.org/10.1130/G24106A.1]
-
Han, R., Shimamoto, T., Hirose, T., Ree, J.-H. and Ando, J.-I., 2007a, Ultralow Friction of Carbonate Faults Caused by Thermal Decomposition. Science, 316, 878-881.
[https://doi.org/10.1126/science.1139763]
-
Hayward, K.S., Cox, S.F., Gerald, J.D.F., Slagmolen, B.J., Shaddock, D.A., Forsyth, P.W.F., Salmon, M.L. and Hawkins, R.P., 2016, Mechanical amorphization, flash heating, and frictional melting: Dramatic changes to fault surfaces during the first millisecond of earthquake slip. Geology, 44, 1043-1046.
[https://doi.org/10.1130/G38242.1]
-
Hirono, T., Asayama, S., Kaneki, S. and Ito, A., 2016, Preservation of amorphous ultrafine material: A proposed proxy for slip during recent earthquakes on active faults. Scientific Reports, 6, 36536.
[https://doi.org/10.1038/srep36536]
-
Hirose, T. and Bystricky, M., 2007, Extreme dynamic weakening of faults during dehydration by coseismic shear heating. Geophysical Research Letters, 34, L14311.
[https://doi.org/10.1029/2007GL030049]
-
Holdsworth, R.E., 2004, Weak Faults--Rotten Cores. Science, 303, 181-182.
[https://doi.org/10.1126/science.1092491]
-
Hwang, B.-H., Ernst, W.G. and Yang, K., 2012, Two different magma series imply a Palaeogene tectonic transition from contraction to extension in the SE Korean Peninsula. International Geology Review, 54, 1284-1295.
[https://doi.org/10.1080/00206814.2011.636990]
-
Ikari, M.J., 2015, Principal slip zones: Precursors but not recorders of earthquake slip. Geology, 43, 955-958.
[https://doi.org/10.1130/G37028.1]
-
Ikari, M.J., Marone, C. and Saffer, D.M., 2011, On the relation between fault strength and frictional stability. Geology, 39, 83-86.
[https://doi.org/10.1130/G31416.1]
-
Janssen, C., Wirth, R., Rybacki, E., Naumann, R., Kemnitz, H., Wenk, H.R. and Dresen, G., 2010, Amorphous material in SAFOD core samples (San Andreas Fault): Evidence for crush-origin pseudotachylytes?. Geophysical Research Letters, 37, L01303.
[https://doi.org/10.1029/2009GL040993]
- Kang, H.-C., Cheon, Y., Ha, S., Seo, K., Kim, J.-S., Shin, H.C. and Son, M., 2018, Geology and U-Pb Age in the Eastern Part of Yeongdeok-gun, Gyeongsangbuk-do, Korea. The Journal of the Petrological Society of Korea, 27, 153-171 (in Korean with English abstract).
- Kee, W.-S., Kim, B.-C., Hwang, J.-H., Song, K.-Y. and Kihm, Y.-H., 2007, Structural Characteristics of Quaternary reverse faulting on the Eupcheon Fault, SE Korea. Journal of the Geological Society of Korea, 43, 311-333 (in Korean with English abstract).
- Kee, W.-S., Kim, Y.-H., Lee, H.-J., Choi, D.-L., Kim, B.-C., Song, K.-Y., Koh, H.-J., Lee, S.R., Gwang, Y.Y., Hwang, S.-H., Park, K.-G. and Sung, N.-H., 2009, South eastern fault variable research and DB Construction. KIGAM, Daejeon, 327 p.
-
Kim, C.-M., Han, R., Jeong, G.Y., Jeong, J.O. and Son, M., 2016, Internal structure and materials of the Yangsan fault, Bogyeongsa area, Pohang, South Korea. Geosciences Journal, 20, 759-773.
[https://doi.org/10.1007/s12303-016-0019-8]
-
Kim, J.H., Ree, J.-H., Choi, J.-H., Chauhan, N., Hirose, T. and Kitamura, M., 2019, Experimental investigations on dating the last earthquake event using OSL signals of quartz from fault gouges. Tectonophysics, 769, 228191.
[https://doi.org/10.1016/j.tecto.2019.228191]
-
Kim, M.-C., Jung, S., Yoon, S., Jeong, R.-Y., Song, C.W. and Son, M., 2016, Neotectonic Crustal Deformation and Current Stress Field in the Korean Peninsula and Their Tectonic Implications: A Review. Journal of the Petrological Society of Korea, 25, 169-193 (in Korean with English abstract).
[https://doi.org/10.7854/JPSK.2016.25.3.169]
- Kim, Y.-S. and Jin, K.M., 2006, Estimated earthquake magnitude from the Yugye Fault displacement on a trench section in Pohang, SE Korea. Journal of the Geological Society of Korea, 42, 79-94 (in Korean with English abstract).
- Kim, Y.-S., Jin, K., Choi, W.-H. and Kee, W.-S., 2011, Understanding of active faults: A review for recent researches. Journal of the Geological Society of Korea, 47, 723-752 (in Korean with English abstract).
-
Klinger, Y., Xu, X., Tapponnier, P., Van der Woerd, J.r., Lasserre, C. and King, G., 2005, High-Resolution Satellite Imagery Mapping of the Surface Rupture and Slip Distribution of the Mw ~7.8, 14 November 2001 Kokoxili Earthquake, Kunlun Fault, Northern Tibet, China. Bulletin of the Seismological Society of America, 95, 1970-1987.
[https://doi.org/10.1785/0120040233]
- Kyung, J.B., 2003, Paleoseismology of the Yangsan fault, southeastern part of the Korean peninsula. Annals of Geophysics, 46, 983-996.
-
Lee, H.-K. and Schwarcz, H.P., 2001, ESR dating of the subsidiary faults in the Yangsan fault system, Korea. Quaternary Science Reviews, 20, 999-1003.
[https://doi.org/10.1016/S0277-3791(00)00055-X]
-
Lee, J., Rezaei, S., Hong, Y., Choi, J.-H., Choi, J.-H., Choi, W.-H., Rhee, K.-W. and Kim, Y.-S., 2015, Quaternary fault analysis through a trench investigation on the northern extension of the Yangsan fault at Dangu-ri, Gyungju-si, Gyeongsangbuk-do. Journal of the Geological Society of Korea, 51, 471-485 (in Korean with English abstract).
[https://doi.org/10.14770/jgsk.2015.51.5.471]
- Lee, K. and Jin, Y.G., 1991, Segmentation of the Yangsan Fault System: Geophysical Studies on Major Faults in the Kyeongsang Basin. Journal of Geological Society of Korea, 27, 434-449.
-
Lin, A., 1994, Glassy pseudotachylyte veins from the Fuyun fault zone, northwest China. Journal of Structural Geology, 16, 71-83.
[https://doi.org/10.1016/0191-8141(94)90019-1]
-
Lin, A., 2001, S-C fabrics developed in cataclas tic rocks from the Nojima fault zone, Japan and their implications for tectonic history. Journal of Structural Geology, 23, 1167-1178.
[https://doi.org/10.1016/S0191-8141(00)00171-1]
-
McCalpin, J.P., 1996, Chapter 2 Field techniques in paleoseismology. (Ed.) International Geophysics, Academic Press, 62, 33-83.
[https://doi.org/10.1016/S0074-6142(96)80069-6]
-
Meyer, B., Lacassin, R., Brulhet, J. and Mouroux, B., 1994, The Basel 1356 earthquake: which fault produced it?. Terra Nova, 6, 54-63.
[https://doi.org/10.1111/j.1365-3121.1994.tb00633.x]
-
Michetti, A.M., Audemard M, F.A. and Marco, S., 2005, Future trends in paleoseismology: Integrated study of the seismic landscape as a vital tool in seismic hazard analyses. Tectonophysics, 408, 3-21.
[https://doi.org/10.1016/j.tecto.2005.05.035]
-
Ota, Y., Azuma, T. and Lin, Y.N., 2009, Application of INQUA Environmental Seismic Intensity Scale to recent earthquakes in Japan and Taiwan. Special Publication of the Geological Society of London, 316, 55-71.
[https://doi.org/10.1144/SP316.4]
-
Rajendran, C.P., Rajendran, K. and John, B., 1996, The 1993 Killari (Latur), central India, earthquake: An example of fault reactivation in the Precambrian crust. Geology, 24, 651-654.
[https://doi.org/10.1130/0091-7613(1996)024<0651:TKLCIE>2.3.CO;2]
-
Rawling, G.C. and Goodwin, L.B., 2003, Cataclasis and particulate flow in faulted, poorly lithified sediments. Journal of Structural Geology, 25, 317-331.
[https://doi.org/10.1016/S0191-8141(02)00041-X]
-
Ree, J.-H., Lee, Y.-J., Rhodes, E.J., Park, Y., Kwon, S.-T., Chwae, U., Jeon, J.-S. and Lee, B., 2003, Quaternary reactivation of Tertiary faults in the southeastern Korean Peninsula: Age constraint by optically stimulated luminescence dating. The Island Arc, 12, 1-12.
[https://doi.org/10.1046/j.1440-1738.2003.00372.x]
-
Rice, J.R., 2006, Heating and weakening of faults during earthquake slip. Journal of Geophysical Research, 111, B05311.
[https://doi.org/10.1029/2005JB004006]
-
Rockwell, T., Barka, A., Dawson, T., Akyuz, S. and Thorup, K., 2001, Paleoseismology of the Gazikoy-Saros segment of the North Anatolia fault, northwestern Turkey: Comparison of the historical and paleoseismic records, implications of regional seismic hazard, and models of earthquake recurrence. Journal of Seismology, 5, 433-448.
[https://doi.org/10.1023/A:1011435927983]
-
Rowe, C.D. and Griffith, W.A., 2015, Do faults preserve a record of seismic slip: A second opinion. Journal of Structural Geology, 78, 1-26.
[https://doi.org/10.1016/j.jsg.2015.06.006]
-
Rowe, C.D., Lamothe, K., Rempe, M., Andrews, M., Mitchell, T.M., Di Toro, G., White, J.C. and Aretusini, S., 2019, Earthquake lubrication and healing explained by amorphous nanosilica. Nature Communications, 10, 1-11.
[https://doi.org/10.1038/s41467-018-08238-y]
- Shibata, K., Uchiumi, S. and Nakagawa, T., 1979, K-Ar age result-1. Bulletin of Geological Survey Japan, 30, 675-686.
-
Shipton, Z.K., Meghraoui, M. and Monro, L., 2017, Seismic slip on the west flank of the Upper Rhine Graben (France-Germany): evidence from tectonic morphology and cataclastic deformation bands. Geological Society, London, Special Publications, 432, 147-161.
[https://doi.org/10.1144/SP432.12]
-
Sibson, R.H., 1977, Fault rocks and fault mechanisms. Journal of the Geological Society of London, 133, 191-213.
[https://doi.org/10.1144/gsjgs.133.3.0191]
-
Sibson, R.H., 1989, Earthquake faulting as a structural process. Journal of Structural Geology, 11, 1-14.
[https://doi.org/10.1016/0191-8141(89)90032-1]
- Sibson, R.H., 1990, Rupture nucleation on unfavorably oriented faults. Bulletin of the Seismological Society of America, 80, 1580-1604.
-
Sibson, R.H., 2003, Thickness of the Seismic Slip Zone. Bulletin of the Seismological Society of America, 93, 1169-1178.
[https://doi.org/10.1785/0120020061]
-
Siman-Tov, S., Aharonov, E., Sagy, A. and Emmanuel, S., 2013, Nanograins form carbonate fault mirrors. Geology, 41, 703-706.
[https://doi.org/10.1130/G34087.1]
-
Smeraglia, L., Billi, A., Carminati, E., Cavallo, A. and Doglioni, C., 2017, Field- to nano-scale evidence for weakening mechanisms along the fault of the 2016 Amatrice and Norcia earthquakes, Italy. Tectonophysics, 712-713, 156-169.
[https://doi.org/10.1016/j.tecto.2017.05.014]
-
Smith, S.A.F., Billi, A., Toro, G.D. and Spiess, R., 2011, Principal Slip Zones in Limestone: Microstructural Characterization and Implications for the Seismic Cycle (Tre Monti Fault, Central Apennines, Italy). Pure and Applied Geophysics, 168, 2365-2393.
[https://doi.org/10.1007/s00024-011-0267-5]
-
Smith, S.A.F., Di Toro, G., Kim, S., Ree, J.-H., Nielsen, S., Billi, A. and Spiess, R., 2013, Coseismic recrystallization during shallow earthquake slip. Geology, 41, 63-66.
[https://doi.org/10.1130/G33588.1]
-
Smith, S.A.F., Griffiths, J.R., Fondriest, M. and Di Toro, G., 2017a, “Coseismic Foliations” in Gouge and Cataclasite: Experimental Observations and Consequences for Interpreting the Fault Rock Record. Fault Zone Dynamic Processes: Evolution of‐Fault Properties During Seismic Rupture, Geophysical Monograph, 277 p.
[https://doi.org/10.1002/9781119156895.ch5]
-
Smith, S.A.F., Tesei, T., Scott, J.M. and Collettini, C., 2017b, Reactivation of normal faults as high-angle reverse faults due to low frictional strength: Experimental data from the Moonlight Fault Zone, New Zealand. Journal of Structural Geology, 105, 34-43.
[https://doi.org/10.1016/j.jsg.2017.10.009]
-
Tagami, T. and Murakami, M., 2007, Probing fault zone heterogeneity on the Nojima fault: Constraints from zircon fission-track analysis of borehole samples. Tectonophysics, 443, 139-152.
[https://doi.org/10.1016/j.tecto.2007.01.013]
-
Tanikawa, W. and Shimamoto, T., 2009, Frictional and transport properties of the Chelungpu fault from shallow borehole data and their correlation with seismic behavior during the 1999 Chi-Chi earthquake. Journal of Geophysical Research: Solid Earth, 114, B01502.
[https://doi.org/10.1029/2008JB005750]
-
Tarling, M.S., Smith, S.A.F., Viti, C. and Scott, J.M., 2018, Dynamic earthquake rupture preserved in a creeping serpentinite shear zone. Nature Communications, 9, 1-8.
[https://doi.org/10.1038/s41467-018-05965-0]
-
Tembe, S., Lockner, D.A. and Wong, T.-F., 2010, Effect of clay content and mineralogy on frictional sliding behavior of simulated gouges: Binary and ternary mixtures of quartz, illite, and montmorillonite. Journal of Geophysical Research: Solid Earth, 115, B03416.
[https://doi.org/10.1029/2009JB006383]
-
Valoroso, L., Chiaraluce, L. and Collettini, C., 2014, Earthquakes and fault zone structure. Geology, 42, 343-346.
[https://doi.org/10.1130/G35071.1]
-
Viti, C., 2011, Exploring fault rocks at the nanoscale. Journal of Structural Geology, 33, 1715-1727.
[https://doi.org/10.1016/j.jsg.2011.10.005]
-
Woo, S. and Han, R., 2019, Shear tests on intact fault gouges preserving natural deformation fabrics. Journal of the Geological Society of Korea, 55, 131-139 (in Korean with English abstract).
[https://doi.org/10.14770/jgsk.2019.55.1.131]
-
Woo, S., Han, R., Kim, C.-M., Jeong, G.Y., Jeong, J.O. and Lee, H., 2016, Relation between temporal change of fault rock materials and mechanical properties. Journal of the Geological Society of Korea, 52, 847-861 (in Korean with English abstract).
[https://doi.org/10.14770/jgsk.2016.52.6.847]
-
Yang, H.L., Chen, J., Yao, L., Liu, C.R., Shimamoto, T. and Thompson Jobe, J.A., 2019, Resetting of OSL/TL/ESR signals by frictional heating in experimentally sheared quartz gouge at seismic slip rates. Quaternary Geochronology, 49, 52-56.
[https://doi.org/10.1016/j.quageo.2018.05.005]
-
Yao, L., Ma, S., Shimamoto, T. and Togo, T., 2013, Structures and high-velocity frictional properties of the Pingxi fault zone in the Longmenshan fault system, Sichuan, China, activated during the 2008 Wenchuan earthquake. Tectonophysics, 599, 135-156.
[https://doi.org/10.1016/j.tecto.2013.04.011]
-
Zwingmann, H., Offler, R., Wilson, T. and Cox, S.F., 2004, K-Ar dating of fault gouge in the northern Sydney Basin, NSW, Australia-implications for the breakup of Gondwana. Journal of Structural Geology, 26, 2285-2295.
[https://doi.org/10.1016/j.jsg.2004.03.007]